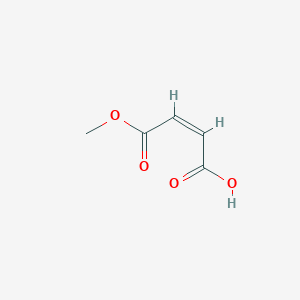
Monomethylmaleat
Übersicht
Beschreibung
Wissenschaftliche Forschungsanwendungen
Monomethylester der Fumarsäure hat verschiedene Anwendungen in der wissenschaftlichen Forschung, insbesondere in den Bereichen Medizin und Biologie:
Behandlung der Multiplen Sklerose: Es wird als krankheitsmodifizierende Therapie eingesetzt, um Schweregrad und Häufigkeit der Symptome der Multiplen Sklerose zu reduzieren.
Entzündungshemmende Wirkungen: Monomethylester der Fumarsäure wurde hinsichtlich seiner potenziellen entzündungshemmenden Wirkungen bei verschiedenen Autoimmunerkrankungen untersucht.
Wirkmechanismus
Monomethylester der Fumarsäure übt seine Wirkungen aus, indem es den NFE2L2-Signalweg aktiviert. Dieser Signalweg reguliert die Expression von antioxidativen Proteinen, die vor oxidativen Schäden schützen, die durch Verletzungen und Entzündungen ausgelöst werden . Durch die Aktivierung von NFE2L2 trägt Monomethylester der Fumarsäure dazu bei, oxidativen Stress und Entzündungen zu reduzieren, die Schlüsselfaktoren für das Fortschreiten der Multiplen Sklerose sind .
Wirkmechanismus
Target of Action
Monomethyl maleate is an unsaturated monomer that belongs to the glycol ether group . It is primarily used in the production of polyvinyl alcohol with strong resistance to water vapor . .
Mode of Action
It is known that monomethyl maleate can be synthesized from maleic anhydride and methanol, with sulfuric acid acting as an acid catalyst, via a nucleophilic acyl substitution for the monomethyl ester .
Biochemical Pathways
Monomethyl maleate may be involved in the esterification of methanol with maleic anhydride . The focus of this reaction is on the production kinetics of monomethyl maleate . .
Pharmacokinetics
It is a colorless liquid that has a melting point of -37°c and a boiling point of 160°c . These properties may influence its bioavailability.
Action Environment
Environmental factors can influence the action, efficacy, and stability of monomethyl maleate. For instance, the esterification of methanol with maleic anhydride has been studied, and the reaction was found to be temperature-dependent . The reaction was carried out in a batch reactor, where the temperature was maintained and controlled by a thermostat .
Biochemische Analyse
Biochemical Properties
Monomethyl maleate is involved in various biochemical reactions. It can be synthesized from maleic anhydride and methanol, with sulfuric acid acting as an acid catalyst, via a nucleophilic acyl substitution for the monomethyl ester, followed by a Fischer esterification reaction for the dimethyl ester
Molecular Mechanism
The molecular mechanism of Monomethyl maleate involves its synthesis from maleic anhydride and methanol . This process involves a nucleophilic acyl substitution for the monomethyl ester, followed by a Fischer esterification reaction for the dimethyl ester . These reactions involve binding interactions with biomolecules, enzyme inhibition or activation, and changes in gene expression.
Metabolic Pathways
Monomethyl maleate is involved in the metabolic pathway of the production of polyvinyl alcohol
Vorbereitungsmethoden
Synthesewege und Reaktionsbedingungen
Monomethylester der Fumarsäure kann durch Veresterung von Fumarsäure mit Methanol synthetisiert werden. Die Reaktion beinhaltet typischerweise die Verwendung eines starken Säurekatalysators, wie z. B. Schwefelsäure, unter Rückflussbedingungen . Die Reaktion verläuft wie folgt:
Fumarsäure+Methanol→Monomethylester der Fumarsäure+Wasser
Industrielle Produktionsverfahren
In industriellen Umgebungen beinhaltet die Produktion von Monomethylester der Fumarsäure ähnliche Veresterungsprozesse, jedoch in größerem Maßstab. Die Reaktionsbedingungen werden optimiert, um eine hohe Ausbeute und Reinheit des Produkts zu gewährleisten. Die Verwendung von Durchflussreaktoren und fortschrittlichen Reinigungsverfahren, wie z. B. Destillation und Kristallisation, sind in der industriellen Produktion weit verbreitet .
Analyse Chemischer Reaktionen
Arten von Reaktionen
Monomethylester der Fumarsäure durchläuft verschiedene Arten chemischer Reaktionen, darunter:
Oxidation: Monomethylester der Fumarsäure kann oxidiert werden, um Fumarsäure zu bilden.
