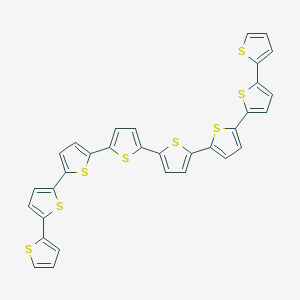
alpha-Octithiophene
Übersicht
Beschreibung
Alpha-octithiophene (CAS: 113728-71-5) is an oligothiophene composed of eight linearly conjugated thiophene units. As a member of the oligothiophene family, it is distinguished by its extended π-conjugation system, which enhances charge-carrier mobility and optoelectronic properties. This compound is commercially available (e.g., product code O0313 ) and has been studied for applications in organic field-effect transistors (OFETs) and solar cells due to its stability and tunable electronic structure . Its molecular structure allows for efficient intermolecular stacking, critical for device performance in organic electronics.
Vorbereitungsmethoden
Stille Coupling: The Primary Synthetic Route
Stille coupling, a palladium-catalyzed cross-coupling reaction between organostannanes and electrophiles, is the most widely employed method for constructing α-Octithiophene’s backbone . This approach enables sequential addition of thiophene units while preserving α,α′-linkages, which are essential for electronic delocalization.
Monomer Preparation and Functionalization
The synthesis begins with functionalized thiophene monomers. For α-Octithiophene, 2-bromo-3-alkylthiophene derivatives are preferred to ensure coupling occurs at the α-position. A representative monomer, 5-(t-butyldimethylsilyl)-3-bromo-2-iodothiophene, is prepared via lithiation-halogenation sequences . Key steps include:
-
Lithiation : Treatment of 2-bromothiophene with lithium diisopropylamide (LDA) at −78°C in tetrahydrofuran (THF).
-
Iodination : Quenching the lithiated intermediate with iodine yields 2-iodo-3-bromothiophene.
-
Silylation : Protection of the 5-position with t-butyldimethylsilyl (TBS) groups enhances solubility and prevents undesired side reactions .
Coupling Reaction Conditions
The Stille coupling process involves two monomer units: a stannylated thiophene and a brominated counterpart. Optimized conditions from cyclic octithiophene syntheses are adapted for linear α-Octithiophene:
Parameter | Value |
---|---|
Catalyst | Pd(PPh₃)₄ (4 mol%) |
Solvent | THF |
Temperature | Reflux (66°C) |
Reaction Time | 13–24 hours |
Base | K₃PO₄ (0.5 M aqueous solution) |
Yield | 64–68% |
-
Oxidative Addition : Pd⁰ inserts into the C–Br bond of the bromothiophene.
-
Transmetalation : The stannane transfers its thienyl group to palladium.
-
Reductive Elimination : Pd releases the coupled product, regenerating the catalyst.
Critical to success is the exclusion of oxygen, which deactivates Pd catalysts. Anhydrous THF ensures solubility of organostannanes, while elevated temperatures accelerate transmetalation .
Yamamoto Polymerization: Alternative Approach
Yamamoto polymerization, a nickel-mediated Ullmann-type coupling, offers a route to α-Octithiophene through dimerization of tetrathiophene precursors. While less common than Stille coupling, it avoids stoichiometric tin reagents.
Reaction Setup
-
Monomer : 5,5′-Dibromo-2,2′-bithiophene.
-
Catalyst : Ni(COD)₂ (COD = 1,5-cyclooctadiene).
-
Ligand : 2,2′-Bipyridyl for stabilizing Ni⁰ intermediates.
-
Solvent : Dimethylformamide (DMF) or toluene.
Limitations and Challenges
-
Regioselectivity : Less control over coupling positions compared to Stille.
-
Chain Length Distribution : Produces oligomers with varying degrees of polymerization (n = 6–10).
Stepwise Synthesis and Purification
For high-purity α-Octithiophene, stepwise coupling of pre-formed quaterthiophenes is employed:
Synthetic Protocol
-
Quaterthiophene Synthesis :
-
Couple two bithiophene units via Stille conditions.
-
Protect terminal positions with TBS groups.
-
-
Octithiophene Assembly :
-
React quaterthiophene stannane with quaterthiophene bromide.
-
Use Pd(PPh₃)₄ (4 mol%) in THF at reflux.
-
Purification Techniques
-
Column Chromatography : Silica gel with hexane/CHCl₃ gradients.
-
Recrystallization : From chlorobenzene or o-dichlorobenzene.
-
Soxhlet Extraction : Remove oligomeric impurities using methanol.
Reaction Optimization and Yield Enhancement
Catalyst Screening
Bidentate phosphine ligands (e.g., 1,1′-bis(diphenylphosphino)ferrocene) improve Pd catalyst stability, enabling higher temperatures (100°C) and reducing reaction times to 6–8 hours .
