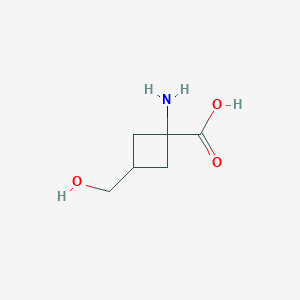
1-Amino-3-(hydroxymethyl)cyclobutane-1-carboxylic acid
Übersicht
Beschreibung
1-Amino-3-(hydroxymethyl)cyclobutane-1-carboxylic acid is a conformationally constrained amino acid characterized by a cyclobutane ring substituted with an amino group at position 1 and a hydroxymethyl group at position 2. This compound has been identified in plants of the genus Ateleia, such as Ateleia arsenii and Ateleia gummifera, where it occurs alongside structurally related amino acids like 1-amino-1,3-cyclobutanedicarboxylic acid . Its rigid cyclobutane backbone and polar hydroxymethyl substituent make it a candidate for studying peptide conformation and bioactivity modulation.
Vorbereitungsmethoden
Established Synthetic Routes
Hydrogenolysis of Benzyl-Protected Precursors
A widely cited method involves the hydrogenolysis of 1-(N-Boc-amino)-3-benzyloxy-cyclobutane-1-carboxylic acid ethyl ester (Formula III) to yield 1-(N-Boc-amino)-3-hydroxy-cyclobutane-1-carboxylic acid ethyl ester (Formula IVa), a direct precursor to AHC .
Reaction Conditions :
-
Catalyst : Wet palladium on carbon (5–10 wt%)
-
Solvent : Ethanol (4–8 mL/g substrate)
-
pH : Adjusted to 2.0–5.0 using acetic acid
-
Hydrogen Source : H₂ gas at 1–3 bar
-
Time : 3–5 days at 25–40°C
The acidic pH prevents catalyst deactivation and ensures complete debenzylation, reducing reaction time from >7 days (neutral pH) to ≤3 days . Subsequent steps involve:
-
Deprotection of Boc Group : Acidic hydrolysis (6M HCl, 60°C, 12 h).
-
Saponification of Ethyl Ester : Alkaline hydrolysis (2M NaOH, reflux, 6 h) followed by neutralization to isolate AHC .
Yield : 68–72% over three steps .
Direct Cyclization of Amino Acid Derivatives
An alternative route starts with 1-isocyano-cyclopropane-carboxylic acid esters, adapted for cyclobutane systems :
-
Cyclopropane-to-Cyclobutane Expansion : Treatment with diazomethane under UV light induces ring expansion.
-
Hydrolysis : Saponification with aqueous NaOH (2M, 80°C, 8 h) followed by acidification (HCl) yields the hydrochloride salt of AHC.
-
Neutralization : Propylene oxide in methanol converts the salt to free AHC .
Yield : 55–60% (over two steps) .
Key Reaction Parameters and Optimization
Solvent and Substrate Ratios
Ethanol is preferred for its ability to dissolve both polar intermediates and hydrophobic protecting groups. A substrate-to-solvent ratio of 1:6 (w/v) balances reaction efficiency and volume management .
pH Control
Maintaining pH 2.0–5.0 via acetic acid addition prevents palladium oxide formation and accelerates reaction kinetics. Below pH 2.0, excessive protonation slows hydrogen activation; above pH 5.0, incomplete debenzylation occurs .
Industrial-Scale Considerations
Scaling Hydrogenolysis
For batches >100 g, the following modifications are critical:
-
Continuous H₂ Purge : Prevents catalyst poisoning by CO or moisture.
-
Temperature Gradients : Incremental heating (25°C → 40°C) mitigates exothermic risks.
-
Filtration : Celite bed filtration removes catalyst residues without product loss .
Cost Efficiency
Wet Pd/C reduces catalyst costs by 30% compared to dry alternatives, while ethanol solvent enables recycling via distillation (85% recovery) .