Reduktion: Es kann reduziert werden, um Bernsteinsäure zu bilden.
Häufige Reagenzien und Bedingungen
Oxidation: Häufige Oxidationsmittel sind Kaliumpermanganat und Wasserstoffperoxid.
Reduktion: Als Reduktionsmittel werden Lithiumaluminiumhydrid oder Natriumborhydrid verwendet.
Substitution: Nukleophile wie Hydroxidionen oder Amine können unter basischen Bedingungen verwendet werden.
Hauptprodukte, die gebildet werden
Oxidation: Fumarsäure
Reduktion: Bernsteinsäure
Substitution: Verschiedene substituierte Fumarsäureester, abhängig vom verwendeten Nukleophil.
Vergleich Mit ähnlichen Verbindungen
Monomethylester der Fumarsäure wird häufig mit anderen Fumaraten verglichen, die zur Behandlung der Multiplen Sklerose eingesetzt werden, wie z. B. Dimethylester der Fumarsäure und Diroximel-Fumarat . Hier sind einige wichtige Vergleichspunkte:
Dimethylester der Fumarsäure: Dimethylester der Fumarsäure ist ein Prodrug, das im Magen-Darm-Trakt in Monomethylester der Fumarsäure umgewandelt wird.
Diroximel-Fumarat: Ähnlich wie Dimethylester der Fumarsäure ist Diroximel-Fumarat ebenfalls ein Prodrug, das in Monomethylester der Fumarsäure umgewandelt wird.
Liste ähnlicher Verbindungen
- Dimethylester der Fumarsäure
- Diroximel-Fumarat
- Tecfidera (Handelsname für Dimethylester der Fumarsäure)
- Vumerity (Handelsname für Diroximel-Fumarat)
Eigenschaften
CAS-Nummer |
3052-50-4 |
---|---|
Molekularformel |
C5H6O4 |
Molekulargewicht |
130.10 g/mol |
IUPAC-Name |
4-methoxy-4-oxobut-2-enoic acid |
InChI |
InChI=1S/C5H6O4/c1-9-5(8)3-2-4(6)7/h2-3H,1H3,(H,6,7) |
InChI-Schlüssel |
NKHAVTQWNUWKEO-UHFFFAOYSA-N |
SMILES |
COC(=O)C=CC(=O)O |
Isomerische SMILES |
COC(=O)/C=C/C(=O)O |
Kanonische SMILES |
COC(=O)C=CC(=O)O |
melting_point |
144.5°C |
Key on ui other cas no. |
3052-50-4 2756-87-8 |
Physikalische Beschreibung |
Solid |
Piktogramme |
Irritant |
Synonyme |
(2Z)-2-Butenedioic Acid 1-Methyl Ester; (2Z)-2-Butenedioic Acid Monomethyl Ester ; ; Maleic Acid Methyl Ester; (Z)-4-Methoxy-4-oxobut-2-enoic Acid; (Z)-4-Methoxy-4-oxobut-2-enoic Acid; Hydrogen Methyl Maleate; Methyl Hydrogen (2Z)-2-Butendioate; Meth |
Herkunft des Produkts |
United States |
Retrosynthesis Analysis
AI-Powered Synthesis Planning: Our tool employs the Template_relevance Pistachio, Template_relevance Bkms_metabolic, Template_relevance Pistachio_ringbreaker, Template_relevance Reaxys, Template_relevance Reaxys_biocatalysis model, leveraging a vast database of chemical reactions to predict feasible synthetic routes.
One-Step Synthesis Focus: Specifically designed for one-step synthesis, it provides concise and direct routes for your target compounds, streamlining the synthesis process.
Accurate Predictions: Utilizing the extensive PISTACHIO, BKMS_METABOLIC, PISTACHIO_RINGBREAKER, REAXYS, REAXYS_BIOCATALYSIS database, our tool offers high-accuracy predictions, reflecting the latest in chemical research and data.
Strategy Settings
Precursor scoring | Relevance Heuristic |
---|---|
Min. plausibility | 0.01 |
Model | Template_relevance |
Template Set | Pistachio/Bkms_metabolic/Pistachio_ringbreaker/Reaxys/Reaxys_biocatalysis |
Top-N result to add to graph | 6 |
Feasible Synthetic Routes
Q1: What are the common methods for synthesizing monomethyl maleate?
A1: Monomethyl maleate is primarily synthesized through the esterification of maleic anhydride with methanol. This reaction can be carried out in various setups, including batch reactors [] and continuous production systems [, ]. The use of catalysts like sulfuric acid can enhance the reaction rate and yield [, ].
Q2: What challenges are associated with the industrial production of dimethyl maleate, and how do newer methods address them?