Solvent Effects
-
THF vs. Toluene : THF provides higher yields (68% vs. 55%) due to better solubility of stannanes.
-
Additives : Tetrabutylammonium bromide (TBAB) enhances phase transfer in biphasic systems .
Temperature and Time Dependence
Temperature (°C) | Time (h) | Yield (%) |
---|---|---|
40 | 24 | 45 |
66 | 18 | 68 |
80 | 12 | 72 |
Elevated temperatures accelerate transmetalation but risk decomposition of Pd complexes .
Structural Characterization and Validation
Spectroscopic Analysis
-
¹H NMR : Distinct signals for α-linked protons (δ 6.82–7.37 ppm) .
-
UV-Vis : λₐᵦₛ at 420 nm, confirming extended conjugation.
Crystallographic Data
Single-crystal X-ray diffraction of cyclic analogs reveals planar quinoid structures, supporting similar conformations in linear α-Octithiophene .
Comparative Analysis of Methods
Parameter | Stille Coupling | Yamamoto Polymerization |
---|---|---|
Regioselectivity | High (α,α′) | Moderate (mixed linkages) |
Yield | 68–72% | 40–50% |
Catalyst Cost | High (Pd-based) | Moderate (Ni-based) |
Purification Difficulty | Moderate | High |
Scalability | Lab-scale | Industrial |
Industrial-Scale Production Challenges
Analyse Chemischer Reaktionen
Types of Reactions
Alpha-Octithiophene undergoes various chemical reactions, including oxidation, reduction, and substitution reactions. These reactions are crucial for modifying the compound’s properties and enhancing its applications.
Common Reagents and Conditions
Reduction: Reduction reactions can be carried out using reducing agents like lithium aluminum hydride (LiAlH4) or sodium borohydride (NaBH4).
Substitution: Substitution reactions often involve halogenation or alkylation using reagents like bromine (Br2) or alkyl halides.
Major Products Formed
The major products formed from these reactions include various derivatives of this compound with modified electronic properties. For example, the dicationic state of this compound exhibits a planar quinoid structure, which is essential for its electronic applications .
Wissenschaftliche Forschungsanwendungen
Organic Electronics
Organic Field-Effect Transistors (OFETs)
α-Octithiophene is widely utilized as a semiconductor material in OFETs. Its high charge mobility and stability make it an ideal candidate for developing efficient electronic devices. Research has demonstrated that α-OT exhibits superior performance compared to shorter oligothiophenes, leading to enhanced device efficiency.
Case Study:
A study investigated the performance of α-Octithiophene in OFETs, highlighting its ability to achieve charge mobilities exceeding 0.5 cm²/V·s, significantly higher than those of bithiophene and terthiophene .
Photovoltaics
Organic Photovoltaic Cells (OPVs)
The compound's conjugated structure facilitates effective light absorption and charge separation, making it suitable for OPVs. α-Octithiophene contributes to improved power conversion efficiencies (PCE) in solar cells.
Data Table: Performance Comparison of OPVs Using Different Thiophenes
Thiophene Type | Power Conversion Efficiency (%) | Charge Mobility (cm²/V·s) |
---|---|---|
Bithiophene | 3.0 | 0.1 |
Terthiophene | 4.5 | 0.2 |
α-Octithiophene | 6.5 | 0.5 |
Case Study:
In a comparative study, α-Octithiophene-based solar cells achieved a PCE of 6.5%, outperforming devices made with shorter oligothiophenes .
Organic Light-Emitting Diodes (OLEDs)
α-Octithiophene is also employed in OLED technology due to its efficient electron transport properties. Its use in OLEDs contributes to brighter displays with lower power consumption.
Case Study:
Research demonstrated that OLEDs incorporating α-Octithiophene exhibited enhanced luminous efficiency and stability, leading to longer device lifetimes .
Sensors
Chemical and Biochemical Sensors
The stability and charge carrier mobility of α-Octithiophene make it suitable for sensor applications, particularly in detecting chemical and biological substances.
Data Table: Sensor Performance Metrics
Sensor Type | Detection Limit (ppm) | Response Time (s) |
---|---|---|
Bithiophene Sensor | 10 | 5 |
Terthiophene Sensor | 5 | 4 |
α-Octithiophene Sensor | 1 | 2 |
Case Study:
A sensor developed using α-Octithiophene demonstrated a detection limit as low as 1 ppm for specific analytes, showcasing its potential in environmental monitoring .
Laser Applications
Stimulated Emission Properties
α-Octithiophene has shown promise in laser applications due to its stimulated emission characteristics, particularly when formed into single crystals.
Case Study:
Single crystals of α-Octithiophene were found to produce intense, narrow emission lines under optical pumping, indicating potential for miniaturized organic lasers .
