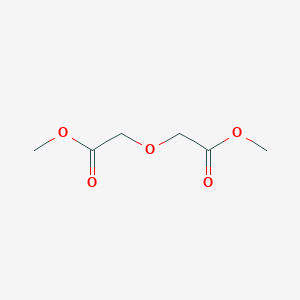
Dimethyldiglycolat
Übersicht
Beschreibung
Dimethyl diglycolate, also known as 2,2’-oxydiethanoic acid dimethyl ester, is a chemical compound with the molecular formula C6H10O5. It is a colorless crystalline solid that is soluble in water and many organic solvents. This compound is primarily used as an intermediate in the synthesis of various chemicals and materials, including high-performance resins and plasticizers .
Wissenschaftliche Forschungsanwendungen
Dimethyl diglycolate has a wide range of applications in scientific research, including:
Chemistry: It is used as a starting material in the synthesis of unsaturated polyester resins and plasticizers.
Medicine: While not directly used as a therapeutic agent, dimethyl diglycolate serves as an intermediate in the synthesis of pharmaceutical compounds.
Wirkmechanismus
Target of Action
Dimethyl diglycolate, also known as 2,2’-oxydiacetic acid dimethyl ester, is a chemical compound used in various industrial applications . . It’s primarily used in the synthesis of high-performance resins such as polyester resins, polyurethane, and photocurable resins .
Mode of Action
The mode of action of dimethyl diglycolate is largely dependent on its chemical properties and the context of its use. As a chemical intermediate, it reacts with other compounds to form new products. For instance, it’s produced by the reaction of diglycolic acid and methanol . .
Biochemical Pathways
It’s worth noting that related compounds, such as dimethyl fumarate, have been studied for their involvement in biological pathways, such as the nuclear factor (erythroid-derived 2)-like 2 (nrf2) pathway .
Pharmacokinetics
It’s known to be a stable compound with low volatility and good solubility in water, alcohols, and ether solvents .
Result of Action
The results of dimethyl diglycolate’s action are largely dependent on its use in chemical reactions. For instance, it’s used as a raw material in the synthesis of high-performance resins, where it contributes to the properties of the final product . .
Biochemische Analyse
Biochemical Properties
It is known that it is a stable compound that is not easily flammable . It is soluble in water, alcohols, and ether solvents
Cellular Effects
It is known that it is a biochemical used in proteomics research , suggesting that it may have some influence on protein function and cellular processes.
Molecular Mechanism
Vorbereitungsmethoden
Synthetic Routes and Reaction Conditions: Dimethyl diglycolate can be synthesized through the esterification of diglycolic acid with methanol. The reaction typically involves the use of thionyl chloride as a catalyst. The process begins by dissolving diglycolic acid in anhydrous methanol under a nitrogen atmosphere and cooling the mixture to 0°C. Thionyl chloride is then added dropwise, and the mixture is allowed to warm to room temperature and stirred overnight. The solvent is removed under reduced pressure, yielding dimethyl diglycolate as a crystalline white solid .
Industrial Production Methods: On an industrial scale, dimethyl diglycolate is produced using similar esterification processes, but with optimized reaction conditions to ensure high yield and purity. The use of continuous flow reactors and advanced purification techniques helps in achieving large-scale production efficiently .
Analyse Chemischer Reaktionen
Types of Reactions: Dimethyl diglycolate undergoes various chemical reactions, including:
Hydrogenation: It can be hydrogenated to produce methyl glycolate using silver catalysts supported on silica (Ag/SiO2).
Hydrolysis: The compound can be hydrolyzed to yield diglycolic acid and methanol. This reaction is usually performed in the presence of water and an acid or base catalyst.
Common Reagents and Conditions:
Hydrogenation: Silver catalysts supported on silica (Ag/SiO2) are commonly used for the hydrogenation of dimethyl diglycolate.
Hydrolysis: Acidic or basic conditions are employed for the hydrolysis of dimethyl diglycolate. Common acids include hydrochloric acid and sulfuric acid, while bases such as sodium hydroxide are also used.
