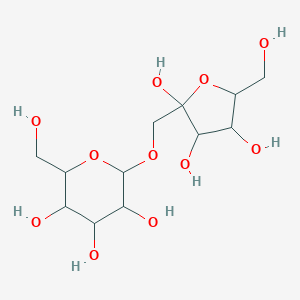
Trehalulose
Übersicht
Beschreibung
Trehalulose (1-O-α-D-glucopyranosyl-D-fructose) is a reducing disaccharide and structural isomer of sucrose, differing in its glycosidic linkage (1→1 vs. sucrose’s 1→2) . It is naturally abundant in stingless bee honey (Meliponini spp.), constituting up to 57% of total sugars in some samples . Key properties include:
- Low glycemic index (GI): Slow glucose release, making it suitable for diabetic diets .
- High solubility: ~650 g/L in water, exceeding sucrose (200 g/L) .
- Antioxidant activity: Contributes to stingless bee honey’s health benefits .
This compound is enzymatically synthesized from sucrose by bacterial sucrose isomerases (SIases), such as those from Pseudomonas mesoacidophila and Erwinia rhapontici, often alongside isomaltulose (6-O-α-D-glucopyranosyl-D-fructose) .
Vorbereitungsmethoden
Enzymatic Preparation Using Microbial Whole-Cell Systems
Protaminobacter rubrum CBS 574.77-Based Bioconversion
The patented method employing Protaminobacter rubrum CBS 574.77 demonstrates a robust whole-cell approach for trehalulose synthesis . The process begins with the preparation of a nutrient-rich medium containing thick juice (65% dry matter), corn steep liquor, and ammonium phosphate, adjusted to pH 7.2. Subcultured cells are incubated at 29°C for 30 hours in a shaker flask, followed by large-scale fermentation in a 30-liter bioreactor under controlled aeration (20 L/min) and agitation (350 rpm). Upon reaching a cell density of 5×10^9 organisms/mL, the biomass is harvested via centrifugation and immobilized for sucrose conversion.
Key Process Parameters:
Parameter | Value |
---|---|
Fermentation Temperature | 29°C |
pH | 7.2 |
Aeration Rate | 20 L/min |
Agitation Speed | 350 rpm |
Sucrose Concentration | 500 g/L |
Conversion Yield | 85% this compound |
This method achieves an 85% conversion yield of sucrose to this compound within 24 hours, with residual isomaltulose (10%) and minor byproducts . The immobilized cells retain 70% activity after five batch cycles, highlighting their operational stability.
Optimization with Pseudomonas mesoacidophila MX-45 Coculture
To enhance this compound specificity, the coculture of Pseudomonas mesoacidophila MX-45 and Protaminobacter rubrum CBS 574.77 has been explored . Varying strain ratios (1:1 to 1:4) modulate the product profile, with a 1:3 ratio yielding 92% this compound and 5% isomaltulose. The synergistic activity of these strains mitigates byproduct formation, as Pseudomonas preferentially metabolizes intermediates that otherwise accumulate.
Recombinant Trehalose Synthase (TreM) Mediated Synthesis
Enzyme Production via Heterologous Expression
The novel trehalose synthase TreM, identified from a thermal spring metagenome, offers a recombinant alternative for this compound production . The treM gene was cloned into Escherichia coli BL21(DE3) using a pET-28a(+) vector, inducing expression with 0.3 mM IPTG at 37°C. Purification via nickel-affinity chromatography yielded a 70 kDa enzyme with dual functionality for trehalose and this compound synthesis.
Purification Metrics:
Step | Total Protein (mg) | Activity (U/mg) | Purification Fold |
---|---|---|---|
Crude Extract | 120 | 15 | 1.0 |
Ni-Affinity Eluate | 38 | 112 | 7.5 |
Substrate Specificity and Kinetic Performance
TreM exhibits broad substrate versatility, catalyzing this compound synthesis from sucrose, jaggery, and cane molasses . Optimal conditions include pH 7.0, 50°C, and 300 mM sucrose, achieving a 90% conversion yield in 12 hours. Notably, the enzyme’s thermostability allows prolonged operation at 50°C, with only 20% activity loss over 24 hours.
Comparative Activity at Varied Temperatures:
Temperature (°C) | This compound Yield (%) | Byproduct (Glucose, %) |
---|---|---|
5 | 74 | 4 |
30 | 57 | 0 |
50 | 90 | 8 |
TreM’s cold-active properties (5°C) minimize glucose formation, making it suitable for high-purity applications .