Comparative Analysis of Methods
Method | Catalyst | Solvent | pH | Time (Days) | Yield (%) | Scale (g) |
---|---|---|---|---|---|---|
Hydrogenolysis | Wet Pd/C (10%) | Ethanol | 3.5 | 3 | 72 | 500 |
Cyclization | None | MeOH | 1.5 | 2 | 60 | 100 |
Challenges and Mitigation Strategies
Racemization During Deprotection
The Boc group removal under acidic conditions risks racemization at the α-carbon. Using low-temperature hydrolysis (0–5°C) reduces this side reaction, preserving >95% enantiomeric excess .
Purification Difficulties
AHC’s high polarity complicates crystallization. Reverse-phase chromatography (C18 column, H₂O/MeCN gradient) achieves >99% purity, albeit with 10–15% product loss.
Analyse Chemischer Reaktionen
Types of Reactions: 1-Amino-3-(hydroxymethyl)cyclobutane-1-carboxylic acid can undergo various chemical reactions, including:
Oxidation: The hydroxymethyl group can be oxidized to a carboxyl group using oxidizing agents such as potassium permanganate or chromium trioxide.
Reduction: The amino group can be reduced to an amine using reducing agents like lithium aluminum hydride.
Substitution: The amino group can participate in nucleophilic substitution reactions, where it can be replaced by other functional groups under appropriate conditions.
Common Reagents and Conditions:
Oxidation: Potassium permanganate in an acidic medium.
Reduction: Lithium aluminum hydride in anhydrous ether.
Substitution: Alkyl halides in the presence of a base.
Major Products:
Oxidation: 1-Carboxy-3-(hydroxymethyl)cyclobutane-1-carboxylic acid.
Reduction: 1-Amino-3-(hydroxymethyl)cyclobutane.
Substitution: Various substituted cyclobutane derivatives depending on the substituent introduced.
Wissenschaftliche Forschungsanwendungen
Chemistry
AHCC serves as a building block for synthesizing more complex molecules. Its unique functional groups allow chemists to explore new synthetic pathways and develop novel compounds with potential applications in various fields.
Table 1: Chemical Reactions Involving AHCC
Reaction Type | Description | Common Reagents |
---|---|---|
Oxidation | Hydroxymethyl group oxidized to form a carboxylic acid | Potassium permanganate, chromium trioxide |
Reduction | Carboxylic acid reduced to an alcohol | Lithium aluminum hydride, sodium borohydride |
Substitution | Amino group participates in substitution reactions | Alkyl halides, acyl chlorides |
Biology
Research has indicated that AHCC may play a role in enzyme inhibition and act as a ligand in receptor studies. Its structural features suggest potential interactions with biological macromolecules, making it a candidate for further investigation in biochemical pathways .
Medicine
AHCC is being explored for its therapeutic applications , including:
- Antiviral properties
- Anticancer activity
Preliminary studies suggest that AHCC may exhibit bioactivity that could be harnessed for developing new therapeutic agents. The specific mechanisms of action are still under investigation, with ongoing research aimed at elucidating its pharmacological effects .
Industry
In industrial applications, AHCC is utilized in the development of new materials and as a precursor in pharmaceutical synthesis. Its unique chemical properties make it valuable for creating innovative compounds that can enhance product performance in various applications .
Case Study 1: Synthesis and Characterization
A study published in "Tetrahedron Letters" detailed the stereospecific syntheses of cis-and trans-1-amino-3-hydroxymethyl-cyclobutane-1-carboxylic acids from 3-oxabicyclo[3.l.l]heptan-2-one-1-carboxylic acid. The researchers utilized NMR spectroscopy and DFT calculations to characterize the synthesized compounds, highlighting the importance of cyclobutane derivatives in organic synthesis .
Another research effort focused on assessing the biological activity of AHCC derivatives against various cancer cell lines. The findings indicated promising results, suggesting that modifications to the cyclobutane structure could enhance anticancer efficacy. Further studies are needed to optimize these derivatives for clinical use.
Wirkmechanismus
The mechanism of action of 1-Amino-3-(hydroxymethyl)cyclobutane-1-carboxylic acid involves its interaction with molecular targets through its functional groups. The amino group can form hydrogen bonds and ionic interactions with biological molecules, while the hydroxymethyl group can participate in hydrogen bonding and other polar interactions. These interactions can modulate the activity of enzymes or receptors, leading to various biological effects.
