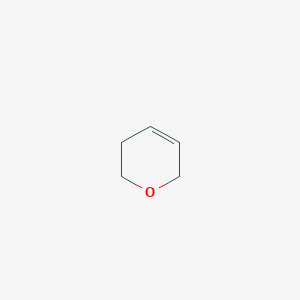
3,6-Dihydro-2H-pyran
Übersicht
Beschreibung
3,6-Dihydro-2H-pyran (DHP) is a six-membered heterocyclic compound containing one oxygen atom and two double bonds (at positions 3,4 and 5,6) (Figure 1). It is a colorless liquid with a molecular formula of C₅H₈O and a molecular weight of 84.11 g/mol . DHP is widely used in organic synthesis as a protecting group for alcohols and amines, as well as a precursor for pharmaceuticals and agrochemicals. Its synthesis typically involves cyclization reactions, such as the Prins cyclization, as demonstrated in the preparation of cis-2,6-disubstituted DHP derivatives (e.g., (2R,6S)-6-benzyl-2-methyl-DHP, synthesized in 48% yield via silyl-Prins cyclization) .
DHP’s pharmacological relevance is highlighted by its role as a morpholine replacement in ATP-competitive inhibitors of the mammalian target of rapamycin (mTOR). Substituting morpholine with DHP in pyrazolopyrimidine and thienopyrimidine derivatives retained equivalent potency and selectivity against PI3K, establishing DHP as a viable hinge-region binding motif .
Vorbereitungsmethoden
Grignard Addition and Boron Trifluoride-Catalyzed Cyclization
The reaction of tetrahydro-4H-pyran-4-one with arylmagnesium bromides represents a robust method for synthesizing 4-aryl-3,6-dihydro-2H-pyrans. As detailed in supplementary protocols , tetrahydro-4H-pyran-4-one (10 mmol) is treated with arylmagnesium bromide (12 mmol) in tetrahydrofuran (THF) at −78°C, followed by warming to room temperature. Subsequent addition of boron trifluoride diethyl etherate (BF₃·Et₂O) induces cyclization, yielding 4-aryl-3,6-dihydro-2H-pyrans after aqueous workup and silica gel chromatography.
Key Mechanistic Insights:
-
Nucleophilic Addition : The Grignard reagent attacks the carbonyl carbon of tetrahydro-4H-pyran-4-one, forming a magnesium alkoxide intermediate.
-
Cyclization : BF₃·Et₂O coordinates to the oxygen lone pairs, facilitating intramolecular etherification and ring closure .
This method tolerates electron-donating and electron-withdrawing aryl groups, though sterically hindered substrates may require extended reaction times. Yields are typically moderate to high (60–85%), with purity confirmed via ¹H NMR and high-resolution mass spectrometry (HRMS) .
Iron(III)-Catalyzed Cyclization of Aryl Ketones
Iron(III) chloride (FeCl₃) catalyzes the conversion of aryl ketones to 3,4-dihydro-2H-pyrans, which isomerize to 3,6-dihydro-2H-pyrans under specific conditions. For example, aryl ketone 11 undergoes FeCl₃-mediated enolization, forming a carbocation that cyclizes to pyran 36 (Fig. 1A) . Monitoring via ¹H NMR revealed transient pyran intermediates, which rearrange to tetrahydronaphthalenes over time. However, quenching the reaction at early stages isolates 3,6-dihydro-2H-pyrans in yields up to 83% .
Reaction Optimization:
-
Catalyst Loading : 10 mol% FeCl₃ in dichloromethane at 0°C.
-
Substrate Scope : Electron-rich aryl ketones exhibit faster cyclization rates than electron-deficient analogs.
Acid-Catalyzed Cyclization of Allyl Ethers
A two-step synthesis from 1-(allyloxy)pent-4-en-2-ol provides access to 3,6-dihydro-2H-pyran-3-ol. Cyclization under acidic conditions (e.g., HCl or H₂SO₄) generates the dihydropyran core, which is further functionalized via halogenation or phosphorylation . For instance, treatment of this compound-3-ol with HCl yields 3-chloro-3,6-dihydro-2H-pyran (2a ) in 75% yield after column chromatography .