Industrial-Scale Considerations and Feedstock Adaptation
Low-Cost Feedstock Utilization
TreM’s compatibility with industrial byproducts like cane molasses and sweet sorghum juice reduces production costs . Enzymatic treatment of jaggery (300 mM equivalent sucrose) yields 86% this compound, while muscovado sugar achieves 82% conversion.
Feedstock Efficiency:
Feedstock | This compound Yield (%) | Purity (%) |
---|---|---|
Refined Sucrose | 90 | 92 |
Cane Molasses | 85 | 88 |
Jaggery | 86 | 89 |
Bioreactor Design and Process Integration
Scale-up trials using immobilized Protaminobacter cells in a packed-bed reactor demonstrate continuous this compound production at 1.2 kg/L/day . In contrast, soluble TreM requires ultrafiltration modules for enzyme retention, achieving a productivity of 0.8 kg/L/day .
Comparative Analysis of Preparation Methods
Method | Yield (%) | Byproducts (%) | Scalability | Cost (USD/kg) |
---|---|---|---|---|
Protaminobacter Whole-Cell | 85 | 15 | High | 12.50 |
Recombinant TreM | 90 | 10 | Moderate | 18.75 |
Whole-cell systems offer cost advantages but face challenges in byproduct management. Recombinant enzymes provide higher specificity but incur higher production costs due to purification requirements.
Analyse Chemischer Reaktionen
Types of Reactions: 1-O-alpha-D-Glucopyranosyl-D-fructose undergoes various chemical reactions, including:
Oxidation: The compound can be oxidized to produce gluconic acid and fructonic acid.
Reduction: Reduction of 1-O-alpha-D-Glucopyranosyl-D-fructose can yield sugar alcohols such as glucitol and mannitol.
Substitution: The hydroxyl groups in the compound can participate in substitution reactions to form esters and ethers.
Common Reagents and Conditions:
Oxidation: Common oxidizing agents include nitric acid and potassium permanganate.
Reduction: Sodium borohydride and hydrogen gas in the presence of a catalyst are commonly used reducing agents.
Substitution: Acidic or basic catalysts are often employed to facilitate substitution reactions.
Major Products:
Oxidation: Gluconic acid, fructonic acid
Reduction: Glucitol, mannitol
Substitution: Various esters and ethers
Wissenschaftliche Forschungsanwendungen
Trehalulose is an intriguing disaccharide with unique properties that offer promising applications across multiple scientific domains. This comprehensive review explores its potential applications based on current research findings.
Nutritional and Metabolic Research
Glycemic Impact
- Significantly lower glycemic response compared to traditional sugars
- Approximately one-third the hydrolysis rate of standard sucrose
- Potential benefits for metabolic health management
Food Industry Innovations
Functional Food Development
- Potential use in:
- Ready-to-eat foods
- Baked goods (bread, cakes)
- Specialized dietary products
Biomedical Potential
Specialized Medical Applications
- Acariogenic Properties : Demonstrates potential in dental health research
- Low Glycemic Characteristics : Promising for diabetes management strategies
- Potential applications in developing specialized nutritional interventions
Research Findings Comparison
Application Domain | Key Characteristics | Potential Impact |
---|---|---|
Nutritional Science | Low glycemic index | Metabolic health management |
Food Technology | Unique sugar profile | Functional food development |
Medical Research | Reduced intestinal hydrolysis | Diabetes intervention strategies |
Biosynthesis Techniques
Recent studies have highlighted innovative production methodologies:
- Microbial conversion techniques
- Enzymatic transformation processes
- High-efficiency starch conversion methods (up to 80% yield)
Geographical Distribution of Research
This compound research has been notably concentrated in:
- Neotropical regions
- Indo-Australian geographical zones
- Advanced biotechnology research centers in Japan and Europe
Unique Discovery Contexts
Natural Sources
- Stingless Bee Honey : Discovered as a major component (13-44g per 100g)
- Identified across multiple bee species
- Confirmed through advanced analytical techniques like NMR and UPLC-MS/MS
Limitations and Future Perspectives
While promising, this compound research remains in early stages. Future investigations should focus on:
- Long-term metabolic impact studies
- Comprehensive clinical trials
- Advanced production scalability
- Potential industrial applications
Wirkmechanismus
The mechanism of action of 1-O-alpha-D-Glucopyranosyl-D-fructose involves its interaction with specific enzymes and transporters in biological systems. The compound is hydrolyzed by enzymes such as sucrase-isomaltase, releasing glucose and fructose, which are then absorbed and metabolized by the body. The molecular targets include various glycosidases and transport proteins involved in carbohydrate metabolism .
