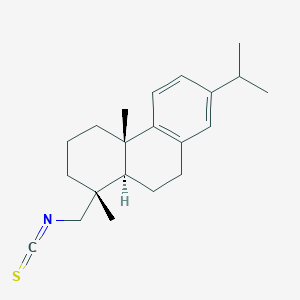
Dehydroabietyl isothiocyanate
Übersicht
Beschreibung
Dehydroabietyl isothiocyanate is a compound with the molecular formula C21H29NS . It is also known by other names such as (1R,4aS,10aR)-1-(isothiocyanatomethyl)-1,4a-dimethyl-7-propan-2-yl-2,3,4,9,10,10a-hexahydrophenanthrene . The molecular weight of this compound is 327.5 g/mol .
Molecular Structure Analysis
The molecular structure of Dehydroabietyl isothiocyanate includes two Zn2+ ions coordinating water/hydroxide and a flexible cap that is implicated in substrate recognition and covers the active site . The InChI string representation of the molecule isInChI=1S/C21H29NS/c1-15(2)16-6-8-18-17(12-16)7-9-19-20(3,13-22-14-23)10-5-11-21(18,19)4/h6,8,12,15,19H,5,7,9-11,13H2,1-4H3/t19-,20-,21+/m0/s1
. Chemical Reactions Analysis
Isothiocyanates, including Dehydroabietyl isothiocyanate, are known to have antimicrobial properties against a variety of pathogens . They are produced via enzymatic hydrolysis of glucosinolates, a class of sulfur-containing secondary metabolites occurring exclusively in the botanical order Brassicales .Physical And Chemical Properties Analysis
The physical and chemical properties of Dehydroabietyl isothiocyanate include a molecular weight of 327.5 g/mol, a XLogP3-AA value of 7.7, a hydrogen bond donor count of 0, a hydrogen bond acceptor count of 2, and a rotatable bond count of 3 . The exact mass of the molecule is 327.20207110 g/mol .Wissenschaftliche Forschungsanwendungen
Antimicrobial Properties
Isothiocyanates, including Dehydroabietyl isothiocyanate, exhibit various biological characteristics, including antimicrobial properties . They can be used to combat a variety of bacterial and fungal infections .
Anti-inflammatory Properties
Isothiocyanates also have anti-inflammatory properties . They can help reduce inflammation in the body, which is beneficial for conditions like arthritis, asthma, and inflammatory bowel disease .
Anticancer Properties
One of the most significant applications of isothiocyanates is their anticancer properties . They have been shown to inhibit the growth of various types of cancer cells .
Antioxidative Properties
Isothiocyanates have antioxidative properties . They can help protect the body from damage caused by harmful free radicals .
Synthetic Chemistry
Isothiocyanates serve as valuable platforms for versatile transformations in synthetic chemistry . They are used as starting materials in various chemical reactions .
Food Preservation
Isothiocyanates have applications in food preservation . They can be used as additives in food products like fruit juices and mayonnaise to extend their shelf life .
Wirkmechanismus
Target of Action
Dehydroabietyl isothiocyanate (ABITC) is a novel phytochemical derived from pine tree bark . Isothiocyanates (ITCs), including ABITC, govern many intracellular targets, including cytochrome P 450 (CYP) enzymes and proteins involved in antioxidant response, tumorigenesis, apoptosis, cell cycle, and metastasis .
Mode of Action
ABITC interacts with its targets, leading to changes in cellular processes. For instance, it can regulate transcription factors and signaling pathways, affecting cell cycle progression and apoptosis . In prostate cancer cells, ABITC has been shown to downregulate mRNA and protein levels of full-length androgen receptor (AR) as well as its splice variants .
Biochemical Pathways
ABITC affects several biochemical pathways. It can inhibit CYP enzymes, induce phase II enzymes via activation of NF-E2-related factor-2 (Nrf2), modulate cell cycle regulators, induce apoptosis, inhibit nuclear factor kappa B (NF-ĸB), inhibit macrophage migration inhibitory factor (MIF), inhibit microtubule polymerization, and inhibit metastasis .
Pharmacokinetics
Itcs are generally metabolized by the mercapturic acid pathway, which includes conjugation of itcs with glutathione (gsh) followed by enzymatic degradation and n-acetylation .
Result of Action
ABITC has demonstrated cytotoxic effects on endometrial cancer (EC) cell lines. It displays dose-dependent and selective cytotoxicity to EC cell lines, leading to apoptosis . In prostate cancer cells, ABITC treatment resulted in growth inhibition and apoptosis induction .
Action Environment
The action of ABITC can be influenced by environmental factors. For instance, the pH and presence of certain cofactors can affect the formation of ITCs . Additionally, the temperature can influence the antioxidant activity of ITCs .