Experimental Highlights:
-
Cyclization Step : 1-(Allyloxy)pent-4-en-2-ol (5 mmol) in THF with BF₃·Et₂O (1.2 equiv) at 0°C for 2 hours.
-
Derivatization : Chlorination with HCl (gas) in diethyl ether at −20°C .
Comparative Analysis of Synthetic Routes
Mechanistic Considerations
Divergent pathways emerge depending on the acid catalyst:
-
Brønsted Acids : Promote pyran formation via protonation of carbonyl oxygen, favoring six-membered transition states.
-
Lewis Acids (e.g., FeCl₃): Stabilize carbocation intermediates, enabling Friedel-Crafts alkylation or rearomatization .
Isotopic labeling studies suggest that FeCl₃-mediated cyclization proceeds through a kinetically controlled carbocation, which equilibrates between pyran and tetrahydronaphthalene products .
Analyse Chemischer Reaktionen
Arzoxifen durchläuft verschiedene Arten von chemischen Reaktionen, darunter:
Oxidation: Arzoxifen kann oxidiert werden, um verschiedene oxidierte Derivate zu bilden.
Reduktion: Reduktionsreaktionen können die funktionellen Gruppen am Benzothiophenkern verändern.
Substitution: Substitutionsreaktionen werden verwendet, um verschiedene funktionelle Gruppen in den Benzothiophenring einzuführen.
Zu den gängigen Reagenzien und Bedingungen, die in diesen Reaktionen verwendet werden, gehören Oxidationsmittel wie Kaliumpermanganat, Reduktionsmittel wie Lithiumaluminiumhydrid und verschiedene Katalysatoren, um Substitutionsreaktionen zu erleichtern. Die Hauptprodukte, die aus diesen Reaktionen gebildet werden, hängen von den verwendeten Reagenzien und Bedingungen ab .
Wissenschaftliche Forschungsanwendungen
Chemical Synthesis
Protecting Group in Organic Chemistry
One of the primary applications of 3,6-dihydro-2H-pyran is its use as a protecting group for alcohols in organic synthesis. It forms tetrahydropyranyl (THP) ethers, which effectively shield hydroxyl groups during chemical reactions. This application is crucial in the synthesis of complex organic molecules where selective reactivity is required. The stability of the THP ether under various reaction conditions makes it a preferred choice among chemists.
Synthetic Pathways
The compound can be synthesized through several methods, including:
- Electrophilic Addition : Dihydropyran can be generated from the reaction of α,β-unsaturated carbonyl compounds with alcohols.
- Cyclization Reactions : It can also be formed via cyclization of 1,3-dienes and carbonyl compounds under acidic conditions.
Medicinal Chemistry
Therapeutic Potential
Research into the derivatives of this compound has revealed promising therapeutic potentials. Notable findings include:
- Antimicrobial Activity : Studies have shown that certain derivatives exhibit significant antibacterial properties against pathogens such as Staphylococcus aureus and Klebsiella pneumoniae. These compounds disrupt cellular integrity and function, indicating their potential for developing new antibiotics .
- Antimalarial and Antituberculosis Properties : Some derivatives have demonstrated activity against Plasmodium falciparum, suggesting modifications to the dihydropyran structure can enhance biological activity. Additionally, there are indications of potential antituberculosis activity.
Case Study: Antimicrobial Activity
A study investigating the efficacy of various this compound derivatives against bacterial strains found that specific modifications led to enhanced inhibition rates. The mechanism involved appears to be linked to the disruption of bacterial cell walls.
Industrial Applications
Fine Chemicals Production
In industrial settings, this compound serves as an intermediate for producing fine chemicals and pharmaceuticals. Its role in synthesizing complex organic compounds is essential for developing new materials and active pharmaceutical ingredients.
Research Findings
Recent research has focused on the thermal stability and decomposition kinetics of this compound derivatives. These studies reveal insights into their reactivity profiles and potential applications in material science.