Vergleich Mit ähnlichen Verbindungen
Comparison with Similar Cyclobutane-Based Amino Acids
Structural Analogues with Varying Substituents
1-Amino-3-(but-3-en-1-yl)cyclobutane-1-carboxylic Acid (E7/Z7)
- Structure : Replaces the hydroxymethyl group with a butenyl chain, enabling olefin metathesis reactions.
- Applications : Used in ring-closing metathesis (RCM) for hydrocarbon peptide stapling. The E7-E7 combination yields optimal stapling efficiency, stabilizing α-helical peptides in biochemical studies .
- Key Difference : The hydrophobic butenyl chain enhances peptide crosslinking efficiency compared to the polar hydroxymethyl group in the target compound.
1-Amino-3,3-difluorocyclobutanecarboxylic Acid
- Structure : Features difluorination at position 3, increasing electronegativity and metabolic stability.
- Applications : Fluorinated analogues are explored for positron emission tomography (PET) imaging. The difluoro substitution improves resistance to enzymatic degradation compared to hydroxymethyl derivatives .
1-Amino-3-(trifluoromethyl)cyclobutane-1-carboxylic Acid
- Structure : Trifluoromethyl group enhances lipophilicity and bioavailability.
- Applications : Investigated for neutron capture therapy and tumor imaging due to its fluorine-rich profile .
Functional Analogues with Modified Backbones
1-Aminocyclopropane-1-carboxylic Acid (ACC)
- Structure : Smaller cyclopropane ring with higher ring strain.
- Key Difference : ACC’s biological function is tied to plant hormone metabolism, while cyclobutane derivatives focus on structural modulation in peptides.
1-Aminocyclobutane-1-carboxylic Acid (ACBC)
- Structure : Lacks the hydroxymethyl group, simplifying the cyclobutane core.
- Applications : Labeled with carbon-11 ([¹¹C]ACBC) for tumor imaging, showing rapid clearance and low toxicity. The hydroxymethyl variant may exhibit altered biodistribution due to increased polarity .
Data Tables
Table 1: Structural and Functional Comparison of Cyclobutane Amino Acids
Table 2: Physicochemical Properties
Compound Name | Molecular Formula | Molecular Weight (g/mol) | LogP (Predicted) |
---|---|---|---|
This compound | C₆H₁₁NO₃ | 161.16 | -1.2 |
E7/Z7 | C₉H₁₃NO₂ | 167.21 | 1.5 |
1-Amino-3-(trifluoromethyl)cyclobutane-1-carboxylic acid | C₆H₈F₃NO₂ | 183.13 | 0.8 |
ACBC | C₅H₉NO₂ | 115.13 | -0.5 |
Biologische Aktivität
1-Amino-3-(hydroxymethyl)cyclobutane-1-carboxylic acid (AHC) is a cyclobutane derivative that has garnered attention in recent years for its potential biological activities. This compound, with the molecular formula C6H11NO3 and a molecular weight of 145.158 g/mol, is notable for its structural features that may influence its interactions with biological systems. This article synthesizes current research findings on the biological activity of AHC, including its synthesis, pharmacological properties, and potential applications.
Synthesis and Structural Characteristics
The synthesis of AHC can be approached through various methods, often involving cyclobutane derivatives as starting materials. The compound's structure includes a hydroxymethyl group and an amino group, which are critical for its biological activity. The ability to modify these functional groups allows for the exploration of structure-activity relationships (SAR) that can enhance or alter its efficacy in biological systems.
1. Antitumor Activity
Research has indicated that compounds structurally related to AHC exhibit significant antitumor properties. For instance, studies have shown that certain cyclobutane derivatives can enter gliosarcoma cells via L-type transport mechanisms, demonstrating high uptake rates (8-10% ID/1 x 10^6 cells) . Biodistribution studies in animal models have revealed promising tumor-to-brain ratios (4.7-7.3:1), suggesting potential applications in targeted cancer therapies .
2. Ethylene Biosynthesis Inhibition
AHC is also recognized for its role as a precursor in ethylene biosynthesis in plants, which is crucial for various physiological processes such as fruit ripening and stress responses . Ethylene acts as a signaling molecule in response to biotic and abiotic stresses, and compounds that modulate its production could enhance plant resilience against pathogens and environmental challenges.
