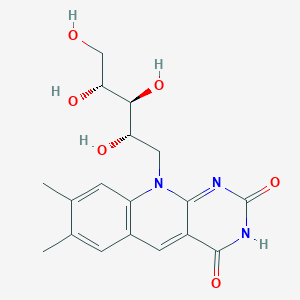
5-Deazariboflavin
Übersicht
Beschreibung
5-Deazariboflavin: is a synthetic analog of naturally occurring flavins, such as riboflavin, flavin mononucleotide, and flavin adenine dinucleotide. It is characterized by the replacement of the nitrogen atom at the N5 position of the isoalloxazine ring with a carbon atom, resulting in a unique structure that significantly alters its electrochemical and photochemical properties . This compound is frequently used as a replacement coenzyme in various flavoproteins to study and manipulate their reaction mechanisms .
Wirkmechanismus
Target of Action
5-Deazariboflavin, an important analogue of naturally occurring flavins such as riboflavin, flavin mononucleotide (FMN), and flavin adenine dinucleotide (FAD), primarily targets flavoproteins . These flavoproteins play a crucial role in various biochemical processes. The use of this compound as a replacement coenzyme in flavoproteins has proven particularly valuable in unraveling and manipulating their reaction mechanisms .
Mode of Action
This compound interacts with its targets, the flavoproteins, by serving as a substitute for natural flavin cofactors. This interaction allows for the investigation and modification of the reaction pathways of flavoproteins . It was frequently reported that one-electron-transfer reactions in flavoproteins are impeded with this compound as a cofactor . Based on these findings, it was concluded that the this compound radical is significantly less stable compared to the respective flavin semiquinone, and quickly re-oxidizes or undergoes disproportionation .
Biochemical Pathways
This compound affects the biochemical pathways of flavoproteins. It serves as a substitute for natural flavin cofactors, allowing for the fine-tuning of the reaction kinetics and absorption characteristics of flavoproteins . The use of this compound as a replacement coenzyme in flavoproteins has proven particularly valuable in unraveling and manipulating their reaction mechanisms .
Pharmacokinetics
Its role as a replacement coenzyme in flavoproteins suggests that it may have unique pharmacokinetic properties that allow it to effectively interact with these proteins .
Result of Action
The result of this compound’s action is the modification of the reaction mechanisms of flavoproteins. By serving as a replacement coenzyme, it allows for the investigation and manipulation of these mechanisms . It was frequently reported that one-electron-transfer reactions in flavoproteins are impeded with this compound as a cofactor .
Action Environment
The action of this compound can be influenced by environmental factors. For example, the stability of the this compound radical, which is less stable compared to the respective flavin semiquinone, may be affected by the presence of oxygen
Biochemische Analyse
Biochemical Properties
5-Deazariboflavin plays a crucial role in biochemical reactions as a cofactor in flavoproteins. It interacts with various enzymes, proteins, and other biomolecules, influencing their reaction mechanisms. For instance, this compound has been used as a replacement coenzyme in flavoproteins to study their reaction pathways. It has been observed that one-electron-transfer reactions in flavoproteins are impeded when this compound is used as a cofactor . This is due to the instability of the this compound radical, which quickly re-oxidizes or undergoes disproportionation . Additionally, this compound derivatives have been identified as inhibitors of tyrosyl-DNA phosphodiesterase 2, a DNA-repair enzyme .
Cellular Effects
This compound influences various cellular processes, including cell signaling pathways, gene expression, and cellular metabolism. It has been shown to affect the function of photolyases, which are flavoenzymes responsible for light-driven repair of DNA damage caused by UV exposure . In particular, this compound derivatives can serve as light-harvesting chromophores in photolyases, facilitating efficient energy transfer for DNA repair . The presence of this compound in cells can also impact the stability and activity of flavoproteins, thereby influencing cellular metabolism and gene expression .
Molecular Mechanism
The molecular mechanism of this compound involves its interaction with various biomolecules, including enzymes and proteins. It acts as a cofactor in flavoproteins, participating in redox reactions. The unique structure of this compound allows it to function as a two-electron/hydride acceptor/donor, similar to nicotinamide . Its radical form is less stable compared to flavin semiquinone, leading to rapid re-oxidation or disproportionation . This property makes this compound a valuable tool for studying the reaction mechanisms of flavoproteins and other redox-active enzymes.
Temporal Effects in Laboratory Settings
In laboratory settings, the effects of this compound can change over time due to its stability and degradation. Studies have shown that the fully reduced states of this compound are stable towards oxygen, making it a potentially useful photochemical tool . The radical form of this compound is less stable and can quickly re-oxidize or undergo disproportionation . Long-term effects on cellular function have been observed in in vitro and in vivo studies, highlighting the importance of considering the temporal stability of this compound in experimental designs.
