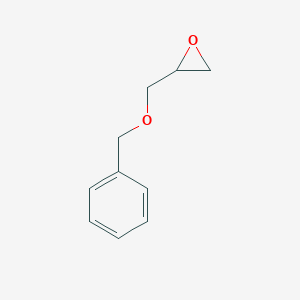
Benzyl glycidyl ether
Übersicht
Beschreibung
Benzyl glycidyl ether (BGE), with the molecular formula C₁₀H₁₂O₂ and molecular weight 164.20 g/mol, is a monofunctional epoxide containing a benzyl group . It exists as a colorless to pale yellow liquid with a boiling point of 130°C at 0.1 mmHg and a density of 1.077 g/mL . BGE is widely used as a reactive diluent in epoxy resins to reduce viscosity and improve processability . Its enantiomeric forms, (R)-(-)-BGE and (S)-(+)-BGE, are critical in asymmetric synthesis, such as producing chiral intermediates for pharmaceuticals like (+)-gigantecin and syn-1,3-polyols .
Vorbereitungsmethoden
Synthetic Routes and Reaction Conditions: Benzyl glycidyl ether can be synthesized through the reaction of epichlorohydrin with benzyl alcohol in the presence of a phase transfer catalyst such as tetrabutylammonium hydrogen sulfate and aqueous sodium hydroxide. The reaction is typically carried out at low temperatures (0°C) to ensure high yield and selectivity .
Industrial Production Methods: In industrial settings, the production of this compound involves the use of continuous flow reactors to maintain optimal reaction conditions and ensure consistent product quality. The process includes the distillation of the product to achieve high purity levels .
Analyse Chemischer Reaktionen
Types of Reactions: Benzyl glycidyl ether undergoes various chemical reactions, including:
Oxidation: The compound can be oxidized to form corresponding diols.
Reduction: It can be reduced to yield alcohols.
Substitution: this compound can participate in nucleophilic substitution reactions, where the glycidyl group is replaced by other nucleophiles.
Common Reagents and Conditions:
Oxidation: Common oxidizing agents include hydrogen peroxide and peracids.
Reduction: Reducing agents such as lithium aluminum hydride are used.
Substitution: Nucleophiles like amines and thiols are commonly employed.
Major Products Formed:
Oxidation: Diols
Reduction: Alcohols
Substitution: Substituted ethers
Wissenschaftliche Forschungsanwendungen
Chemical Properties and Structure
Benzyl glycidyl ether is characterized by its molecular formula and a molecular weight of 164.201 g/mol. It exists as a colorless and transparent liquid with low toxicity and volatility, making it suitable for diverse applications in industrial processes .
Epoxy Resins
BGE is primarily utilized as a reactive diluent in epoxy resin formulations. Its incorporation helps to:
- Reduce viscosity : This makes the epoxy easier to process and apply.
- Enhance thermal properties : Compared to other glycidyl ethers, BGE contributes to higher heat distortion temperatures in cured products, improving their performance in high-temperature applications .
Table 1: Comparison of BGE with Other Glycidyl Ethers
Property | This compound | Butyl Glycidyl Ether |
---|---|---|
Viscosity Reduction | High | Moderate |
Heat Distortion Temp. | High | Moderate |
Toxicity | Low | Moderate |
Nanocomposites
In the field of nanocomposites, BGE plays a crucial role as:
- Dispersant for graphene : It enhances the dispersion of graphene within epoxy matrices, leading to improved mechanical properties.
- Interfacial bonding agent : BGE facilitates strong interactions between the graphene and the epoxy matrix through π-π interactions, significantly increasing the strength and modulus of the composite material .
Biocatalytic Applications
This compound is also valuable in biocatalysis for producing enantiomerically enriched compounds. The bioresolution process using microorganisms such as Bacillus alcalophilus allows for:
- Enantiomeric excess production : Controlled culture conditions enable the production of specific enantiomers, which are essential in pharmaceuticals .