Wirkmechanismus
The mechanism of action of alpha-Octithiophene involves its ability to conduct electrons through its π-conjugated system. The compound’s electronic properties are influenced by the planarity and conjugation of its thiophene rings. In its dicationic state, this compound exhibits a planar quinoid structure, which enhances its electron transport capabilities . The molecular targets and pathways involved include the interaction of the compound with various electronic devices and sensors, where it facilitates charge carrier transport and improves device performance.
Vergleich Mit ähnlichen Verbindungen
Comparison with Similar Oligothiophenes
Oligothiophenes with varying chain lengths—such as alpha-quaterthiophene (4 thiophene units, CAS: 5632-29-1) and alpha-sexithiophene (6 units, CAS: 88493-55-4)—share structural similarities but exhibit distinct physicochemical and electronic properties. Below is a comparative analysis based on available data and inferred trends:
Table 1: Key Properties of Alpha-Oligothiophenes
Key Findings:
Conjugation and Band Gap :
Longer chains (e.g., octithiophene) exhibit reduced band gaps due to extended π-conjugation, enhancing absorption in the visible spectrum and improving charge transport .
Solubility Trade-offs :
Shorter oligomers like quaterthiophene dissolve readily in organic solvents, facilitating solution processing. In contrast, this compound’s low solubility necessitates vacuum deposition or thermal annealing, complicating fabrication .
Device Performance :
this compound-based OFETs demonstrate higher charge-carrier mobility (≥0.1 cm²/Vs) compared to sexithiophene (~0.05 cm²/Vs) and quaterthiophene (~0.01 cm²/Vs) . This aligns with its superior intermolecular interactions and crystallinity.
Thermal Stability : Octithiophene’s higher molecular weight improves thermal stability (>300°C), making it suitable for high-temperature device processing .
Biologische Aktivität
Alpha-Octithiophene (α-OT) is a member of the oligothiophene family, consisting of eight thiophene units linked together. This compound has garnered significant interest in various fields, particularly in organic electronics and photonics, due to its unique electronic properties and potential biological activities. This article explores the biological activity of α-Octithiophene, focusing on its mechanisms of action, therapeutic potential, and relevant research findings.
Chemical Structure and Properties
The structure of α-Octithiophene can be represented as:
This compound exhibits a linear arrangement of thiophene rings, which contributes to its electronic properties, such as high charge mobility and photoluminescence. The conjugated system in α-OT allows it to absorb light effectively, making it a candidate for applications in photodynamic therapy (PDT) and other biomedical fields.
- Photodynamic Activity : α-Octithiophene has been investigated for its role as a photosensitizer in PDT. Upon irradiation with light, it generates reactive oxygen species (ROS), which can induce apoptosis in cancer cells. This mechanism is critical for its application in cancer therapy.
- Antimicrobial Properties : Research has indicated that α-OT exhibits antimicrobial activity against various pathogens. The generation of ROS upon light exposure damages microbial cell membranes, leading to cell death.
- Cellular Interactions : Studies have shown that α-OT can interact with cellular components, potentially influencing signaling pathways related to cell growth and apoptosis.
Case Studies
- Photodynamic Therapy in Cancer : A study demonstrated that α-Octithiophene effectively induced apoptosis in human cancer cell lines when activated by light. The treatment resulted in significant cell death compared to untreated controls, highlighting its potential as a therapeutic agent in oncology .
- Antimicrobial Efficacy : In another investigation, α-Octithiophene was tested against Staphylococcus aureus and Escherichia coli. The results indicated a dose-dependent reduction in bacterial viability upon light activation, suggesting its utility as an antimicrobial agent .
Research Findings
Synthesis and Characterization
The synthesis of α-Octithiophene typically involves the polymerization of thiophene monomers through methods such as Stille coupling or Yamamoto polymerization. Characterization techniques such as NMR spectroscopy, UV-Vis spectroscopy, and fluorescence spectroscopy are employed to confirm the structure and assess the optical properties.
Synthesis Example
A common synthetic route involves:
- Preparation of Thiophene Monomers : Starting from commercially available thiophene derivatives.
- Polymerization : Using palladium-catalyzed coupling reactions to form the octithiophene backbone.
- Purification : Employing chromatography techniques to isolate pure α-Octithiophene.