Vergleich Mit ähnlichen Verbindungen
Comparison with Structurally Similar Disaccharides
Structural and Physicochemical Properties
Functional and Metabolic Differences
- Digestion : this compound is hydrolyzed slowly by intestinal α-glucosidases, leading to gradual glucose absorption, unlike maltose (rapidly digested) or sucrose (intermediate rate) .
- Cariogenicity: this compound and isomaltulose are non-cariogenic, whereas sucrose and maltose promote dental caries .
- Antioxidant Capacity : this compound in stingless bee honey exhibits higher antioxidant activity than sucrose or maltose .
Analytical Differentiation from Similar Sugars
This compound has been historically misidentified as maltose in honey due to overlapping retention times in HPLC . Advanced methods resolve this:
- HPTLC : Retardation factor (RF) = 0.045 for this compound vs. 0.15–0.30 for glucose, fructose, and sucrose .
- UPLC-MS/MS : this compound fragments at m/z 179 (dominant ion) and lacks the m/z 221 peak characteristic of maltose/isomaltulose .
- NMR : Distinct <sup>13</sup>C signals at δ 93.5 ppm (C1 of fructose) confirm its α(1→1) linkage .
Enzymatic Production and Product Specificity
Sucrose isomerases (SIases) produce this compound and isomaltulose ratios dependent on enzyme source and conditions:
Mutations in SIases (e.g., SmuA-V465E) can shift product specificity toward isomaltulose, reducing this compound byproducts .
Q & A
Basic Research Questions
Q. How can trehalulose be reliably identified in complex biological matrices like honey?
this compound identification requires chromatographic separation coupled with structural validation. High-performance thin-layer chromatography (HPTLC) using silica gel 60 F254 plates and a mobile phase of 1-butanol-2-propanol-aqueous boric acid (30:50:10, V/V) achieves baseline separation from fructose, glucose, maltose, and sucrose (RF = 0.045 for this compound vs. 0.11–0.29 for other sugars). Post-separation derivatization with aniline-diphenylamine-phosphoric acid reagent produces a distinct orange-brown band for this compound. Confirmatory analyses like 2D-HPTLC or NMR should be used to rule out co-elution with interfering compounds .
Q. What experimental approaches are used to investigate this compound's role as a biomarker for stingless bee honey authentication?
Comparative sugar profiling via HPTLC or UPLC-MS/MS across honey samples from diverse bee species (e.g., Tetragonula, Heterotrigona) can establish this compound's uniqueness. Key steps include:
- Quantifying this compound levels (typically 13–44 g/100 g in stingless bee honey).
- Demonstrating absence in Apis mellifera (common honeybee) honey.
- Correlating this compound concentration with geographic or species-specific factors. Validation requires parallel NMR analysis to confirm glycosidic linkage (α-1,1 for this compound vs. α-1,4 for maltose) .
Q. Which analytical methods are most suitable for quantifying this compound, and what are their limitations?
- HPTLC : Cost-effective for high-throughput screening (linear range: 100–800 ng/band; LOD: 20.04 ng/band). Validated per ICH Q2(R1) guidelines for specificity, precision (RSD < 2%), and recovery (>98%). Limited by lower resolution compared to HPLC .
- HPLC/UPLC-MS/MS : Higher sensitivity and specificity but requires expensive instrumentation and specialized columns (e.g., NH2-bonded phases). Suitable for trace analysis .
- NMR : Gold standard for structural confirmation but impractical for routine quantification due to low throughput and high sample requirements .
Advanced Research Questions
Q. How can researchers validate the specificity of an HPTLC method for this compound in the presence of structurally similar sugars?
Specificity validation involves:
- Overspotting tests : Spiking honey samples with this compound standards and confirming band purity via 2D chromatography.
- Forced degradation studies : Exposing samples to heat, acid, or enzymatic hydrolysis to assess interference from degradation products.
- Peak purity analysis : Using spectral overlays in HPTLC software (e.g., visionCATS) to verify single-component bands .
Q. What experimental designs resolve contradictions in this compound quantification data across different analytical platforms?
Discrepancies often arise from:
- Matrix effects : Honey composition (e.g., phenolic acids) may interfere with detection. Mitigate via sample cleanup (solid-phase extraction) or standard addition calibration .