Case Study: Bioresolution Process
In a study involving Bacillus alcalophilus, factors such as bacterial age and nutrient composition were optimized to achieve a high enantiomeric excess of BGE derivatives. The results indicated that specific conditions could yield up to 42% enantiomeric excess for certain products .
Pharmaceutical Applications
BGE serves as an intermediate in synthesizing various pharmaceuticals, particularly optically active compounds. Its utility in producing chiral intermediates makes it valuable for developing drugs that require specific stereochemistry .
Table 2: Pharmaceutical Intermediates Derived from BGE
Compound Name | Application Area | Enantiomeric Excess (%) |
---|---|---|
Cryptocarya Diacetate | Antimicrobial | 85 |
Synargentoide A | Anticancer | 90 |
Environmental Considerations
Due to its low toxicity and favorable environmental profile, BGE is increasingly considered for use in eco-friendly formulations. Its stability and reactivity make it an attractive option for producing sustainable materials without compromising performance .
Wirkmechanismus
Benzyl glycidyl ether can be compared with other glycidyl ethers such as methylthis compound and dimethoxylthis compound. These compounds share similar reactivity but differ in their substituents on the phenyl ring, which affects their chemical properties and applications. For instance, methylthis compound has a methyl group, while dimethoxylthis compound has methoxy groups, leading to variations in their reactivity and selectivity in chemical reactions .
Vergleich Mit ähnlichen Verbindungen
Chemical Structure and Physical Properties
BGE is structurally distinct from other glycidyl ethers due to its benzyl substituent, which influences its reactivity and physical properties. Key comparisons include:
Table 1: Physical and Chemical Properties of BGE and Analogous Glycidyl Ethers
Notes:
- BGE’s low viscosity (5–8 mPa·s) makes it ideal for epoxy resin dilution, whereas multifunctional glycidyl ethers like TEGDGE and CHDMDGE have higher viscosities due to branched structures .
- The benzyl group in BGE enhances solubility in organic solvents compared to aliphatic glycidyl ethers like glycidyl methyl ether (GME) .
Reactivity in Polymerization
BGE’s cationic photopolymerization behavior differs significantly from multifunctional glycidyl ethers due to differences in oxonium ion stability:
Table 2: Polymerization Characteristics
Key Findings :
- BGE’s shorter induction period and higher front velocity result from its less stable secondary oxonium ion, making it suitable for rapid curing applications .
- Multifunctional epoxides like TEGDGE and pentaerythritol tetraglycidyl ether (PETGE) form highly cross-linked networks with longer induction times, ideal for high-temperature-resistant materials .
Enantioselective Hydrolysis
BGE’s chiral resolution via biocatalytic hydrolysis is a key distinction from non-chiral glycidyl ethers. Enzymatic selectivity varies by microbial source:
Table 3: Enantioselectivity in Hydrolysis
Notes:
Biologische Aktivität
Benzyl glycidyl ether (BGE), a compound with the chemical formula CHO, is a versatile organic molecule that exhibits significant biological activity. This article delves into its mechanisms of action, biochemical pathways, and effects on various biological systems, supported by relevant case studies and research findings.
BGE primarily interacts with epoxide hydrolase (EH) enzymes, which catalyze the hydrolysis of epoxides to their corresponding diols. This reaction is crucial for the production of chiral epoxides, which have applications in pharmaceuticals and organic synthesis. The interaction of BGE with EH has been shown to facilitate the resolution of racemic mixtures into optically pure compounds, enhancing its utility in drug synthesis .
Biochemical Pathways
The primary biochemical pathway influenced by BGE is the etherification process of glycerol . This process is vital for synthesizing various drugs and natural products. The biocatalytic resolution of BGE by microorganisms such as Talaromyces flavus has been documented, highlighting the role of microbial metabolism in its biological activity .
Pharmacokinetics
Research indicates that BGE can be metabolized by specific microorganisms, which suggests a potential for biotransformation in various environments. The optimization of fermentation conditions can significantly enhance the enantioselectivity of BGE's hydrolysis, leading to higher yields of desired enantiomers .