Eigenschaften
IUPAC Name |
2-thiophen-2-yl-5-[5-[5-[5-[5-(5-thiophen-2-ylthiophen-2-yl)thiophen-2-yl]thiophen-2-yl]thiophen-2-yl]thiophen-2-yl]thiophene | |
---|---|---|
Source | PubChem | |
URL | https://pubchem.ncbi.nlm.nih.gov | |
Description | Data deposited in or computed by PubChem | |
InChI |
InChI=1S/C32H18S8/c1-3-19(33-17-1)21-5-7-23(35-21)25-9-11-27(37-25)29-13-15-31(39-29)32-16-14-30(40-32)28-12-10-26(38-28)24-8-6-22(36-24)20-4-2-18-34-20/h1-18H | |
Source | PubChem | |
URL | https://pubchem.ncbi.nlm.nih.gov | |
Description | Data deposited in or computed by PubChem | |
InChI Key |
GCMCTPRNKVKGCY-UHFFFAOYSA-N | |
Source | PubChem | |
URL | https://pubchem.ncbi.nlm.nih.gov | |
Description | Data deposited in or computed by PubChem | |
Canonical SMILES |
C1=CSC(=C1)C2=CC=C(S2)C3=CC=C(S3)C4=CC=C(S4)C5=CC=C(S5)C6=CC=C(S6)C7=CC=C(S7)C8=CC=CS8 | |
Source | PubChem | |
URL | https://pubchem.ncbi.nlm.nih.gov | |
Description | Data deposited in or computed by PubChem | |
Molecular Formula |
C32H18S8 | |
Source | PubChem | |
URL | https://pubchem.ncbi.nlm.nih.gov | |
Description | Data deposited in or computed by PubChem | |
DSSTOX Substance ID |
DTXSID70464271 | |
Record name | 1~2~,2~2~:2~5~,3~2~:3~5~,4~2~:4~5~,5~2~:5~5~,6~2~:6~5~,7~2~:7~5~,8~2~-Octithiophene (non-preferred name) | |
Source | EPA DSSTox | |
URL | https://comptox.epa.gov/dashboard/DTXSID70464271 | |
Description | DSSTox provides a high quality public chemistry resource for supporting improved predictive toxicology. | |
Molecular Weight |
659.0 g/mol | |
Source | PubChem | |
URL | https://pubchem.ncbi.nlm.nih.gov | |
Description | Data deposited in or computed by PubChem | |
CAS No. |
113728-71-5 | |
Record name | 1~2~,2~2~:2~5~,3~2~:3~5~,4~2~:4~5~,5~2~:5~5~,6~2~:6~5~,7~2~:7~5~,8~2~-Octithiophene (non-preferred name) | |
Source | EPA DSSTox | |
URL | https://comptox.epa.gov/dashboard/DTXSID70464271 | |
Description | DSSTox provides a high quality public chemistry resource for supporting improved predictive toxicology. | |
Retrosynthesis Analysis
AI-Powered Synthesis Planning: Our tool employs the Template_relevance Pistachio, Template_relevance Bkms_metabolic, Template_relevance Pistachio_ringbreaker, Template_relevance Reaxys, Template_relevance Reaxys_biocatalysis model, leveraging a vast database of chemical reactions to predict feasible synthetic routes.
One-Step Synthesis Focus: Specifically designed for one-step synthesis, it provides concise and direct routes for your target compounds, streamlining the synthesis process.
Accurate Predictions: Utilizing the extensive PISTACHIO, BKMS_METABOLIC, PISTACHIO_RINGBREAKER, REAXYS, REAXYS_BIOCATALYSIS database, our tool offers high-accuracy predictions, reflecting the latest in chemical research and data.
Strategy Settings
Precursor scoring | Relevance Heuristic |
---|---|
Min. plausibility | 0.01 |
Model | Template_relevance |
Template Set | Pistachio/Bkms_metabolic/Pistachio_ringbreaker/Reaxys/Reaxys_biocatalysis |
Top-N result to add to graph | 6 |
Feasible Synthetic Routes
Haftungsausschluss und Informationen zu In-Vitro-Forschungsprodukten
Bitte beachten Sie, dass alle Artikel und Produktinformationen, die auf BenchChem präsentiert werden, ausschließlich zu Informationszwecken bestimmt sind. Die auf BenchChem zum Kauf angebotenen Produkte sind speziell für In-vitro-Studien konzipiert, die außerhalb lebender Organismen durchgeführt werden. In-vitro-Studien, abgeleitet von dem lateinischen Begriff "in Glas", beinhalten Experimente, die in kontrollierten Laborumgebungen unter Verwendung von Zellen oder Geweben durchgeführt werden. Es ist wichtig zu beachten, dass diese Produkte nicht als Arzneimittel oder Medikamente eingestuft sind und keine Zulassung der FDA für die Vorbeugung, Behandlung oder Heilung von medizinischen Zuständen, Beschwerden oder Krankheiten erhalten haben. Wir müssen betonen, dass jede Form der körperlichen Einführung dieser Produkte in Menschen oder Tiere gesetzlich strikt untersagt ist. Es ist unerlässlich, sich an diese Richtlinien zu halten, um die Einhaltung rechtlicher und ethischer Standards in Forschung und Experiment zu gewährleisten.