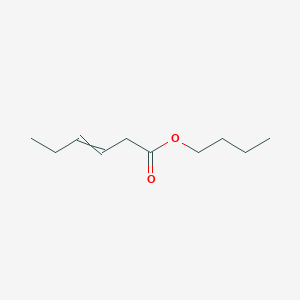
Butylester der (3E)-Hex-3-ensäure
- Klicken Sie auf QUICK INQUIRY, um ein Angebot von unserem Expertenteam zu erhalten.
- Mit qualitativ hochwertigen Produkten zu einem WETTBEWERBSFÄHIGEN Preis können Sie sich mehr auf Ihre Forschung konzentrieren.
Übersicht
Beschreibung
Butyl (3E)-hex-3-enoate is an organic compound belonging to the ester family. Esters are characterized by their pleasant fragrances and are commonly found in essential oils and pheromones. Butyl (3E)-hex-3-enoate is specifically known for its fruity aroma, making it a valuable component in the flavor and fragrance industry.
Wissenschaftliche Forschungsanwendungen
Butyl (3E)-hex-3-enoate has several applications in scientific research:
Chemistry: Used as a model compound in studies of esterification and hydrolysis reactions.
Biology: Investigated for its role in pheromone signaling in insects.
Medicine: Explored for its potential use in drug delivery systems due to its ability to form biodegradable polymers.
Industry: Widely used in the flavor and fragrance industry for its fruity aroma.
Wirkmechanismus
Target of Action
Butyl (3E)-hex-3-enoate, as a derivative of butyl, is a four-carbon alkyl radical or substituent group . The primary targets of butyl compounds can vary depending on the specific compound and its structure.
Mode of Action
For instance, some butyl compounds have been found to inhibit acetyl coenzyme-A carboxylase, a pivotal enzyme in plant fatty acid biosynthesis .
Biochemical Pathways
For example, some butyl compounds have been found to affect the synthesis of fatty acids
Pharmacokinetics
The pharmacokinetics of butyl compounds can be influenced by various factors, including the specific structure of the compound, the route of administration, and the individual’s physiological condition .
Result of Action
For instance, some butyl compounds have been found to inhibit the activity of certain enzymes, leading to changes in cellular metabolism .
Action Environment
The action, efficacy, and stability of Butyl (3E)-hex-3-enoate can be influenced by various environmental factors. For instance, the presence of other chemicals, pH, temperature, and other environmental conditions can affect the action of butyl compounds . .
Biochemische Analyse
Biochemical Properties
It is speculated that it may interact with various enzymes, proteins, and other biomolecules, influencing their function and participating in biochemical reactions .
Cellular Effects
It is possible that it influences cell function, including impacts on cell signaling pathways, gene expression, and cellular metabolism .
Molecular Mechanism
It may exert its effects at the molecular level, including potential binding interactions with biomolecules, enzyme inhibition or activation, and changes in gene expression .
Metabolic Pathways
It could potentially affect metabolic flux or metabolite levels .
Vorbereitungsmethoden
Synthetic Routes and Reaction Conditions: Butyl (3E)-hex-3-enoate can be synthesized through the esterification reaction between butanol and hex-3-enoic acid. The reaction typically requires an acid catalyst, such as sulfuric acid, and is conducted under reflux conditions to drive the reaction to completion. The general reaction is as follows:
C4H9OH+C6H10O2→C10H18O2+H2O
Industrial Production Methods: In industrial settings, the production of butyl (3E)-hex-3-enoate often involves continuous esterification processes. These processes utilize large-scale reactors and efficient separation techniques to maximize yield and purity. The use of catalysts such as p-toluenesulfonic acid or ion-exchange resins can enhance the reaction rate and efficiency.
Types of Reactions:
Oxidation: Butyl (3E)-hex-3-enoate can undergo oxidation reactions, particularly at the double bond, leading to the formation of epoxides or diols.
Reduction: The ester functional group can be reduced to the corresponding alcohol using reducing agents like lithium aluminum hydride.
Substitution: Nucleophilic substitution reactions can occur at the ester carbonyl carbon, leading to the formation of various derivatives.
Common Reagents and Conditions:
Oxidation: Reagents such as peracids (e.g., m-chloroperbenzoic acid) are commonly used for epoxidation.
Reduction: Lithium aluminum hydride or sodium borohydride in anhydrous conditions.
Substitution: Nucleophiles like amines or alcohols under basic or acidic conditions.
Major Products:
Oxidation: Epoxides, diols.
Reduction: Butyl hex-3-en-1-ol.
Substitution: Amides, esters with different alcohol groups.
Vergleich Mit ähnlichen Verbindungen
Butyl acetate: Another ester with a fruity aroma, commonly used as a solvent.
Hexyl acetate: Similar in structure but with a hexyl group instead of a butyl group, also used in flavors and fragrances.
Ethyl (3E)-hex-3-enoate: Similar ester with an ethyl group, used in the flavor industry.
Uniqueness: Butyl (3E)-hex-3-enoate is unique due to its specific combination of a butyl group and a hex-3-enoate moiety, which imparts a distinct aroma profile. Its double bond at the 3-position also allows for unique chemical reactivity compared to saturated esters.