Dosage Effects in Animal Models
The effects of this compound vary with different dosages in animal models. Studies have shown that at lower dosages, this compound can effectively participate in biochemical reactions without causing adverse effects . At higher dosages, toxic or adverse effects may be observed, including potential disruption of cellular metabolism and gene expression . It is important to determine the optimal dosage of this compound to achieve the desired biochemical effects while minimizing toxicity.
Metabolic Pathways
This compound is involved in various metabolic pathways, particularly those related to redox reactions. It interacts with enzymes and cofactors, facilitating the transfer of electrons and hydrides in biochemical processes . For example, this compound serves as a cofactor in methanogenic archaea, participating in substrate oxidation, C1 pathways, respiration, and oxygen detoxification . Its unique electrochemical properties make it a versatile cofactor in metabolic pathways, enhancing the metabolic flexibility of microorganisms.
Transport and Distribution
Within cells and tissues, this compound is transported and distributed through interactions with transporters and binding proteins. These interactions influence its localization and accumulation in specific cellular compartments . For instance, this compound derivatives can be transported to the catalytic center of photolyases, where they function as light-harvesting chromophores . Understanding the transport and distribution mechanisms of this compound is crucial for optimizing its use in biochemical research.
Subcellular Localization
The subcellular localization of this compound affects its activity and function. It can be directed to specific compartments or organelles through targeting signals or post-translational modifications . For example, in photolyases, this compound derivatives are localized to the catalytic center, where they facilitate DNA repair . The localization of this compound within cells can influence its interactions with other biomolecules and its overall biochemical effects.
Vorbereitungsmethoden
Synthetic Routes and Reaction Conditions: The synthesis of 5-Deazariboflavin typically involves the following steps:
Formation of the Isoalloxazine Ring: The synthesis begins with the formation of the isoalloxazine ring system. This can be achieved through the condensation of appropriate aromatic amines with glyoxal and urea under acidic conditions.
Introduction of the Deaza Modification: The key step involves the replacement of the nitrogen atom at the N5 position with a carbon atom.
Industrial Production Methods: While specific industrial production methods for this compound are not widely documented, the general approach would involve scaling up the laboratory synthesis procedures. This includes optimizing reaction conditions, using high-purity reagents, and employing efficient purification techniques such as chromatography to obtain the desired product in large quantities .
Analyse Chemischer Reaktionen
Types of Reactions: 5-Deazariboflavin undergoes several types of chemical reactions, including:
Oxidation: It can participate in oxidation reactions, where it acts as an electron acceptor.
Reduction: It can also undergo reduction reactions, serving as an electron donor.
Common Reagents and Conditions:
Oxidation Reactions: Common oxidizing agents include hydrogen peroxide and molecular oxygen. These reactions are typically carried out under mild conditions to prevent degradation of the compound.
Reduction Reactions: Reducing agents such as sodium borohydride and lithium aluminum hydride are commonly used.
Major Products: The major products formed from these reactions depend on the specific conditions and reagents used. For example, oxidation reactions may yield various oxidized forms of this compound, while reduction reactions can produce reduced forms with different degrees of hydrogenation .
Wissenschaftliche Forschungsanwendungen
Vergleich Mit ähnlichen Verbindungen
Riboflavin: A naturally occurring flavin that serves as a coenzyme in various biological reactions.
Flavin Mononucleotide: A derivative of riboflavin that acts as a coenzyme in redox reactions.
Flavin Adenine Dinucleotide: Another derivative of riboflavin that plays a crucial role in cellular respiration and energy production.
Uniqueness of 5-Deazariboflavin: The primary distinction of this compound lies in the replacement of the nitrogen atom at the N5 position with a carbon atom. This modification significantly alters its electrochemical and photochemical properties, making it a valuable tool for studying the mechanisms of flavoprotein-catalyzed reactions. Unlike natural flavins, this compound is less stable in its radical form, which provides unique insights into the electron transfer processes in flavoproteins .
