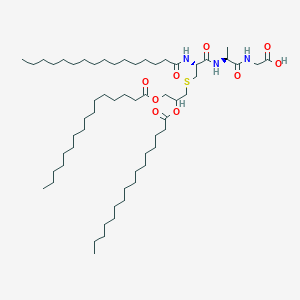
Pam3-Cys-Ala-Gly
Übersicht
Beschreibung
S-(2,3-Bis(palmitoyloxy)propyl)-N-palmitoylcysteinyl-alanyl-glycine is a synthetic lipopeptide known for its immunostimulatory properties. This compound is a derivative of bacterial lipoproteins and is often used in scientific research due to its ability to activate immune responses.
Wissenschaftliche Forschungsanwendungen
Immunotherapy Applications
Cancer Immunotherapy
- Recent studies indicate that Pam3-Cys-Ala-Gly can enhance anti-tumor immune responses. It has been shown to activate various immune cells that can target and destroy cancer cells. For instance, research has demonstrated its potential in inducing cytotoxic T lymphocyte (CTL) responses against tumors .
Allergy Treatment
- This compound is being explored as an adjuvant in allergen immunotherapy. Its ability to modulate immune responses can potentially shift the balance from Th2 (associated with allergic reactions) to Th1 responses, thereby reducing allergy symptoms. Studies have indicated that it may enhance the efficacy of allergen-specific immunotherapy by promoting a more balanced immune response .
Vaccine Development
This compound serves as an effective adjuvant in vaccine formulations. Its ability to enhance the immunogenicity of vaccine candidates has been documented in various studies:
- Peptide Vaccines : When conjugated with specific peptide antigens, this compound has been shown to improve antibody responses and T cell activation in animal models .
- Mucosal Vaccination : Research indicates that mucosal administration of vaccines containing this compound can induce robust local and systemic immune responses, making it a candidate for developing effective mucosal vaccines against pathogens such as influenza .
Case Studies and Research Findings
Comparative Analysis with Other Compounds
This compound can be compared with other lipopeptides based on their structure and immunological properties:
Compound Name | Structure Characteristics | Unique Features |
---|---|---|
Pam2-Cys | Two palmitic acid groups linked to cysteine | Less potent than this compound in TLR activation |
Pam3CSK4 | Triacylated with an additional lysine residue | Enhanced solubility and immunogenicity |
Lipopolysaccharides | Complex polysaccharides with lipid components | Stronger innate immune response but different mechanism |
This compound stands out due to its specific combination of triacylation and peptide sequence, which optimally activates TLR2 compared to other lipopeptides .
Wirkmechanismus
Target of Action
Pam3-Cys-Ala-Gly, also known as 2-[[(2S)-2-[[(2R)-3-[2,3-di(hexadecanoyloxy)propylsulfanyl]-2-(hexadecanoylamino)propanoyl]amino]propanoyl]amino]acetic acid or S-(2,3-Bis(palmitoyloxy)propyl)-N-palmitoylcysteinyl-alanyl-glycine, is a synthetic bacterial lipopeptide . It primarily targets macrophages and B cells , acting as a potent activator .
Mode of Action
The compound interacts with Toll-like receptor 2 (TLR2) to induce an antimicrobial pathway . This interaction triggers the upregulation of the vitamin D receptor and the induction of the antimicrobial peptide cathelicidin, ultimately resulting in the killing of intracellular Mycobacterium tuberculosis .
Biochemical Pathways
The activation of TLR2 by this compound leads to the induction of an antimicrobial pathway. This pathway involves the upregulation of the vitamin D receptor and the induction of the antimicrobial peptide cathelicidin . The downstream effects include the activation of macrophages and B cells, leading to an enhanced immune response .
Pharmacokinetics
It’s known that macrophages efficiently take up this compound
Result of Action
The result of this compound’s action is the activation of macrophages and B cells, leading to an enhanced immune response . In vitro studies with human macrophages have shown that this compound triggers the release of significantly higher levels of TNF, IL-12, and IL-10 than controls .