Biologische Aktivität
Butyl (3E)-hex-3-enoate, a fatty acid ester, is recognized for its diverse biological activities and applications in various fields, including chemistry, biology, and medicine. This compound is synthesized through the esterification of butanol and hex-3-enoic acid, resulting in a structure characterized by a double bond at the 3-position of the hexanoate chain. This unique configuration influences its reactivity and biological interactions.
Chemical Structure and Properties
The molecular formula of Butyl (3E)-hex-3-enoate is C10H18O2, with a molecular weight of approximately 170.25 g/mol. The structure can be depicted as follows:
Target of Action
Butyl (3E)-hex-3-enoate has been identified as a potential modulator in biochemical pathways, particularly influencing fatty acid biosynthesis by inhibiting key enzymes like acetyl coenzyme-A carboxylase.
Cellular Effects
Research indicates that this compound may influence cell signaling pathways, gene expression, and overall cellular metabolism. It is speculated to interact with various enzymes, thereby participating in biochemical reactions that could alter metabolic flux or metabolite levels.
Insect Pheromone Signaling
One of the notable biological activities of Butyl (3E)-hex-3-enoate is its role in pheromone signaling among insects. This compound has been studied for its potential to act as an attractant or repellent, influencing insect behavior and ecology.
Medical Applications
In medicinal chemistry, Butyl (3E)-hex-3-enoate has been explored for its potential use in drug delivery systems. Its ability to form biodegradable polymers makes it a candidate for developing targeted drug delivery methods.
Biochemical Studies
A study investigating the effects of Butyl (3E)-hex-3-enoate on enzyme activity revealed significant inhibition of certain metabolic pathways. For instance, the compound was shown to reduce the activity of enzymes involved in fatty acid synthesis, thereby affecting lipid metabolism.
Comparative Analysis with Similar Compounds
Compound Name | Biological Activity | Unique Features |
---|---|---|
Butyl acetate | Solvent in various applications | Fruity aroma; less reactive |
Hexyl acetate | Flavoring agent | Similar structure; longer carbon chain |
Ethyl (3E)-hex-3-enoate | Used in flavor industry | Distinct aroma profile |
Eigenschaften
IUPAC Name |
butyl hex-3-enoate |
Source
|
---|---|---|
Details | Computed by Lexichem TK 2.7.0 (PubChem release 2021.05.07) | |
Source | PubChem | |
URL | https://pubchem.ncbi.nlm.nih.gov | |
Description | Data deposited in or computed by PubChem | |
InChI |
InChI=1S/C10H18O2/c1-3-5-7-8-10(11)12-9-6-4-2/h5,7H,3-4,6,8-9H2,1-2H3 |
Source
|
Details | Computed by InChI 1.0.6 (PubChem release 2021.05.07) | |
Source | PubChem | |
URL | https://pubchem.ncbi.nlm.nih.gov | |
Description | Data deposited in or computed by PubChem | |
InChI Key |
XZKZOAYDHBSAQW-UHFFFAOYSA-N |
Source
|
Details | Computed by InChI 1.0.6 (PubChem release 2021.05.07) | |
Source | PubChem | |
URL | https://pubchem.ncbi.nlm.nih.gov | |
Description | Data deposited in or computed by PubChem | |
Canonical SMILES |
CCCCOC(=O)CC=CCC |
Source
|
Details | Computed by OEChem 2.3.0 (PubChem release 2021.05.07) | |
Source | PubChem | |
URL | https://pubchem.ncbi.nlm.nih.gov | |
Description | Data deposited in or computed by PubChem | |
Molecular Formula |
C10H18O2 |
Source
|
Details | Computed by PubChem 2.1 (PubChem release 2021.05.07) | |
Source | PubChem | |
URL | https://pubchem.ncbi.nlm.nih.gov | |
Description | Data deposited in or computed by PubChem | |
Molecular Weight |
170.25 g/mol |
Source
|
Details | Computed by PubChem 2.1 (PubChem release 2021.05.07) | |
Source | PubChem | |
URL | https://pubchem.ncbi.nlm.nih.gov | |
Description | Data deposited in or computed by PubChem | |
Haftungsausschluss und Informationen zu In-Vitro-Forschungsprodukten
Bitte beachten Sie, dass alle Artikel und Produktinformationen, die auf BenchChem präsentiert werden, ausschließlich zu Informationszwecken bestimmt sind. Die auf BenchChem zum Kauf angebotenen Produkte sind speziell für In-vitro-Studien konzipiert, die außerhalb lebender Organismen durchgeführt werden. In-vitro-Studien, abgeleitet von dem lateinischen Begriff "in Glas", beinhalten Experimente, die in kontrollierten Laborumgebungen unter Verwendung von Zellen oder Geweben durchgeführt werden. Es ist wichtig zu beachten, dass diese Produkte nicht als Arzneimittel oder Medikamente eingestuft sind und keine Zulassung der FDA für die Vorbeugung, Behandlung oder Heilung von medizinischen Zuständen, Beschwerden oder Krankheiten erhalten haben. Wir müssen betonen, dass jede Form der körperlichen Einführung dieser Produkte in Menschen oder Tiere gesetzlich strikt untersagt ist. Es ist unerlässlich, sich an diese Richtlinien zu halten, um die Einhaltung rechtlicher und ethischer Standards in Forschung und Experiment zu gewährleisten.