Thermal Decomposition Studies
Research indicates that substituents on the dihydropyran ring influence thermal decomposition pathways significantly. The activation energies for these reactions vary based on structural modifications, which can inform synthetic strategies .
Wirkmechanismus
Arzoxifene exerts its effects by binding to estrogen receptors and modulating their activity. It acts as an antagonist in mammary and uterine tissues, blocking the effects of estrogen and reducing the risk of cancer development. In bone tissue, arzoxifene functions as an agonist, promoting bone density and reducing the risk of osteoporosis. The molecular targets and pathways involved include estrogen receptor alpha and estrogen receptor beta, which regulate the expression of various genes involved in cell growth, differentiation, and survival .
Vergleich Mit ähnlichen Verbindungen
Comparison with Structurally Similar Compounds
Thermal Stability and Decomposition
A computational study compared the thermal decomposition profiles of DHP and its methyl-substituted derivatives (Table 1) :
Compound | Decomposition Temperature Range (°C) | Activation Energy (Eₐ, kJ·mol⁻¹) |
---|---|---|
3,6-Dihydro-2H-pyran (DHP) | 329–374 | 208.1 |
cis-2,6-Dimethyl-DHP (DMDHP) | 300–351 | 196.3 |
4-Methyl-DHP (MDHP) | 311–361 | 209.5 |
2-Methyl-3,4-dihydro-2H-pyran | 364–393 | 191.5 |
Key Findings :
- Methyl substitution at the 2,6-positions (DMDHP) lowers both decomposition temperature and activation energy compared to DHP, indicating reduced thermal stability.
- 4-Methyl-DHP exhibits similar stability to DHP, while 2-methyl-3,4-dihydro-2H-pyran shows higher decomposition temperatures but lower Eₐ, suggesting distinct decomposition pathways influenced by substituent position .
Pharmacological Activity: DHP vs. Morpholine
DHP and morpholine are both used as hinge-binding motifs in kinase inhibitors. A study replacing morpholine with DHP in mTOR inhibitors demonstrated:
- Equivalent potency : DHP-containing inhibitors (e.g., compound 21 ) showed IC₅₀ values comparable to morpholine analogues (e.g., compound 25 ) against mTOR .
- Variable degradation efficiency : In Cyclin K degraders, DHP (21 ) exhibited complete degradation in the CR8 core but only partial degradation in the dinaciclib core (37 ), despite structural similarity between CR8 and dinaciclib. This suggests context-dependent degradation mechanisms unrelated to permeability (PAMPA data showed comparable permeabilities for DHP and morpholine) .
Natural Occurrence and Derivatives
DHP derivatives are found in natural products and bioactive molecules:
- Lobane diterpenoids: 5,6-Dihydro-2H-pyran and pyranone moieties are common in Lobophytum soft corals, though DHP itself is rare in nature .
- Minor volatile compounds: 2-Methyl-5,6-dihydro-2H-pyran was detected as a minor component (1–3%) in CF016 culture extracts, alongside β-elemene and selinene derivatives .
Data Tables
Table 2: Key Physicochemical Properties
Property | This compound | Morpholine |
---|---|---|
Molecular Formula | C₅H₈O | C₄H₉NO |
Boiling Point | 84–86°C | 128–129°C |
LogP (Hydrophobicity) | 0.92 | -0.86 |
Role in Kinase Inhibitors | Hinge-binding motif | Hinge-binding motif |
Biologische Aktivität
3,6-Dihydro-2H-pyran (DHP) is a cyclic ether that has garnered attention in various fields of chemistry and biology due to its diverse biological activities and potential applications in medicinal chemistry. This article explores the biological activity of DHP, highlighting its pharmacological properties, mechanisms of action, and relevant case studies.
Chemical Structure and Properties
This compound features a six-membered ring structure containing one oxygen atom. Its molecular formula is , and it exhibits unique chemical properties that facilitate various biological interactions. The compound's boiling point is approximately 92-93 °C, and it has a density of 1.101 g/mL at 25 °C, which influences its bioavailability and interaction with biological systems.