Eigenschaften
IUPAC Name |
7,8-dimethyl-10-[(2S,3S,4R)-2,3,4,5-tetrahydroxypentyl]pyrimido[4,5-b]quinoline-2,4-dione | |
---|---|---|
Source | PubChem | |
URL | https://pubchem.ncbi.nlm.nih.gov | |
Description | Data deposited in or computed by PubChem | |
InChI |
InChI=1S/C18H21N3O6/c1-8-3-10-5-11-16(19-18(27)20-17(11)26)21(12(10)4-9(8)2)6-13(23)15(25)14(24)7-22/h3-5,13-15,22-25H,6-7H2,1-2H3,(H,20,26,27)/t13-,14+,15-/m0/s1 | |
Source | PubChem | |
URL | https://pubchem.ncbi.nlm.nih.gov | |
Description | Data deposited in or computed by PubChem | |
InChI Key |
XHELUUHUHSGXOC-ZNMIVQPWSA-N | |
Source | PubChem | |
URL | https://pubchem.ncbi.nlm.nih.gov | |
Description | Data deposited in or computed by PubChem | |
Canonical SMILES |
CC1=CC2=C(C=C1C)N(C3=NC(=O)NC(=O)C3=C2)CC(C(C(CO)O)O)O | |
Source | PubChem | |
URL | https://pubchem.ncbi.nlm.nih.gov | |
Description | Data deposited in or computed by PubChem | |
Isomeric SMILES |
CC1=CC2=C(C=C1C)N(C3=NC(=O)NC(=O)C3=C2)C[C@@H]([C@@H]([C@@H](CO)O)O)O | |
Source | PubChem | |
URL | https://pubchem.ncbi.nlm.nih.gov | |
Description | Data deposited in or computed by PubChem | |
Molecular Formula |
C18H21N3O6 | |
Source | PubChem | |
URL | https://pubchem.ncbi.nlm.nih.gov | |
Description | Data deposited in or computed by PubChem | |
DSSTOX Substance ID |
DTXSID00941025 | |
Record name | 1-Deoxy-1-(4-hydroxy-7,8-dimethyl-2-oxopyrimido[4,5-b]quinolin-10(2H)-yl)pentitol | |
Source | EPA DSSTox | |
URL | https://comptox.epa.gov/dashboard/DTXSID00941025 | |
Description | DSSTox provides a high quality public chemistry resource for supporting improved predictive toxicology. | |
Molecular Weight |
375.4 g/mol | |
Source | PubChem | |
URL | https://pubchem.ncbi.nlm.nih.gov | |
Description | Data deposited in or computed by PubChem | |
CAS No. |
19342-73-5 | |
Record name | 5-Deazariboflavin | |
Source | ChemIDplus | |
URL | https://pubchem.ncbi.nlm.nih.gov/substance/?source=chemidplus&sourceid=0019342735 | |
Description | ChemIDplus is a free, web search system that provides access to the structure and nomenclature authority files used for the identification of chemical substances cited in National Library of Medicine (NLM) databases, including the TOXNET system. | |
Record name | 1-Deoxy-1-(4-hydroxy-7,8-dimethyl-2-oxopyrimido[4,5-b]quinolin-10(2H)-yl)pentitol | |
Source | EPA DSSTox | |
URL | https://comptox.epa.gov/dashboard/DTXSID00941025 | |
Description | DSSTox provides a high quality public chemistry resource for supporting improved predictive toxicology. | |
Retrosynthesis Analysis
AI-Powered Synthesis Planning: Our tool employs the Template_relevance Pistachio, Template_relevance Bkms_metabolic, Template_relevance Pistachio_ringbreaker, Template_relevance Reaxys, Template_relevance Reaxys_biocatalysis model, leveraging a vast database of chemical reactions to predict feasible synthetic routes.
One-Step Synthesis Focus: Specifically designed for one-step synthesis, it provides concise and direct routes for your target compounds, streamlining the synthesis process.
Accurate Predictions: Utilizing the extensive PISTACHIO, BKMS_METABOLIC, PISTACHIO_RINGBREAKER, REAXYS, REAXYS_BIOCATALYSIS database, our tool offers high-accuracy predictions, reflecting the latest in chemical research and data.
Strategy Settings
Precursor scoring | Relevance Heuristic |
---|---|
Min. plausibility | 0.01 |
Model | Template_relevance |
Template Set | Pistachio/Bkms_metabolic/Pistachio_ringbreaker/Reaxys/Reaxys_biocatalysis |
Top-N result to add to graph | 6 |
Feasible Synthetic Routes
Haftungsausschluss und Informationen zu In-Vitro-Forschungsprodukten
Bitte beachten Sie, dass alle Artikel und Produktinformationen, die auf BenchChem präsentiert werden, ausschließlich zu Informationszwecken bestimmt sind. Die auf BenchChem zum Kauf angebotenen Produkte sind speziell für In-vitro-Studien konzipiert, die außerhalb lebender Organismen durchgeführt werden. In-vitro-Studien, abgeleitet von dem lateinischen Begriff "in Glas", beinhalten Experimente, die in kontrollierten Laborumgebungen unter Verwendung von Zellen oder Geweben durchgeführt werden. Es ist wichtig zu beachten, dass diese Produkte nicht als Arzneimittel oder Medikamente eingestuft sind und keine Zulassung der FDA für die Vorbeugung, Behandlung oder Heilung von medizinischen Zuständen, Beschwerden oder Krankheiten erhalten haben. Wir müssen betonen, dass jede Form der körperlichen Einführung dieser Produkte in Menschen oder Tiere gesetzlich strikt untersagt ist. Es ist unerlässlich, sich an diese Richtlinien zu halten, um die Einhaltung rechtlicher und ethischer Standards in Forschung und Experiment zu gewährleisten.