Inhalation Studies
Inhalation studies conducted on rats exposed to BGE vapors have revealed potential toxic effects, including testicular atrophy at concentrations as high as 400 mg/m³. Symptoms observed in these studies included respiratory irritation and central nervous system effects such as delirium and depression .
Sensitization
Human case reports indicate that BGE may cause skin sensitization, leading to irritation characterized by erythema and vesiculation upon contact with undiluted samples .
Case Studies and Research Findings
- Kinetic Resolution : A study demonstrated the kinetic resolution of racemic BGE using recombinant epoxide hydrolase from Yarrowia lipolytica. The process yielded (R)-BGE with high enantiomeric excess (ee), showcasing its potential in producing chiral intermediates for pharmaceuticals .
- Microbial Metabolism : The use of Bacillus alcalophilus for the bioresolution of BGE has been explored. Optimizing culture conditions such as nitrogen sources and pH significantly improved the yield and enantiomeric purity of the products derived from BGE .
- Polymer Applications : BGE has been incorporated into polymer formulations due to its low toxicity and ability to enhance mechanical properties. Its role as a reactive diluent in epoxy resins has been particularly noted for improving strength and viscosity characteristics in composite materials .
Summary Table: Biological Activity Overview
Aspect | Details |
---|---|
Chemical Formula | CHO |
Primary Target | Epoxide hydrolase (EH) |
Biochemical Pathway | Etherification process of glycerol |
Toxicological Effects | Respiratory irritation, skin sensitization |
Microbial Metabolism | Effective biotransformation using Talaromyces flavus and Bacillus alcalophilus |
Applications | Drug synthesis, polymer formulations, chiral intermediates |
Q & A
Basic Research Questions
Q. What are the standard analytical methods for determining the purity and epoxy value of Benzyl Glycidyl Ether (BGE)?
To ensure quality control, researchers should employ:
- Epoxy Value Titration : Measure epoxy content (eq/100g) via acid titration, adhering to thresholds ≥0.43 eq/100g .
- Viscosity Analysis : Use a viscometer at 25°C, targeting 5–8 mPa·s .
- Gas Chromatography (GC) : For kinetic studies or purity checks, GC with short analysis times (<15 minutes per injection) effectively separates BGE from byproducts .
- Chlorine Content Testing : Quantify organic (≤0.02 eq/100g) and inorganic chlorine (≤0.001 eq/100g) to assess reactivity and side reactions .
Table 1: Key Specifications for BGE
Parameter | Value/Range | Method |
---|---|---|
Epoxy Value | ≥0.43 eq/100g | Acid titration |
Viscosity (25°C) | 5–8 mPa·s | Viscometer |
Organic Chlorine | ≤0.02 eq/100g | Volumetric analysis |
Moisture Content | ≤0.1% | Karl Fischer titration |
Q. How does BGE function as a reactive diluent in epoxy resin formulations?
BGE reduces epoxy resin viscosity while maintaining thermomechanical properties. Methodological considerations include:
- Dilution Efficiency : BGE’s low viscosity (5–8 mPa·s) enables homogeneous mixing without phase separation .
- Curing Optimization : Adjust stoichiometry with amines or anhydrides; BGE’s epoxy group participates in crosslinking, enhancing flexibility .
- Thermal Stability : Post-curing, BGE-modified resins retain stability up to 200°C, suitable for electronics and coatings .
Q. What safety protocols are essential when handling BGE in laboratory settings?
- Ventilation : Use fume hoods to avoid inhalation; BGE emits vapors above 70°C .
- Personal Protective Equipment (PPE) : Nitrile gloves and goggles mitigate skin/eye irritation (Risk Phrase R36/37/38) .
- Storage : Keep at 0–6°C in sealed containers to prevent polymerization .
- Waste Disposal : Neutralize residual epoxide groups before disposal to avoid uncontrolled reactions .