Pharmacological Activities
DHP has been studied for its potential as an ATP-competitive inhibitor of the mammalian target of rapamycin (mTOR), a key regulator in cell growth and metabolism. This property makes it a candidate for cancer therapy, particularly in targeting pathways involved in tumor growth .
Table 1: Summary of Biological Activities of this compound
The biological activity of DHP can be attributed to its interaction with various biomolecules:
- Enzyme Interaction : DHP can modulate the activity of enzymes such as alcohol dehydrogenases, influencing metabolic pathways.
- Cell Signaling : It affects cellular signaling pathways, potentially altering gene expression and cellular metabolism.
- Thermal Stability : Studies indicate that DHP's thermal decomposition properties may play a role in its biological efficacy, as different substituents can affect its reactivity .
Inhibition of mTOR Pathway
A study demonstrated the effectiveness of DHP derivatives in inhibiting the mTOR pathway. The research involved synthesizing various DHP-based compounds and evaluating their inhibitory effects on mTOR activity in vitro. The findings revealed that specific substitutions on the DHP ring significantly enhanced inhibitory potency, suggesting a structure-activity relationship (SAR) that could be exploited for drug development .
Neuroprotective Effects
Research has indicated that DHP exhibits neuroprotective effects, making it a candidate for Alzheimer's disease treatment. In vitro studies showed that DHP could protect neuronal cells from oxidative stress-induced apoptosis by modulating antioxidant enzyme activity . These findings highlight the potential for DHP derivatives to serve as therapeutic agents in neurodegenerative disorders.
Research Findings and Data Analysis
Recent studies have utilized computational methods to analyze the activation energies associated with the thermal decomposition of DHP derivatives. The results indicate that variations in substituent groups significantly influence the stability and reactivity of these compounds. For instance:
Table 2: Activation Energies (Ea) of DHP Derivatives
Compound | Ea (kJ/mol) | Computational Method |
---|---|---|
This compound | 208 | B3LYP |
4-Methyl-3,6-dihydro-2H-pyran | 210 | M062X |
2,6-Dimethyl-3,6-dihydro-2H-pyran | 196 | PBE0 |
These data illustrate how computational chemistry can aid in understanding the biological activity by predicting stability and reactivity patterns based on molecular structure .
Q & A
Basic Research Questions
Q. What are the standard synthetic routes for preparing 3,6-Dihydro-2H-pyran derivatives, and how can reaction conditions be optimized?
- Methodology : this compound derivatives are synthesized via Pd-catalyzed allylic alkylation cascades or multi-component reactions involving arylamines, acetylenedicarboxylates, aldehydes, and cyclic diketones . Optimization involves adjusting catalysts (e.g., Bi(OTf)₃ or TMSOTf), solvent polarity, and temperature to control regioselectivity. For example, halogen-substituted derivatives (e.g., 4-fluoro, 4-chloro) are synthesized by reacting (Z)-silyl ethers with substituted oxabicycles, followed by column chromatography purification .
Q. What safety protocols are critical when handling this compound in laboratory settings?
- Methodology : The compound is highly flammable (UN 2376) and requires storage in well-ventilated, cool areas away from oxidizers. Use static-discharge-proof equipment and wear protective gloves/eyewear. In case of skin contact, rinse immediately with water and consult a physician. Spills should be contained using inert absorbents to avoid environmental contamination .
Q. How can diastereomeric purity of this compound derivatives be assessed during synthesis?
- Methodology : Thin-layer chromatography (TLC) and high-performance liquid chromatography (HPLC) are used for preliminary purity checks. For diastereomer separation, silica gel column chromatography with gradient elution (e.g., hexane/ethyl acetate) is effective. Confirmatory analysis via H NMR can detect splitting of proton signals due to stereochemical differences .