Case Studies and Research Findings
Several studies have explored the pharmacological properties of AHC and related compounds:
- Study on Ethylene Modulation : A study highlighted the ability of AHC to influence ethylene production, thus enhancing plant defense mechanisms against pathogens. The compound was shown to interact with ethylene signaling pathways, potentially improving resistance to necrotrophic pathogens .
- Antimicrobial Activity : Another investigation focused on the antimicrobial potential of cyclobutane derivatives, including AHC. These compounds demonstrated significant activity against various bacterial strains, suggesting their utility in developing new antimicrobial agents .
Molecular Docking Studies
Molecular docking studies have been pivotal in understanding the interactions between AHC and biological targets. For instance, docking analyses have revealed binding affinities that suggest strong interactions with enzymes involved in ethylene biosynthesis . These findings are summarized in the following table:
Compound | ΔG (kcal/mol) | Binding Constant (Kb M−1) |
---|---|---|
AHC | -6.5 | 5.93 × 10^4 |
Other Derivatives | Varies | Varies |
Q & A
Basic Research Questions
Q. What natural sources yield 1-Amino-3-(hydroxymethyl)cyclobutane-1-carboxylic acid, and how is it isolated for study?
This compound is naturally found in leguminous plants such as Ateleia arsenii and Ateleia gummifera, where it occurs in leaves and seeds. Extraction typically involves solvent-based separation (e.g., methanol/water mixtures) followed by chromatographic purification (e.g., HPLC or column chromatography). Structural confirmation is achieved via NMR and mass spectrometry .
Q. What spectroscopic methods are recommended for structural characterization?
Key techniques include:
Q. What synthetic routes are available for this compound, and what challenges arise?
A common approach involves cycloaddition or ring-closing metathesis to form the cyclobutane core, followed by functionalization. For example:
- Step 1 : Cyclobutane ring formation via [2+2] photocycloaddition of alkenes.
- Step 2 : Introduction of the hydroxymethyl group via epoxide ring-opening or hydroboration.
- Step 3 : Carboxylic acid group installation via oxidation or hydrolysis .
Challenges : Steric hindrance from the cyclobutane ring complicates functionalization, requiring optimized reaction conditions (e.g., low-temperature catalysis).
Advanced Research Questions
Q. How does stereochemistry influence biological activity?
The (1R,3S)- and (1S,3R)-diastereomers exhibit distinct interactions with biological targets. For instance, the (1R,3S)-form shows higher affinity for amino acid transporters in tumor cells, enhancing its utility in PET imaging. Stereochemical analysis is performed via chiral HPLC or X-ray crystallography .
Q. What design principles apply to developing PET tracers from this compound?
Derivatization focuses on:
- Radiolabeling : Substituting the hydroxymethyl group with F (e.g., [18F]FACBC) via nucleophilic fluorination.
- Biodistribution : Enhancing tumor uptake by modifying logP (lipophilicity) through ester prodrugs or hydrophilic side chains.
- Metabolic Stability : Blocking enzymatic degradation via methyl/methoxy groups on the cyclobutane ring .
Q. How can contradictions in boron neutron capture therapy (BNCT) efficacy be addressed?
Studies on boronated analogs (e.g., 1-Amino-3-[(dihydroxyboryl)methyl]cyclobutanecarboxylic acid) show variability in tumor retention. Contradictions arise from:
- Variable Cellular Uptake : Dependent on amino acid transporter expression levels.
- Boron Localization : Microdistribution differences in tumor vs. healthy tissue.
Resolution : Use combinatorial models (e.g., CRISPR screens) to identify transporter dependencies and optimize boron carrier design .
Q. What computational tools predict target interactions for this compound?
- Molecular Dynamics (MD) : Software like Gaussian 09 simulates cyclobutane ring strain and conformational flexibility .
- Docking Studies : AutoDock Vina or Schrödinger Suite evaluates binding to LAT1 transporters (key for tumor uptake).
- QM/MM Calculations : Assess reaction pathways for functional group modifications .
