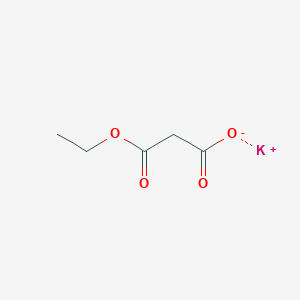
Ethyl potassium malonate
Übersicht
Beschreibung
Ethyl potassium malonate, also known as Monoethyl malonate potassium salt or Potassium monoethyl malonate, is an organic compound with the linear formula C2H5OCOCH2CO2K . It has a molecular weight of 170.20 . It is used as a competitive inhibitor of the enzyme succinate dehydrogenase .
Synthesis Analysis
Ethyl potassium malonate can be synthesized from di-ester of malonic acid which is deprotonated with a weak base, and then undergoes C–C bond formation at the alpha position with an alkyl halide (enolate alkylation). Treatment with aqueous acid results in hydrolysis of the ester . It is also formed as an intermediate during the synthesis of ethyl tert-butyl malonate .Molecular Structure Analysis
The molecular formula of Ethyl potassium malonate is C5H7KO4 . The structure can be represented as [K+].CCOC(=O)CC([O-])=O .Physical And Chemical Properties Analysis
Ethyl potassium malonate is a solid substance . It has a melting point of 194 °C (dec.) . Unfortunately, other physical and chemical properties like density, boiling point, vapour pressure, enthalpy of vaporization, flash point, index of refraction, molar refractivity, etc. are not available .Wissenschaftliche Forschungsanwendungen
Intermediate in Organic Synthesis
Ethyl potassium malonate is used as an intermediate during the synthesis of ethyl tert-butyl malonate . This makes it a valuable compound in the field of organic synthesis where it can be used to create a variety of complex organic molecules.
Production of β-Ketoesters
Ethyl potassium malonate can be used to generate (trimethylsilyl)ethyl malonate in situ, which can be acylated to prepare a variety of β-ketoesters . β-Ketoesters are important compounds in organic chemistry and have applications in pharmaceuticals and other chemical industries.
Preparation of β-Amino Acrylates
Ethyl potassium malonate reacts with aryl nitriles in the presence of zinc chloride and a catalytic amount of Hünig’s base to yield β-amino acrylates . β-Amino acrylates are useful compounds in the synthesis of a variety of pharmaceuticals and other biologically active compounds.
Enzyme Inhibition
Ethyl potassium malonate is used as a competitive inhibitor of the enzyme succinate dehydrogenase . This makes it useful in biochemical research, particularly in studies involving cellular respiration and energy production.
Alkylidene Malonates Preparation
The in situ generated (trimethylsilyl)ethyl malonate from Ethyl potassium malonate can also be used to prepare alkylidene malonates . These compounds are useful in a variety of chemical reactions, including the synthesis of pharmaceuticals and other organic compounds.
Research and Development
Due to its various applications in organic synthesis, Ethyl potassium malonate is a valuable compound in research and development. It can be used to explore new synthetic routes and develop new chemical reactions .
Wirkmechanismus
Target of Action
Ethyl potassium malonate, also known as potassium ethyl malonate, primarily targets the enzyme succinate dehydrogenase . This enzyme plays a crucial role in the citric acid cycle, where it catalyzes the oxidation of succinate to fumarate.
Mode of Action
Ethyl potassium malonate acts as a competitive inhibitor of succinate dehydrogenase . This means it competes with the substrate, succinate, for the active site of the enzyme. When ethyl potassium malonate binds to the enzyme, it prevents the conversion of succinate to fumarate, thereby disrupting the citric acid cycle.
Safety and Hazards
Ethyl potassium malonate is considered hazardous. It is a moderate to severe irritant to the skin and eyes . It is recommended to avoid dust formation, breathing mist, gas or vapours, and contacting with skin and eye. Use of personal protective equipment, chemical-resistant rubber gloves, and chemical safety goggles is advised .