Advanced Research Questions
Q. How can computational models predict NMR chemical shifts to assign stereochemistry in this compound systems?
- Methodology : Axial shielding effects, influenced by pseudo-axial substituents and ring conformation, are modeled using correction constants derived from experimental data. For example, DFT calculations match shifts for cis- and trans-diastereomers with >95% confidence (DP⁴ probability). Discrepancies at C4/C5 (<1 ppm) are critical for distinguishing stereochemistry when only one diastereomer is available .
Q. What strategies resolve contradictions in stereochemical assignments of this compound derivatives lacking crystallographic data?
- Methodology : Combine / HSQC NMR with computational shielding analysis. For instance, axial substituents in cis-diastereomers exhibit distinct deshielding at C3/C6 due to hyperconjugation effects, while trans-isomers show shielding at C2. This differential pattern aligns with calculated conformational biases .
Q. How do multi-component reactions (MCRs) enable diversification of this compound scaffolds for drug discovery?
- Methodology : MCRs involving arylamines, acetylenedicarboxylates, and cyclic diketones yield 3,4-dihydropyridin-2-ones or pyran derivatives. Selectivity is controlled by diketone ring size: five-membered rings favor pyran formation, while six-membered rings yield pyridinones. This modular approach accelerates library generation for bioactivity screening .
Q. What role does axial shielding play in the Ferrier reaction mechanism for functionalizing this compound?
- Methodology : In Pd-catalyzed allylic alkylation, axial shielding from substituents directs nucleophilic attack. For example, bulky groups at C3/C6 hinder equatorial attack, favoring axial addition. This steric guidance is quantified via NMR shifts and corroborated by transition-state DFT calculations .
Eigenschaften
IUPAC Name |
3,6-dihydro-2H-pyran | |
---|---|---|
Source | PubChem | |
URL | https://pubchem.ncbi.nlm.nih.gov | |
Description | Data deposited in or computed by PubChem | |
InChI |
InChI=1S/C5H8O/c1-2-4-6-5-3-1/h1-2H,3-5H2 | |
Source | PubChem | |
URL | https://pubchem.ncbi.nlm.nih.gov | |
Description | Data deposited in or computed by PubChem | |
InChI Key |
MUGSKSNNEORSJG-UHFFFAOYSA-N | |
Source | PubChem | |
URL | https://pubchem.ncbi.nlm.nih.gov | |
Description | Data deposited in or computed by PubChem | |
Canonical SMILES |
C1COCC=C1 | |
Source | PubChem | |
URL | https://pubchem.ncbi.nlm.nih.gov | |
Description | Data deposited in or computed by PubChem | |
Molecular Formula |
C5H8O | |
Source | PubChem | |
URL | https://pubchem.ncbi.nlm.nih.gov | |
Description | Data deposited in or computed by PubChem | |
DSSTOX Substance ID |
DTXSID10953679 | |
Record name | 3,6-Dihydro-2H-pyran | |
Source | EPA DSSTox | |
URL | https://comptox.epa.gov/dashboard/DTXSID10953679 | |
Description | DSSTox provides a high quality public chemistry resource for supporting improved predictive toxicology. | |
Molecular Weight |
84.12 g/mol | |
Source | PubChem | |
URL | https://pubchem.ncbi.nlm.nih.gov | |
Description | Data deposited in or computed by PubChem | |
CAS No. |
3174-74-1 | |
Record name | 3,6-Dihydro-2H-pyran | |
Source | CAS Common Chemistry | |
URL | https://commonchemistry.cas.org/detail?cas_rn=3174-74-1 | |
Description | CAS Common Chemistry is an open community resource for accessing chemical information. Nearly 500,000 chemical substances from CAS REGISTRY cover areas of community interest, including common and frequently regulated chemicals, and those relevant to high school and undergraduate chemistry classes. This chemical information, curated by our expert scientists, is provided in alignment with our mission as a division of the American Chemical Society. | |
Explanation | The data from CAS Common Chemistry is provided under a CC-BY-NC 4.0 license, unless otherwise stated. | |
Record name | 2H-Pyran, 3,6-dihydro- | |