Major Products Formed:
Hydrogenation: Methyl glycolate
Hydrolysis: Diglycolic acid and methanol
Vergleich Mit ähnlichen Verbindungen
Dimethyl diglycolate can be compared with other similar compounds such as:
Dimethyl oxalate: Both compounds are esters and undergo similar reactions, but dimethyl oxalate is more commonly used in the production of ethylene glycol.
Diglycolic acid: This is the parent compound of dimethyl diglycolate and is used in similar applications, but it is more reactive due to the presence of free carboxylic acid groups.
Methyl glycolate: This is a hydrogenation product of dimethyl diglycolate and is used in the synthesis of various fine chemicals and intermediates.
Dimethyl diglycolate is unique in its ability to serve as a versatile intermediate in the synthesis of high-performance materials and its relatively mild reaction conditions compared to other esters .
Eigenschaften
IUPAC Name |
methyl 2-(2-methoxy-2-oxoethoxy)acetate | |
---|---|---|
Source | PubChem | |
URL | https://pubchem.ncbi.nlm.nih.gov | |
Description | Data deposited in or computed by PubChem | |
InChI |
InChI=1S/C6H10O5/c1-9-5(7)3-11-4-6(8)10-2/h3-4H2,1-2H3 | |
Source | PubChem | |
URL | https://pubchem.ncbi.nlm.nih.gov | |
Description | Data deposited in or computed by PubChem | |
InChI Key |
KUCRTUQUAYLJDC-UHFFFAOYSA-N | |
Source | PubChem | |
URL | https://pubchem.ncbi.nlm.nih.gov | |
Description | Data deposited in or computed by PubChem | |
Canonical SMILES |
COC(=O)COCC(=O)OC | |
Source | PubChem | |
URL | https://pubchem.ncbi.nlm.nih.gov | |
Description | Data deposited in or computed by PubChem | |
Molecular Formula |
C6H10O5 | |
Source | PubChem | |
URL | https://pubchem.ncbi.nlm.nih.gov | |
Description | Data deposited in or computed by PubChem | |
DSSTOX Substance ID |
DTXSID30220673 | |
Record name | Acetic acid, oxydi-, dimethyl ester | |
Source | EPA DSSTox | |
URL | https://comptox.epa.gov/dashboard/DTXSID30220673 | |
Description | DSSTox provides a high quality public chemistry resource for supporting improved predictive toxicology. | |
Molecular Weight |
162.14 g/mol | |
Source | PubChem | |
URL | https://pubchem.ncbi.nlm.nih.gov | |
Description | Data deposited in or computed by PubChem | |
CAS No. |
7040-23-5 | |
Record name | 1,1′-Dimethyl 2,2′-oxybis[acetate] | |
Source | CAS Common Chemistry | |
URL | https://commonchemistry.cas.org/detail?cas_rn=7040-23-5 | |
Description | CAS Common Chemistry is an open community resource for accessing chemical information. Nearly 500,000 chemical substances from CAS REGISTRY cover areas of community interest, including common and frequently regulated chemicals, and those relevant to high school and undergraduate chemistry classes. This chemical information, curated by our expert scientists, is provided in alignment with our mission as a division of the American Chemical Society. | |
Explanation | The data from CAS Common Chemistry is provided under a CC-BY-NC 4.0 license, unless otherwise stated. | |
Record name | Acetic acid, oxydi-, dimethyl ester | |
Source | ChemIDplus | |
URL | https://pubchem.ncbi.nlm.nih.gov/substance/?source=chemidplus&sourceid=0007040235 | |
Description | ChemIDplus is a free, web search system that provides access to the structure and nomenclature authority files used for the identification of chemical substances cited in National Library of Medicine (NLM) databases, including the TOXNET system. | |
Record name | Acetic acid, oxydi-, dimethyl ester | |
Source | EPA DSSTox | |
URL | https://comptox.epa.gov/dashboard/DTXSID30220673 | |
Description | DSSTox provides a high quality public chemistry resource for supporting improved predictive toxicology. | |
Synthesis routes and methods
Procedure details
Retrosynthesis Analysis
AI-Powered Synthesis Planning: Our tool employs the Template_relevance Pistachio, Template_relevance Bkms_metabolic, Template_relevance Pistachio_ringbreaker, Template_relevance Reaxys, Template_relevance Reaxys_biocatalysis model, leveraging a vast database of chemical reactions to predict feasible synthetic routes.