Advanced Research Questions
Q. What methodologies enhance the enantioselectivity of epoxide hydrolases (EHs) in resolving racemic BGE?
- Enzyme Selection : Use EH from Talaromyces flavus or Agromyces mediolanus for (R)-enantiomer resolution (e.e. >96%) .
- Fermentation Optimization : Increase biomass (14.8 → 31.3 g DCW/L) and EH activity (13.5 → 26.2 U/g DCW) via pH control (7.0–7.5) and carbon source tuning .
- Substituent Effects : Avoid phenyl ring modifications (e.g., nitro or methyl groups), which reduce enantioselectivity (E <5) .
Table 2: Optimization of EH Activity in Talaromyces flavus
Parameter | Initial Value | Optimized Value |
---|---|---|
Biomass Concentration | 14.8 g DCW/L | 31.3 g DCW/L |
Specific EH Activity | 13.5 U/g DCW | 26.2 U/g DCW |
Enantiomeric Excess (e.e.) | 90% | 96% |
Q. How do structural modifications of BGE influence its reactivity in polymer chemistry?
- Side-Chain Engineering : Replace benzyl with alkyl groups (e.g., butyl glycidyl ether) to alter hydrophobicity and curing rates .
- Steric Effects : Bulky substituents reduce crosslinking density but improve fracture toughness .
- Epoxide Ring Stability : Electron-withdrawing groups (e.g., nitro) accelerate ring-opening reactions with nucleophiles .
Q. How can researchers resolve contradictions in reported toxicity profiles of glycidyl ether derivatives?
- Contextual Analysis : BGE itself is classified as an irritant (Xi), while 1-butyl glycidyl ether is Group 2B carcinogenic; differentiate handling protocols .
- Dose-Response Studies : Conduct in vitro assays (e.g., Ames test) to assess mutagenicity thresholds for BGE derivatives .
- Comparative Toxicology : Cross-reference Safety Data Sheets (SDS) and IARC classifications to align lab protocols with regulatory standards .
Q. What experimental strategies optimize BGE’s application in enantioselective synthesis of triglycerides?
- Chiral Intermediate Preparation : Use (S)-(+)-BGE to synthesize 1-octadecyl-3-O-benzyl-sn-glycerol, a precursor for α-eleostearic acid triglycerides .
- Catalytic Asymmetry : Employ lipases or esterases under anhydrous conditions to preserve BGE’s stereochemical integrity .
Eigenschaften
IUPAC Name |
2-(phenylmethoxymethyl)oxirane | |
---|---|---|
Source | PubChem | |
URL | https://pubchem.ncbi.nlm.nih.gov | |
Description | Data deposited in or computed by PubChem | |
InChI |
InChI=1S/C10H12O2/c1-2-4-9(5-3-1)6-11-7-10-8-12-10/h1-5,10H,6-8H2 | |
Source | PubChem | |
URL | https://pubchem.ncbi.nlm.nih.gov | |
Description | Data deposited in or computed by PubChem | |
InChI Key |
QNYBOILAKBSWFG-UHFFFAOYSA-N | |
Source | PubChem | |
URL | https://pubchem.ncbi.nlm.nih.gov | |
Description | Data deposited in or computed by PubChem | |
Canonical SMILES |
C1C(O1)COCC2=CC=CC=C2 | |
Source | PubChem | |
URL | https://pubchem.ncbi.nlm.nih.gov | |
Description | Data deposited in or computed by PubChem | |
Molecular Formula |
C10H12O2 | |
Source | PubChem | |
URL | https://pubchem.ncbi.nlm.nih.gov | |
Description | Data deposited in or computed by PubChem | |
DSSTOX Substance ID |
DTXSID001019317 | |
Record name | 2‐[(Benzyloxy)methyl]oxirane | |
Source | EPA DSSTox | |
URL | https://comptox.epa.gov/dashboard/DTXSID001019317 | |
Description | DSSTox provides a high quality public chemistry resource for supporting improved predictive toxicology. | |
Molecular Weight |
164.20 g/mol | |
Source | PubChem | |
URL | https://pubchem.ncbi.nlm.nih.gov | |
Description | Data deposited in or computed by PubChem | |
CAS No. |
2930-05-4 | |
Record name | Benzyl glycidyl ether | |
Source | CAS Common Chemistry | |