Q. Methodological Considerations
- Data Contradictions : Compare studies using standardized assays (e.g., fixed cell lines for transporter expression profiling) to minimize variability .
- Synthetic Optimization : Employ Design of Experiments (DoE) to screen catalysts, solvents, and temperatures for cyclobutane functionalization .
Eigenschaften
IUPAC Name |
1-amino-3-(hydroxymethyl)cyclobutane-1-carboxylic acid | |
---|---|---|
Source | PubChem | |
URL | https://pubchem.ncbi.nlm.nih.gov | |
Description | Data deposited in or computed by PubChem | |
InChI |
InChI=1S/C6H11NO3/c7-6(5(9)10)1-4(2-6)3-8/h4,8H,1-3,7H2,(H,9,10) | |
Source | PubChem | |
URL | https://pubchem.ncbi.nlm.nih.gov | |
Description | Data deposited in or computed by PubChem | |
InChI Key |
URCBHRLIPUAWTF-UHFFFAOYSA-N | |
Source | PubChem | |
URL | https://pubchem.ncbi.nlm.nih.gov | |
Description | Data deposited in or computed by PubChem | |
Canonical SMILES |
C1C(CC1(C(=O)O)N)CO | |
Source | PubChem | |
URL | https://pubchem.ncbi.nlm.nih.gov | |
Description | Data deposited in or computed by PubChem | |
Molecular Formula |
C6H11NO3 | |
Source | PubChem | |
URL | https://pubchem.ncbi.nlm.nih.gov | |
Description | Data deposited in or computed by PubChem | |
Molecular Weight |
145.16 g/mol | |
Source | PubChem | |
URL | https://pubchem.ncbi.nlm.nih.gov | |
Description | Data deposited in or computed by PubChem | |
Retrosynthesis Analysis
AI-Powered Synthesis Planning: Our tool employs the Template_relevance Pistachio, Template_relevance Bkms_metabolic, Template_relevance Pistachio_ringbreaker, Template_relevance Reaxys, Template_relevance Reaxys_biocatalysis model, leveraging a vast database of chemical reactions to predict feasible synthetic routes.
One-Step Synthesis Focus: Specifically designed for one-step synthesis, it provides concise and direct routes for your target compounds, streamlining the synthesis process.
Accurate Predictions: Utilizing the extensive PISTACHIO, BKMS_METABOLIC, PISTACHIO_RINGBREAKER, REAXYS, REAXYS_BIOCATALYSIS database, our tool offers high-accuracy predictions, reflecting the latest in chemical research and data.
Strategy Settings
Precursor scoring | Relevance Heuristic |
---|---|
Min. plausibility | 0.01 |
Model | Template_relevance |
Template Set | Pistachio/Bkms_metabolic/Pistachio_ringbreaker/Reaxys/Reaxys_biocatalysis |
Top-N result to add to graph | 6 |
Feasible Synthetic Routes
Haftungsausschluss und Informationen zu In-Vitro-Forschungsprodukten
Bitte beachten Sie, dass alle Artikel und Produktinformationen, die auf BenchChem präsentiert werden, ausschließlich zu Informationszwecken bestimmt sind. Die auf BenchChem zum Kauf angebotenen Produkte sind speziell für In-vitro-Studien konzipiert, die außerhalb lebender Organismen durchgeführt werden. In-vitro-Studien, abgeleitet von dem lateinischen Begriff "in Glas", beinhalten Experimente, die in kontrollierten Laborumgebungen unter Verwendung von Zellen oder Geweben durchgeführt werden. Es ist wichtig zu beachten, dass diese Produkte nicht als Arzneimittel oder Medikamente eingestuft sind und keine Zulassung der FDA für die Vorbeugung, Behandlung oder Heilung von medizinischen Zuständen, Beschwerden oder Krankheiten erhalten haben. Wir müssen betonen, dass jede Form der körperlichen Einführung dieser Produkte in Menschen oder Tiere gesetzlich strikt untersagt ist. Es ist unerlässlich, sich an diese Richtlinien zu halten, um die Einhaltung rechtlicher und ethischer Standards in Forschung und Experiment zu gewährleisten.