Eigenschaften
IUPAC Name |
potassium;3-ethoxy-3-oxopropanoate | |
---|---|---|
Source | PubChem | |
URL | https://pubchem.ncbi.nlm.nih.gov | |
Description | Data deposited in or computed by PubChem | |
InChI |
InChI=1S/C5H8O4.K/c1-2-9-5(8)3-4(6)7;/h2-3H2,1H3,(H,6,7);/q;+1/p-1 | |
Source | PubChem | |
URL | https://pubchem.ncbi.nlm.nih.gov | |
Description | Data deposited in or computed by PubChem | |
InChI Key |
WVUCPRGADMCTBN-UHFFFAOYSA-M | |
Source | PubChem | |
URL | https://pubchem.ncbi.nlm.nih.gov | |
Description | Data deposited in or computed by PubChem | |
Canonical SMILES |
CCOC(=O)CC(=O)[O-].[K+] | |
Source | PubChem | |
URL | https://pubchem.ncbi.nlm.nih.gov | |
Description | Data deposited in or computed by PubChem | |
Molecular Formula |
C5H7KO4 | |
Source | PubChem | |
URL | https://pubchem.ncbi.nlm.nih.gov | |
Description | Data deposited in or computed by PubChem | |
Molecular Weight |
170.20 g/mol | |
Source | PubChem | |
URL | https://pubchem.ncbi.nlm.nih.gov | |
Description | Data deposited in or computed by PubChem | |
Product Name |
Ethyl potassium malonate | |
CAS RN |
6148-64-7 | |
Record name | Potassium ethyl malonate | |
Source | ChemIDplus | |
URL | https://pubchem.ncbi.nlm.nih.gov/substance/?source=chemidplus&sourceid=0006148647 | |
Description | ChemIDplus is a free, web search system that provides access to the structure and nomenclature authority files used for the identification of chemical substances cited in National Library of Medicine (NLM) databases, including the TOXNET system. | |
Record name | Potassium ethyl malonate | |
Source | European Chemicals Agency (ECHA) | |
URL | https://echa.europa.eu/substance-information/-/substanceinfo/100.025.597 | |
Description | The European Chemicals Agency (ECHA) is an agency of the European Union which is the driving force among regulatory authorities in implementing the EU's groundbreaking chemicals legislation for the benefit of human health and the environment as well as for innovation and competitiveness. | |
Explanation | Use of the information, documents and data from the ECHA website is subject to the terms and conditions of this Legal Notice, and subject to other binding limitations provided for under applicable law, the information, documents and data made available on the ECHA website may be reproduced, distributed and/or used, totally or in part, for non-commercial purposes provided that ECHA is acknowledged as the source: "Source: European Chemicals Agency, http://echa.europa.eu/". Such acknowledgement must be included in each copy of the material. ECHA permits and encourages organisations and individuals to create links to the ECHA website under the following cumulative conditions: Links can only be made to webpages that provide a link to the Legal Notice page. | |
Synthesis routes and methods
Procedure details
Retrosynthesis Analysis
AI-Powered Synthesis Planning: Our tool employs the Template_relevance Pistachio, Template_relevance Bkms_metabolic, Template_relevance Pistachio_ringbreaker, Template_relevance Reaxys, Template_relevance Reaxys_biocatalysis model, leveraging a vast database of chemical reactions to predict feasible synthetic routes.
One-Step Synthesis Focus: Specifically designed for one-step synthesis, it provides concise and direct routes for your target compounds, streamlining the synthesis process.
Accurate Predictions: Utilizing the extensive PISTACHIO, BKMS_METABOLIC, PISTACHIO_RINGBREAKER, REAXYS, REAXYS_BIOCATALYSIS database, our tool offers high-accuracy predictions, reflecting the latest in chemical research and data.
Strategy Settings
Precursor scoring | Relevance Heuristic |
---|---|
Min. plausibility | 0.01 |
Model | Template_relevance |
Template Set | Pistachio/Bkms_metabolic/Pistachio_ringbreaker/Reaxys/Reaxys_biocatalysis |
Top-N result to add to graph | 6 |
Feasible Synthetic Routes
Q & A
Q1: What are the primary applications of Ethyl potassium malonate in pharmaceutical synthesis?
A1: Ethyl potassium malonate serves as a crucial building block in synthesizing various pharmaceuticals. For instance, it plays a vital role in synthesizing orbifloxacin, a veterinary fluoroquinolone antibiotic. [] The process involves reacting ethyl potassium malonate with pentafluorobenzoyl chloride, followed by a series of reactions culminating in orbifloxacin formation. [] Similarly, ethyl potassium malonate is employed in synthesizing sitagliptin phosphate, a drug used to manage type 2 diabetes. []
Q2: How does the use of ethyl potassium malonate compare to other methods in terms of efficiency?
A2: Research suggests that using ethyl potassium malonate can offer advantages in terms of efficiency and simplicity. For example, a study demonstrated a streamlined method for producing monoethyl malonate using ethyl potassium malonate. [] This method boasted an impressive 88.5% yield and required fewer steps and less processing time compared to previous approaches. [] This highlights the potential of ethyl potassium malonate to contribute to more efficient and cost-effective synthetic routes for various compounds.
Q3: What analytical techniques are commonly employed to characterize and confirm the presence of ethyl potassium malonate?
A3: Researchers rely on various analytical techniques to confirm the identity and purity of synthesized compounds, including ethyl potassium malonate. For example, in the synthesis of orbifloxacin, researchers utilized Infrared Spectroscopy (IR), proton Nuclear Magnetic Resonance (1H NMR), and Mass Spectrometry (MS) to verify the final product's structure. [] These techniques provide complementary information about the molecule's functional groups, proton environments, and mass-to-charge ratio, respectively, enabling confident confirmation of the synthesized compound.
Haftungsausschluss und Informationen zu In-Vitro-Forschungsprodukten
Bitte beachten Sie, dass alle Artikel und Produktinformationen, die auf BenchChem präsentiert werden, ausschließlich zu Informationszwecken bestimmt sind. Die auf BenchChem zum Kauf angebotenen Produkte sind speziell für In-vitro-Studien konzipiert, die außerhalb lebender Organismen durchgeführt werden. In-vitro-Studien, abgeleitet von dem lateinischen Begriff "in Glas", beinhalten Experimente, die in kontrollierten Laborumgebungen unter Verwendung von Zellen oder Geweben durchgeführt werden. Es ist wichtig zu beachten, dass diese Produkte nicht als Arzneimittel oder Medikamente eingestuft sind und keine Zulassung der FDA für die Vorbeugung, Behandlung oder Heilung von medizinischen Zuständen, Beschwerden oder Krankheiten erhalten haben. Wir müssen betonen, dass jede Form der körperlichen Einführung dieser Produkte in Menschen oder Tiere gesetzlich strikt untersagt ist. Es ist unerlässlich, sich an diese Richtlinien zu halten, um die Einhaltung rechtlicher und ethischer Standards in Forschung und Experiment zu gewährleisten.