- Method sensitivity : Cross-validate using orthogonal techniques (e.g., HPTLC for screening, NMR for confirmation).
- Enzymatic interconversion : Monitor sucrose-to-trehalulose conversion during storage using time-course studies with α-glucosidase inhibitors .
Q. What enzymatic mechanisms drive this compound biosynthesis in stingless bees, and how can these be experimentally characterized?
this compound synthesis involves sucrose isomerase activity in bee salivary glands. Key steps for mechanistic studies:
- In vitro assays : Incubate sucrose with bee-derived enzyme extracts and quantify this compound via HPTLC.
- Substrate specificity tests : Compare conversion rates of sucrose vs. glucose/fructose mixtures (no this compound forms without sucrose) .
- Kinetic analysis : Determine Km and Vmax for the isomerase using Lineweaver-Burk plots .
Q. How can researchers optimize this compound production in microbial systems for functional studies?
Recombinant expression of sucrose isomerases (e.g., Pantoea dispersa SI or Deinococcus geothermalis amylosucrase) in E. coli enables scalable production. Optimization strategies include:
- pH/Temperature modulation : P. dispersa SI achieves >90% this compound yield at pH 5 and 35°C.
- Feedstock engineering : Use low-cost substrates like molasses or jaggery instead of pure sucrose .
- Enzyme immobilization : Enhance thermostability (e.g., 80% activity retention after 24h at 50°C for D. geothermalis amylosucrase) .
Q. Methodological Considerations
Q. What parameters are critical for ensuring robustness in this compound HPTLC quantification?
Robustness testing requires evaluating:
- Mobile phase variability : ±2 mL solvent volume tolerance.
- Plate batch differences : Assess inter-plate RF consistency (target: 0.045 ± 0.002).
- Detection wavelength : T white illumination provides optimal accuracy (vs. R white or RT white) .
Q. How should researchers design in vitro studies to assess this compound's bioactivity (e.g., antioxidant or glycemic effects)?
- Antioxidant assays : Use DPPH/ABTS radical scavenging models with dose-response curves (1–100 mg/mL this compound).
- Glycemic impact : Measure glucose uptake in Caco-2/HT-29 cell co-cultures or murine models, comparing this compound to sucrose/maltose .
- Control for confounding factors : Include purity checks (HPLC) to exclude residual fructose/glucose effects .
Eigenschaften
IUPAC Name |
2-(hydroxymethyl)-6-[[2,3,4-trihydroxy-5-(hydroxymethyl)oxolan-2-yl]methoxy]oxane-3,4,5-triol | |
---|---|---|
Details | Computed by Lexichem TK 2.7.0 (PubChem release 2021.05.07) | |
Source | PubChem | |
URL | https://pubchem.ncbi.nlm.nih.gov | |
Description | Data deposited in or computed by PubChem | |
InChI |
InChI=1S/C12H22O11/c13-1-4-6(15)8(17)9(18)11(22-4)21-3-12(20)10(19)7(16)5(2-14)23-12/h4-11,13-20H,1-3H2 | |
Details | Computed by InChI 1.0.6 (PubChem release 2021.05.07) | |
Source | PubChem | |
URL | https://pubchem.ncbi.nlm.nih.gov | |
Description | Data deposited in or computed by PubChem | |
InChI Key |
NMXLJRHBJVMYPD-UHFFFAOYSA-N | |
Details | Computed by InChI 1.0.6 (PubChem release 2021.05.07) | |
Source | PubChem | |
URL | https://pubchem.ncbi.nlm.nih.gov | |
Description | Data deposited in or computed by PubChem | |
Canonical SMILES |
C(C1C(C(C(C(O1)OCC2(C(C(C(O2)CO)O)O)O)O)O)O)O | |
Details | Computed by OEChem 2.3.0 (PubChem release 2021.05.07) | |
Source | PubChem | |
URL | https://pubchem.ncbi.nlm.nih.gov | |
Description | Data deposited in or computed by PubChem | |
Molecular Formula |
C12H22O11 | |
Details | Computed by PubChem 2.1 (PubChem release 2021.05.07) | |
Source | PubChem | |
URL | https://pubchem.ncbi.nlm.nih.gov | |
Description | Data deposited in or computed by PubChem | |
Molecular Weight |
342.30 g/mol | |
Details | Computed by PubChem 2.1 (PubChem release 2021.05.07) | |
Source | PubChem | |
URL | https://pubchem.ncbi.nlm.nih.gov | |
Description | Data deposited in or computed by PubChem | |
Physical Description |
Solid | |
Record name | Trehalulose | |
Source | Human Metabolome Database (HMDB) | |
URL | http://www.hmdb.ca/metabolites/HMDB0039727 | |
Description | The Human Metabolome Database (HMDB) is a freely available electronic database containing detailed information about small molecule metabolites found in the human body. | |