Action Environment
The action of this compound can be influenced by various environmental factors. For instance, the formulation and delivery of the compound can affect its efficacy
Biochemische Analyse
Biochemical Properties
Pam3-Cys-Ala-Gly plays a significant role in biochemical reactions. It interacts with Toll-like receptor 2, inducing an antimicrobial pathway . This interaction triggers the activation of macrophages and B cells, which are crucial components of the immune system .
Cellular Effects
The effects of this compound on cells are profound. It influences cell function by modulating cell signaling pathways and gene expression. Specifically, it activates macrophages and B cells, enhancing their ability to respond to microbial threats .
Molecular Mechanism
The molecular mechanism of action of this compound involves binding interactions with biomolecules, particularly Toll-like receptor 2 . This binding triggers a cascade of events leading to the activation of macrophages and B cells .
Metabolic Pathways
This compound is involved in several metabolic pathways, primarily through its interaction with Toll-like receptor 2
Vorbereitungsmethoden
Synthetic Routes and Reaction Conditions
The synthesis of S-(2,3-Bis(palmitoyloxy)propyl)-N-palmitoylcysteinyl-alanyl-glycine involves multiple steps. The process typically starts with the preparation of the lipid moiety, 2,3-bis(palmitoyloxy)propyl, which is then conjugated to the peptide sequence. The peptide is synthesized using solid-phase peptide synthesis (SPPS), where amino acids are sequentially added to a growing chain anchored to a solid resin. The lipid moiety is then attached to the peptide through a thioether bond formed between the cysteine residue and the lipid .
Industrial Production Methods
Industrial production of this compound follows similar synthetic routes but on a larger scale. The process involves optimization of reaction conditions to ensure high yield and purity. Techniques such as high-performance liquid chromatography (HPLC) are used for purification .
Analyse Chemischer Reaktionen
Types of Reactions
S-(2,3-Bis(palmitoyloxy)propyl)-N-palmitoylcysteinyl-alanyl-glycine undergoes various chemical reactions, including:
Oxidation: The thiol group in the cysteine residue can be oxidized to form disulfides.
Reduction: Disulfides can be reduced back to thiols.
Substitution: The lipid moiety can be substituted with other fatty acids to modify the compound’s properties.
Common Reagents and Conditions
Common reagents used in these reactions include oxidizing agents like hydrogen peroxide for oxidation and reducing agents like dithiothreitol for reduction. Substitution reactions often require catalysts and specific reaction conditions to ensure selective modification .
Major Products Formed
The major products formed from these reactions include disulfide-linked dimers, reduced thiol forms, and lipid-modified derivatives .
Vergleich Mit ähnlichen Verbindungen
Similar Compounds
Pam3CSK4: Another synthetic lipopeptide with similar immunostimulatory properties.
FSL-1: A lipopeptide that also activates TLR2 but has a different lipid moiety.
MALP-2: A natural lipopeptide derived from Mycoplasma that activates TLR2 and TLR6.
Uniqueness
S-(2,3-Bis(palmitoyloxy)propyl)-N-palmitoylcysteinyl-alanyl-glycine is unique due to its specific lipid and peptide structure, which provides a balanced activation of immune responses with minimal side effects. Its synthetic nature allows for precise modifications to tailor its properties for specific research and therapeutic applications .
Biologische Aktivität
Pam3-Cys-Ala-Gly (Pam3Cys) is a synthetic lipopeptide that serves as a potent agonist for Toll-like receptor 2 (TLR2). This compound is recognized for its significant immunostimulatory effects, particularly in the context of immune modulation and therapeutic applications such as cancer immunotherapy and allergy treatments. This article examines the biological activity of Pam3Cys, highlighting its mechanisms of action, effects on various immune cells, and potential applications in clinical settings.