Eigenschaften
IUPAC Name |
(1R,4aS,10aR)-1-(isothiocyanatomethyl)-1,4a-dimethyl-7-propan-2-yl-2,3,4,9,10,10a-hexahydrophenanthrene | |
---|---|---|
Source | PubChem | |
URL | https://pubchem.ncbi.nlm.nih.gov | |
Description | Data deposited in or computed by PubChem | |
InChI |
InChI=1S/C21H29NS/c1-15(2)16-6-8-18-17(12-16)7-9-19-20(3,13-22-14-23)10-5-11-21(18,19)4/h6,8,12,15,19H,5,7,9-11,13H2,1-4H3/t19-,20-,21+/m0/s1 | |
Source | PubChem | |
URL | https://pubchem.ncbi.nlm.nih.gov | |
Description | Data deposited in or computed by PubChem | |
InChI Key |
AKTYQDWLPBOJTD-PCCBWWKXSA-N | |
Source | PubChem | |
URL | https://pubchem.ncbi.nlm.nih.gov | |
Description | Data deposited in or computed by PubChem | |
Canonical SMILES |
CC(C)C1=CC2=C(C=C1)C3(CCCC(C3CC2)(C)CN=C=S)C | |
Source | PubChem | |
URL | https://pubchem.ncbi.nlm.nih.gov | |
Description | Data deposited in or computed by PubChem | |
Isomeric SMILES |
CC(C)C1=CC2=C(C=C1)[C@]3(CCC[C@@]([C@@H]3CC2)(C)CN=C=S)C | |
Source | PubChem | |
URL | https://pubchem.ncbi.nlm.nih.gov | |
Description | Data deposited in or computed by PubChem | |
Molecular Formula |
C21H29NS | |
Source | PubChem | |
URL | https://pubchem.ncbi.nlm.nih.gov | |
Description | Data deposited in or computed by PubChem | |
DSSTOX Substance ID |
DTXSID60921666 | |
Record name | 18-Isothiocyanatoabieta-8,11,13-triene | |
Source | EPA DSSTox | |
URL | https://comptox.epa.gov/dashboard/DTXSID60921666 | |
Description | DSSTox provides a high quality public chemistry resource for supporting improved predictive toxicology. | |
Molecular Weight |
327.5 g/mol | |
Source | PubChem | |
URL | https://pubchem.ncbi.nlm.nih.gov | |
Description | Data deposited in or computed by PubChem | |
Product Name |
Dehydroabietyl isothiocyanate | |
CAS RN |
115269-93-7 | |
Record name | 18-Isothiocyanatoabieta-8,11,13-triene | |
Source | EPA DSSTox | |
URL | https://comptox.epa.gov/dashboard/DTXSID60921666 | |
Description | DSSTox provides a high quality public chemistry resource for supporting improved predictive toxicology. | |
Record name | 115269-93-7 | |
Source | European Chemicals Agency (ECHA) | |
URL | https://echa.europa.eu/information-on-chemicals | |
Description | The European Chemicals Agency (ECHA) is an agency of the European Union which is the driving force among regulatory authorities in implementing the EU's groundbreaking chemicals legislation for the benefit of human health and the environment as well as for innovation and competitiveness. | |
Explanation | Use of the information, documents and data from the ECHA website is subject to the terms and conditions of this Legal Notice, and subject to other binding limitations provided for under applicable law, the information, documents and data made available on the ECHA website may be reproduced, distributed and/or used, totally or in part, for non-commercial purposes provided that ECHA is acknowledged as the source: "Source: European Chemicals Agency, http://echa.europa.eu/". Such acknowledgement must be included in each copy of the material. ECHA permits and encourages organisations and individuals to create links to the ECHA website under the following cumulative conditions: Links can only be made to webpages that provide a link to the Legal Notice page. | |
Haftungsausschluss und Informationen zu In-Vitro-Forschungsprodukten
Bitte beachten Sie, dass alle Artikel und Produktinformationen, die auf BenchChem präsentiert werden, ausschließlich zu Informationszwecken bestimmt sind. Die auf BenchChem zum Kauf angebotenen Produkte sind speziell für In-vitro-Studien konzipiert, die außerhalb lebender Organismen durchgeführt werden. In-vitro-Studien, abgeleitet von dem lateinischen Begriff "in Glas", beinhalten Experimente, die in kontrollierten Laborumgebungen unter Verwendung von Zellen oder Geweben durchgeführt werden. Es ist wichtig zu beachten, dass diese Produkte nicht als Arzneimittel oder Medikamente eingestuft sind und keine Zulassung der FDA für die Vorbeugung, Behandlung oder Heilung von medizinischen Zuständen, Beschwerden oder Krankheiten erhalten haben. Wir müssen betonen, dass jede Form der körperlichen Einführung dieser Produkte in Menschen oder Tiere gesetzlich strikt untersagt ist. Es ist unerlässlich, sich an diese Richtlinien zu halten, um die Einhaltung rechtlicher und ethischer Standards in Forschung und Experiment zu gewährleisten.