One-Step Synthesis Focus: Specifically designed for one-step synthesis, it provides concise and direct routes for your target compounds, streamlining the synthesis process.
Accurate Predictions: Utilizing the extensive PISTACHIO, BKMS_METABOLIC, PISTACHIO_RINGBREAKER, REAXYS, REAXYS_BIOCATALYSIS database, our tool offers high-accuracy predictions, reflecting the latest in chemical research and data.
Strategy Settings
Precursor scoring | Relevance Heuristic |
---|---|
Min. plausibility | 0.01 |
Model | Template_relevance |
Template Set | Pistachio/Bkms_metabolic/Pistachio_ringbreaker/Reaxys/Reaxys_biocatalysis |
Top-N result to add to graph | 6 |
Feasible Synthetic Routes
Q1: What is the main application of dimethyl diglycolate highlighted in the provided research papers?
A: The research papers primarily focus on utilizing dimethyl diglycolate as a starting material for synthesizing various 3,4-disubstituted furan-2,5-dicarboxylic acids. [, , ] This is achieved through a condensation reaction with different 1,2-carbonyl compounds like benzil, glyoxal trimer dihydrate, and diethyl oxalate.
Q2: What are the advantages of the synthesis methods described in these papers?
A2: The described synthesis methods offer several advantages:
- Conciseness: The reactions are generally one-step procedures, simplifying the synthesis process. [, ]
- Efficiency: The reported yields for the target furan-2,5-dicarboxylic acids are high, ranging from 69.1% to 98.6%. [, ]
- Versatility: By varying the 1,2-carbonyl compound used, different 3,4-disubstituted furan-2,5-dicarboxylic acids can be synthesized. [, ]
Q3: What are the differences in reaction conditions used in the presented research?
A3: While both studies utilize dimethyl diglycolate and 1,2-carbonyl compounds for the synthesis, they differ in the specific conditions:
- Solvent: One study employs methanol as the solvent, [] while the other uses cyclohexane. []
- Catalyst: One study uses sodium methoxide (CH3ONa) as a base catalyst, [] while the other uses potassium hydroxide (KOH). []
- Reaction time: Reaction times vary slightly, with one study reporting 6 hours [] and the other 6-8 hours. []
Haftungsausschluss und Informationen zu In-Vitro-Forschungsprodukten
Bitte beachten Sie, dass alle Artikel und Produktinformationen, die auf BenchChem präsentiert werden, ausschließlich zu Informationszwecken bestimmt sind. Die auf BenchChem zum Kauf angebotenen Produkte sind speziell für In-vitro-Studien konzipiert, die außerhalb lebender Organismen durchgeführt werden. In-vitro-Studien, abgeleitet von dem lateinischen Begriff "in Glas", beinhalten Experimente, die in kontrollierten Laborumgebungen unter Verwendung von Zellen oder Geweben durchgeführt werden. Es ist wichtig zu beachten, dass diese Produkte nicht als Arzneimittel oder Medikamente eingestuft sind und keine Zulassung der FDA für die Vorbeugung, Behandlung oder Heilung von medizinischen Zuständen, Beschwerden oder Krankheiten erhalten haben. Wir müssen betonen, dass jede Form der körperlichen Einführung dieser Produkte in Menschen oder Tiere gesetzlich strikt untersagt ist. Es ist unerlässlich, sich an diese Richtlinien zu halten, um die Einhaltung rechtlicher und ethischer Standards in Forschung und Experiment zu gewährleisten.