Pam3Cys consists of a triacylated lipid moiety linked to a peptide sequence composed of cysteine, alanine, and glycine. The lipid component is essential for its immunostimulatory properties, allowing it to mimic bacterial components and activate immune responses. It primarily engages TLR2, often forming heterodimers with TLR1 or TLR6, which is crucial for its biological activity.
Table 1: Comparison of TLR Agonists
Compound Name | Structure Characteristics | Unique Features |
---|---|---|
Pam3Cys | Triacylated lipid linked to Cys-Ala-Gly | Potent TLR2 agonist; induces Th2 responses |
Pam2Cys | Two palmitic acid groups linked to cysteine | Less potent than Pam3Cys in TLR activation |
Pam3CSK4 | Triacylated with an additional lysine residue | Enhanced solubility and immunogenicity |
Lipopolysaccharides | Complex polysaccharides with lipid components | Stronger innate immune response but different mechanism |
Immunological Effects
Pam3Cys has been shown to activate various immune cells, including macrophages, dendritic cells (DCs), and natural killer (NK) cells. This activation results in the production of inflammatory cytokines and chemokines, which play critical roles in orchestrating immune responses.
Cytokine Production
Studies indicate that Pam3Cys can induce the production of several key cytokines:
- IL-1 : Involved in the inflammatory response.
- IL-6 : Plays a role in B cell differentiation.
- TNF-α : Critical for systemic inflammation.
- IL-12 : Promotes Th1 responses.
In murine models, co-administration of Pam3Cys with allergens has been shown to potentiate Th2 responses by increasing IL-5 and IL-13 production from naïve CD4+ T cells. This suggests its potential role in allergy therapies as an adjuvant .
Case Studies and Research Findings
-
Cancer Immunotherapy :
Recent research has highlighted the potential of Pam3Cys in cancer immunotherapy. It activates anti-tumor immune responses by stimulating various immune cells, leading to enhanced tumor rejection in preclinical models. For instance, studies have demonstrated that Pam3Cys can significantly increase the efficacy of cancer vaccines by promoting robust T cell responses. -
Allergen Immunotherapy :
In studies involving murine models of asthma induced by ovalbumin (OVA), Pam3Cys was found to enhance Th2 polarization. This effect was characterized by increased levels of IL-4 and IL-13, suggesting its utility as an adjuvant in allergen immunotherapy . -
Mechanistic Studies :
Research utilizing enzyme-linked immunosorbent assays (ELISA) and quantitative polymerase chain reaction (qPCR) has shown that Pam3Cys activates mitogen-activated protein kinase (MAPK) pathways, specifically ERK1/2 and p38 MAPK. These pathways are crucial for mediating pro-inflammatory gene expression .
Eigenschaften
IUPAC Name |
2-[[(2S)-2-[[(2R)-3-[2,3-di(hexadecanoyloxy)propylsulfanyl]-2-(hexadecanoylamino)propanoyl]amino]propanoyl]amino]acetic acid | |
---|---|---|
Source | PubChem | |
URL | https://pubchem.ncbi.nlm.nih.gov | |
Description | Data deposited in or computed by PubChem | |
InChI |
InChI=1S/C59H111N3O9S/c1-5-8-11-14-17-20-23-26-29-32-35-38-41-44-54(63)62-53(59(69)61-51(4)58(68)60-47-55(64)65)50-72-49-52(71-57(67)46-43-40-37-34-31-28-25-22-19-16-13-10-7-3)48-70-56(66)45-42-39-36-33-30-27-24-21-18-15-12-9-6-2/h51-53H,5-50H2,1-4H3,(H,60,68)(H,61,69)(H,62,63)(H,64,65)/t51-,52?,53-/m0/s1 | |
Source | PubChem | |