Explanation | HMDB is offered to the public as a freely available resource. Use and re-distribution of the data, in whole or in part, for commercial purposes requires explicit permission of the authors and explicit acknowledgment of the source material (HMDB) and the original publication (see the HMDB citing page). We ask that users who download significant portions of the database cite the HMDB paper in any resulting publications. | |
CAS No. |
51411-23-5 | |
Record name | Trehalulose | |
Source | Human Metabolome Database (HMDB) | |
URL | http://www.hmdb.ca/metabolites/HMDB0039727 | |
Description | The Human Metabolome Database (HMDB) is a freely available electronic database containing detailed information about small molecule metabolites found in the human body. | |
Explanation | HMDB is offered to the public as a freely available resource. Use and re-distribution of the data, in whole or in part, for commercial purposes requires explicit permission of the authors and explicit acknowledgment of the source material (HMDB) and the original publication (see the HMDB citing page). We ask that users who download significant portions of the database cite the HMDB paper in any resulting publications. | |
Melting Point |
90 - 95 °C | |
Record name | Trehalulose | |
Source | Human Metabolome Database (HMDB) | |
URL | http://www.hmdb.ca/metabolites/HMDB0039727 | |
Description | The Human Metabolome Database (HMDB) is a freely available electronic database containing detailed information about small molecule metabolites found in the human body. | |
Explanation | HMDB is offered to the public as a freely available resource. Use and re-distribution of the data, in whole or in part, for commercial purposes requires explicit permission of the authors and explicit acknowledgment of the source material (HMDB) and the original publication (see the HMDB citing page). We ask that users who download significant portions of the database cite the HMDB paper in any resulting publications. | |
Retrosynthesis Analysis
AI-Powered Synthesis Planning: Our tool employs the Template_relevance Pistachio, Template_relevance Bkms_metabolic, Template_relevance Pistachio_ringbreaker, Template_relevance Reaxys, Template_relevance Reaxys_biocatalysis model, leveraging a vast database of chemical reactions to predict feasible synthetic routes.
One-Step Synthesis Focus: Specifically designed for one-step synthesis, it provides concise and direct routes for your target compounds, streamlining the synthesis process.
Accurate Predictions: Utilizing the extensive PISTACHIO, BKMS_METABOLIC, PISTACHIO_RINGBREAKER, REAXYS, REAXYS_BIOCATALYSIS database, our tool offers high-accuracy predictions, reflecting the latest in chemical research and data.
Strategy Settings
Precursor scoring | Relevance Heuristic |
---|---|
Min. plausibility | 0.01 |
Model | Template_relevance |
Template Set | Pistachio/Bkms_metabolic/Pistachio_ringbreaker/Reaxys/Reaxys_biocatalysis |
Top-N result to add to graph | 6 |
Feasible Synthetic Routes
Haftungsausschluss und Informationen zu In-Vitro-Forschungsprodukten
Bitte beachten Sie, dass alle Artikel und Produktinformationen, die auf BenchChem präsentiert werden, ausschließlich zu Informationszwecken bestimmt sind. Die auf BenchChem zum Kauf angebotenen Produkte sind speziell für In-vitro-Studien konzipiert, die außerhalb lebender Organismen durchgeführt werden. In-vitro-Studien, abgeleitet von dem lateinischen Begriff "in Glas", beinhalten Experimente, die in kontrollierten Laborumgebungen unter Verwendung von Zellen oder Geweben durchgeführt werden. Es ist wichtig zu beachten, dass diese Produkte nicht als Arzneimittel oder Medikamente eingestuft sind und keine Zulassung der FDA für die Vorbeugung, Behandlung oder Heilung von medizinischen Zuständen, Beschwerden oder Krankheiten erhalten haben. Wir müssen betonen, dass jede Form der körperlichen Einführung dieser Produkte in Menschen oder Tiere gesetzlich strikt untersagt ist. Es ist unerlässlich, sich an diese Richtlinien zu halten, um die Einhaltung rechtlicher und ethischer Standards in Forschung und Experiment zu gewährleisten.