URL | https://commonchemistry.cas.org/detail?cas_rn=2930-05-4 | |
Description | CAS Common Chemistry is an open community resource for accessing chemical information. Nearly 500,000 chemical substances from CAS REGISTRY cover areas of community interest, including common and frequently regulated chemicals, and those relevant to high school and undergraduate chemistry classes. This chemical information, curated by our expert scientists, is provided in alignment with our mission as a division of the American Chemical Society. | |
Explanation | The data from CAS Common Chemistry is provided under a CC-BY-NC 4.0 license, unless otherwise stated. | |
Record name | Propane, 1-(benzyloxy)-2,3-epoxy- | |
Source | ChemIDplus | |
URL | https://pubchem.ncbi.nlm.nih.gov/substance/?source=chemidplus&sourceid=0002930054 | |
Description | ChemIDplus is a free, web search system that provides access to the structure and nomenclature authority files used for the identification of chemical substances cited in National Library of Medicine (NLM) databases, including the TOXNET system. | |
Record name | 2‐[(Benzyloxy)methyl]oxirane | |
Source | EPA DSSTox | |
URL | https://comptox.epa.gov/dashboard/DTXSID001019317 | |
Description | DSSTox provides a high quality public chemistry resource for supporting improved predictive toxicology. | |
Synthesis routes and methods I
Procedure details
Synthesis routes and methods II
Procedure details
Synthesis routes and methods III
Procedure details
Retrosynthesis Analysis
AI-Powered Synthesis Planning: Our tool employs the Template_relevance Pistachio, Template_relevance Bkms_metabolic, Template_relevance Pistachio_ringbreaker, Template_relevance Reaxys, Template_relevance Reaxys_biocatalysis model, leveraging a vast database of chemical reactions to predict feasible synthetic routes.
One-Step Synthesis Focus: Specifically designed for one-step synthesis, it provides concise and direct routes for your target compounds, streamlining the synthesis process.
Accurate Predictions: Utilizing the extensive PISTACHIO, BKMS_METABOLIC, PISTACHIO_RINGBREAKER, REAXYS, REAXYS_BIOCATALYSIS database, our tool offers high-accuracy predictions, reflecting the latest in chemical research and data.
Strategy Settings
Precursor scoring | Relevance Heuristic |
---|---|
Min. plausibility | 0.01 |
Model | Template_relevance |
Template Set | Pistachio/Bkms_metabolic/Pistachio_ringbreaker/Reaxys/Reaxys_biocatalysis |
Top-N result to add to graph | 6 |
Feasible Synthetic Routes
Haftungsausschluss und Informationen zu In-Vitro-Forschungsprodukten
Bitte beachten Sie, dass alle Artikel und Produktinformationen, die auf BenchChem präsentiert werden, ausschließlich zu Informationszwecken bestimmt sind. Die auf BenchChem zum Kauf angebotenen Produkte sind speziell für In-vitro-Studien konzipiert, die außerhalb lebender Organismen durchgeführt werden. In-vitro-Studien, abgeleitet von dem lateinischen Begriff "in Glas", beinhalten Experimente, die in kontrollierten Laborumgebungen unter Verwendung von Zellen oder Geweben durchgeführt werden. Es ist wichtig zu beachten, dass diese Produkte nicht als Arzneimittel oder Medikamente eingestuft sind und keine Zulassung der FDA für die Vorbeugung, Behandlung oder Heilung von medizinischen Zuständen, Beschwerden oder Krankheiten erhalten haben. Wir müssen betonen, dass jede Form der körperlichen Einführung dieser Produkte in Menschen oder Tiere gesetzlich strikt untersagt ist. Es ist unerlässlich, sich an diese Richtlinien zu halten, um die Einhaltung rechtlicher und ethischer Standards in Forschung und Experiment zu gewährleisten.