A2: Traditional methods for dimethyl maleate production often face issues like high catalyst consumption, uneven catalyst distribution in reaction towers, and limited energy efficiency. To tackle these challenges, innovative approaches have been developed, such as:
- Continuous production processes: Utilizing the waste heat from the esterification reaction to enhance energy efficiency [].
- Multi-stage methanol steam input: Optimizing methanol distribution and contact time within the reaction tower to improve yield and reduce production costs [, ].
- Fixed-bed reactors: Employing fixed-bed reactors with specific catalysts to enhance conversion rates and minimize catalyst replacement frequency [].
Q3: What is the molecular structure of monomethyl maleate, and how is it confirmed?
A3: Monomethyl maleate possesses a single ester group linked to the maleic acid backbone. Its structure is characterized by a carbon-carbon double bond, influencing its reactivity. Analytical techniques like 1H-NMR, 13C-NMR, and IR spectroscopy are employed to confirm the structure and study the compound's behavior [, ].
Q4: How does the presence of the double bond in monomethyl maleate influence its reactivity?
A4: The double bond in monomethyl maleate makes it susceptible to various reactions, including:
- Addition reactions: The double bond readily undergoes addition reactions, as evidenced by its reaction with sodium hypophosphite to modify wood properties [].
- Polymerization: Monomethyl maleate serves as a monomer in polymerization reactions with styrene, vinyl acetate, and methyl methacrylate, yielding polymers with distinct properties [, ].
- Isomerization: Under specific conditions and with appropriate catalysts, like hydrochloric acid, monomethyl maleate can undergo isomerization to form monomethyl fumarate [].
Q5: How does the esterification of maleic acid to form monomethyl maleate impact its properties?
A5: The esterification of maleic acid significantly influences its reactivity and physical characteristics. For instance, monomethyl maleate exhibits different fragmentation patterns compared to maleic acid during mass spectrometry analysis []. Furthermore, the presence of the ester group modifies its solubility and interaction with other molecules.
Q6: How is monomethyl maleate utilized in material science and polymer chemistry?
A6: Monomethyl maleate plays a crucial role as a building block in synthesizing various polymers. It's copolymerized with other monomers to create materials with specific properties:
- Cross-linked ultrathin films: Synthesized using layer-by-layer assembly with polymers like poly(allylamine hydrochloride), resulting in films with controlled thickness and selective permeability [, ].
- Water-reducing and slump-retaining agents: Used in concrete admixtures to enhance workability and performance [, ].
- Plant oil-based polymers: Reacting epoxidized soybean oil with monomethyl maleate produces monomers for environmentally friendly liquid molding resins [].
Q7: What are the potential applications of monomethyl maleate derivatives in the pharmaceutical industry?
A7: Derivatives of monomethyl maleate, like monomethyl fumarate (MMF), hold promise as therapeutic agents. MMF, synthesized via the isomerization of monomethyl maleate [, ], demonstrates antimicrobial activity against various microorganisms []. Research is ongoing to explore its potential in treating conditions like psoriasis and multiple sclerosis, focusing on developing prodrugs with improved bioavailability [].
Q8: How is monomethyl maleate used to study the stabilization mechanisms of poly(vinyl chloride)?
A8: Researchers utilized radioactively tagged monomethyl maleate in organotin stabilizers to investigate their interaction with poly(vinyl chloride) (PVC) []. This study revealed insights into the formation of associative complexes between the stabilizer and PVC, shedding light on the stabilization mechanism against degradation.
Haftungsausschluss und Informationen zu In-Vitro-Forschungsprodukten
Bitte beachten Sie, dass alle Artikel und Produktinformationen, die auf BenchChem präsentiert werden, ausschließlich zu Informationszwecken bestimmt sind. Die auf BenchChem zum Kauf angebotenen Produkte sind speziell für In-vitro-Studien konzipiert, die außerhalb lebender Organismen durchgeführt werden. In-vitro-Studien, abgeleitet von dem lateinischen Begriff "in Glas", beinhalten Experimente, die in kontrollierten Laborumgebungen unter Verwendung von Zellen oder Geweben durchgeführt werden. Es ist wichtig zu beachten, dass diese Produkte nicht als Arzneimittel oder Medikamente eingestuft sind und keine Zulassung der FDA für die Vorbeugung, Behandlung oder Heilung von medizinischen Zuständen, Beschwerden oder Krankheiten erhalten haben. Wir müssen betonen, dass jede Form der körperlichen Einführung dieser Produkte in Menschen oder Tiere gesetzlich strikt untersagt ist. Es ist unerlässlich, sich an diese Richtlinien zu halten, um die Einhaltung rechtlicher und ethischer Standards in Forschung und Experiment zu gewährleisten.