URL | https://pubchem.ncbi.nlm.nih.gov | |
Description | Data deposited in or computed by PubChem | |
InChI Key |
MELKZHAKCJIPSL-JYHYCRSOSA-N | |
Source | PubChem | |
URL | https://pubchem.ncbi.nlm.nih.gov | |
Description | Data deposited in or computed by PubChem | |
Canonical SMILES |
CCCCCCCCCCCCCCCC(=O)NC(CSCC(COC(=O)CCCCCCCCCCCCCCC)OC(=O)CCCCCCCCCCCCCCC)C(=O)NC(C)C(=O)NCC(=O)O | |
Source | PubChem | |
URL | https://pubchem.ncbi.nlm.nih.gov | |
Description | Data deposited in or computed by PubChem | |
Isomeric SMILES |
CCCCCCCCCCCCCCCC(=O)N[C@@H](CSCC(COC(=O)CCCCCCCCCCCCCCC)OC(=O)CCCCCCCCCCCCCCC)C(=O)N[C@@H](C)C(=O)NCC(=O)O | |
Source | PubChem | |
URL | https://pubchem.ncbi.nlm.nih.gov | |
Description | Data deposited in or computed by PubChem | |
Molecular Formula |
C59H111N3O9S | |
Source | PubChem | |
URL | https://pubchem.ncbi.nlm.nih.gov | |
Description | Data deposited in or computed by PubChem | |
DSSTOX Substance ID |
DTXSID50922566 | |
Record name | N-{2-[(3-{[2,3-Bis(hexadecanoyloxy)propyl]sulfanyl}-1-hydroxy-2-[(1-hydroxyhexadecylidene)amino]propylidene)amino]-1-hydroxypropylidene}glycine | |
Source | EPA DSSTox | |
URL | https://comptox.epa.gov/dashboard/DTXSID50922566 | |
Description | DSSTox provides a high quality public chemistry resource for supporting improved predictive toxicology. | |
Molecular Weight |
1038.6 g/mol | |
Source | PubChem | |
URL | https://pubchem.ncbi.nlm.nih.gov | |
Description | Data deposited in or computed by PubChem | |
CAS No. |
117858-54-5 | |
Record name | N-Palmitoyl-5,6-dipalmitoylcysteinyl-alanyl-glycine | |
Source | ChemIDplus | |
URL | https://pubchem.ncbi.nlm.nih.gov/substance/?source=chemidplus&sourceid=0117858545 | |
Description | ChemIDplus is a free, web search system that provides access to the structure and nomenclature authority files used for the identification of chemical substances cited in National Library of Medicine (NLM) databases, including the TOXNET system. | |
Record name | N-{2-[(3-{[2,3-Bis(hexadecanoyloxy)propyl]sulfanyl}-1-hydroxy-2-[(1-hydroxyhexadecylidene)amino]propylidene)amino]-1-hydroxypropylidene}glycine | |
Source | EPA DSSTox | |
URL | https://comptox.epa.gov/dashboard/DTXSID50922566 | |
Description | DSSTox provides a high quality public chemistry resource for supporting improved predictive toxicology. | |
Haftungsausschluss und Informationen zu In-Vitro-Forschungsprodukten
Bitte beachten Sie, dass alle Artikel und Produktinformationen, die auf BenchChem präsentiert werden, ausschließlich zu Informationszwecken bestimmt sind. Die auf BenchChem zum Kauf angebotenen Produkte sind speziell für In-vitro-Studien konzipiert, die außerhalb lebender Organismen durchgeführt werden. In-vitro-Studien, abgeleitet von dem lateinischen Begriff "in Glas", beinhalten Experimente, die in kontrollierten Laborumgebungen unter Verwendung von Zellen oder Geweben durchgeführt werden. Es ist wichtig zu beachten, dass diese Produkte nicht als Arzneimittel oder Medikamente eingestuft sind und keine Zulassung der FDA für die Vorbeugung, Behandlung oder Heilung von medizinischen Zuständen, Beschwerden oder Krankheiten erhalten haben. Wir müssen betonen, dass jede Form der körperlichen Einführung dieser Produkte in Menschen oder Tiere gesetzlich strikt untersagt ist. Es ist unerlässlich, sich an diese Richtlinien zu halten, um die Einhaltung rechtlicher und ethischer Standards in Forschung und Experiment zu gewährleisten.