Source | ChemIDplus | |
URL | https://pubchem.ncbi.nlm.nih.gov/substance/?source=chemidplus&sourceid=0003174741 | |
Description | ChemIDplus is a free, web search system that provides access to the structure and nomenclature authority files used for the identification of chemical substances cited in National Library of Medicine (NLM) databases, including the TOXNET system. | |
Record name | 3,6-Dihydro-2H-pyran | |
Source | EPA DSSTox | |
URL | https://comptox.epa.gov/dashboard/DTXSID10953679 | |
Description | DSSTox provides a high quality public chemistry resource for supporting improved predictive toxicology. | |
Record name | 3,6-dihydro-2H-pyran | |
Source | European Chemicals Agency (ECHA) | |
URL | https://echa.europa.eu/information-on-chemicals | |
Description | The European Chemicals Agency (ECHA) is an agency of the European Union which is the driving force among regulatory authorities in implementing the EU's groundbreaking chemicals legislation for the benefit of human health and the environment as well as for innovation and competitiveness. | |
Explanation | Use of the information, documents and data from the ECHA website is subject to the terms and conditions of this Legal Notice, and subject to other binding limitations provided for under applicable law, the information, documents and data made available on the ECHA website may be reproduced, distributed and/or used, totally or in part, for non-commercial purposes provided that ECHA is acknowledged as the source: "Source: European Chemicals Agency, http://echa.europa.eu/". Such acknowledgement must be included in each copy of the material. ECHA permits and encourages organisations and individuals to create links to the ECHA website under the following cumulative conditions: Links can only be made to webpages that provide a link to the Legal Notice page. | |
Retrosynthesis Analysis
AI-Powered Synthesis Planning: Our tool employs the Template_relevance Pistachio, Template_relevance Bkms_metabolic, Template_relevance Pistachio_ringbreaker, Template_relevance Reaxys, Template_relevance Reaxys_biocatalysis model, leveraging a vast database of chemical reactions to predict feasible synthetic routes.
One-Step Synthesis Focus: Specifically designed for one-step synthesis, it provides concise and direct routes for your target compounds, streamlining the synthesis process.
Accurate Predictions: Utilizing the extensive PISTACHIO, BKMS_METABOLIC, PISTACHIO_RINGBREAKER, REAXYS, REAXYS_BIOCATALYSIS database, our tool offers high-accuracy predictions, reflecting the latest in chemical research and data.
Strategy Settings
Precursor scoring | Relevance Heuristic |
---|---|
Min. plausibility | 0.01 |
Model | Template_relevance |
Template Set | Pistachio/Bkms_metabolic/Pistachio_ringbreaker/Reaxys/Reaxys_biocatalysis |
Top-N result to add to graph | 6 |
Feasible Synthetic Routes
Haftungsausschluss und Informationen zu In-Vitro-Forschungsprodukten
Bitte beachten Sie, dass alle Artikel und Produktinformationen, die auf BenchChem präsentiert werden, ausschließlich zu Informationszwecken bestimmt sind. Die auf BenchChem zum Kauf angebotenen Produkte sind speziell für In-vitro-Studien konzipiert, die außerhalb lebender Organismen durchgeführt werden. In-vitro-Studien, abgeleitet von dem lateinischen Begriff "in Glas", beinhalten Experimente, die in kontrollierten Laborumgebungen unter Verwendung von Zellen oder Geweben durchgeführt werden. Es ist wichtig zu beachten, dass diese Produkte nicht als Arzneimittel oder Medikamente eingestuft sind und keine Zulassung der FDA für die Vorbeugung, Behandlung oder Heilung von medizinischen Zuständen, Beschwerden oder Krankheiten erhalten haben. Wir müssen betonen, dass jede Form der körperlichen Einführung dieser Produkte in Menschen oder Tiere gesetzlich strikt untersagt ist. Es ist unerlässlich, sich an diese Richtlinien zu halten, um die Einhaltung rechtlicher und ethischer Standards in Forschung und Experiment zu gewährleisten.