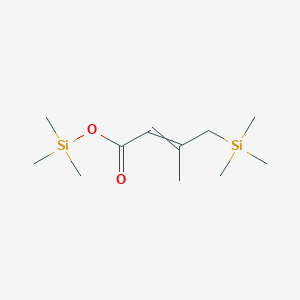
Trimethylsilyl 3-methyl-4-(trimethylsilyl)but-2-enoate
Übersicht
Beschreibung
Trimethylsilyl 3-methyl-4-(trimethylsilyl)but-2-enoate is a silyl ester characterized by a but-2-enoate backbone with a methyl group at position 3 and a trimethylsilyl (TMS) group at position 4. The ester functionality is also protected by a trimethylsilyl group, resulting in two TMS substituents. This structural configuration enhances steric bulk and lipophilicity, which may influence its reactivity and stability in synthetic applications.
Vorbereitungsmethoden
Silylation of Dienolic Intermediates
The foundational approach to synthesizing Trimethylsilyl 3-methyl-4-(trimethylsilyl)but-2-enoate involves sequential silylation of a dienolic precursor. A key method, adapted from palladium-catalyzed coupling protocols, begins with the reaction of 3-methyl-4-hydroxybut-2-enoic acid with trimethylsilyl chloride (TMSCl) in the presence of a base such as diisopropylethylamine (DIPEA). The reaction proceeds via nucleophilic substitution, where the hydroxyl groups at the 3- and 4-positions are replaced by trimethylsilyl moieties .
Reaction Conditions:
-
Solvent: Tetrahydrofuran (THF) or dichloromethane (DCM)
-
Temperature: 0°C to room temperature
-
Base: DIPEA or triethylamine (TEA)
Critical to this method is the order of silylation. Introducing the first trimethylsilyl group at the 4-position prior to esterification minimizes steric hindrance, as demonstrated in analogous syntheses of methyl 3-((trimethylsilyl)oxy)-2-butenoate . A representative reaction pathway is shown below:
Palladium-Catalyzed Cross-Coupling for Direct Silylation
A second method leverages palladium catalysis to install both trimethylsilyl groups in a single step. This route, inspired by the synthesis of palbociclib intermediates, employs a palladium(II) catalyst (e.g., trans-dichlorobis(acetonitrile)palladium(II)) with tri-o-tolylphosphine as a ligand . The substrate, typically a halogenated enoate, reacts with hexamethyldisilane (HMDS) under controlled conditions.
Optimized Protocol:
-
Catalyst: trans-Dichlorobis(acetonitrile)palladium(II) (2–5 mol%)
-
Ligand: Tri-o-tolylphosphine (2–5 mol%)
-
Base: DIPEA or potassium carbonate
-
Solvent: THF or toluene
-
Temperature: 70–80°C
-
Reaction Time: 12–18 hours
This method is advantageous for substrates sensitive to strong bases, as the palladium complex facilitates oxidative addition of the silicon reagent. However, the requirement for rigorous degassing (via nitrogen/vacuum cycles) to prevent catalyst deactivation adds complexity .
Sequential Esterification and Silylation
A stepwise approach involves first synthesizing the methyl ester of 3-methyl-4-(trimethylsilyl)but-2-enoic acid, followed by silylation of the remaining hydroxyl group. This method, detailed in analogous syntheses of methyl 3-(trimethylsilyl)-4-pentenoate, uses trimethylsilyl trifluoromethanesulfonate (TMSOTf) as a potent silylating agent .
Procedure Summary:
-
Esterification: React 3-methyl-4-hydroxybut-2-enoic acid with methanol in the presence of sulfuric acid.
-
Silylation: Treat the intermediate methyl ester with TMSOTf and 2,6-lutidine in DCM at –78°C.
-
Workup: Quench with aqueous sodium bicarbonate and purify via silica gel chromatography.
Key Data:
This method offers flexibility in modifying the ester group but requires low-temperature conditions to suppress side reactions such as over-silylation.
Intramolecular Cyclization of Silylated Precursors
Intramolecular cyclization presents an alternative route for constructing the bis-silylated structure. A patent describing the synthesis of palbociclib intermediates highlights the use of acid anhydrides (e.g., acetic anhydride) to promote cyclization of silylated enol ethers . For this compound, this method involves:
-
Preparing a mono-silylated enol ether via reaction with TMSCl.
-
Subjecting the intermediate to acetic anhydride at 70–80°C for 3 hours.
-
Isolating the product via acid-base extraction and distillation.
Advantages:
-
Reduced Purification Steps: Cyclization minimizes byproducts.
Challenges:
-
Strict temperature control is required to prevent desilylation.
Comparative Analysis of Methods
The table below evaluates the four methods based on yield, complexity, and applicability:
Analyse Chemischer Reaktionen
Types of Reactions
Trimethylsilyl 3-methyl-4-(trimethylsilyl)but-2-enoate undergoes various chemical reactions, including:
Oxidation: The compound can be oxidized to form corresponding oxides.
Reduction: Reduction reactions can yield alcohols or other reduced forms.
Substitution: The trimethylsilyl groups can be substituted with other functional groups under appropriate conditions.
Common Reagents and Conditions
Oxidation: Common oxidizing agents include potassium permanganate and chromium trioxide.
Reduction: Reducing agents such as lithium aluminum hydride or sodium borohydride are used.
Substitution: Reagents like halides or nucleophiles can facilitate substitution reactions.
Major Products
The major products formed from these reactions depend on the specific reagents and conditions used. For example, oxidation may yield carboxylic acids, while reduction can produce alcohols .
Wissenschaftliche Forschungsanwendungen
Organic Synthesis
Trimethylsilyl 3-methyl-4-(trimethylsilyl)but-2-enoate is primarily utilized as an intermediate in organic synthesis. Its unique structural features allow it to participate in different chemical transformations, which are crucial for synthesizing complex organic molecules. The compound can be synthesized from 3-methyl-4-butenoic acid and trimethylsilyl chloride through a straightforward reaction, producing hydrochloric acid as a byproduct:
This reaction highlights the compound's utility as a building block in organic chemistry.
Medicinal Chemistry
In the realm of medicinal chemistry, this compound has been investigated for its potential role in drug development. Its structural similarity to other bioactive compounds suggests that it may interact with biological targets, warranting further exploration into its pharmacodynamics and pharmacokinetics. For instance, research indicates that silyl-protected derivatives, including this compound, can be used in the synthesis of pharmaceuticals like palbociclib, a drug for treating certain types of cancer .
Materials Science
The compound's dual trimethylsilyl groups contribute to its unique properties, making it valuable in materials science applications. These groups enhance the compound's stability and solubility in organic solvents, allowing it to be used in the formulation of advanced materials such as polymers and coatings. The incorporation of such silylated compounds can improve the mechanical and thermal properties of materials.
Case Studies and Research Findings
Several studies have highlighted the applications of this compound:
- Synthesis of Bioactive Compounds : Research has demonstrated its role as an intermediate in synthesizing bioactive molecules, showcasing its importance in drug discovery .
- Asymmetric Synthesis : The compound has been employed in asymmetric synthesis processes, improving yields and selectivity in complex reactions .
- Polymer Development : Investigations into its use in polymer formulations have shown enhanced properties like thermal stability and mechanical strength due to the incorporation of silylated functionalities.
Wirkmechanismus
The mechanism of action of Trimethylsilyl 3-methyl-4-(trimethylsilyl)but-2-enoate involves its ability to act as a protecting group for reactive functional groups in organic synthesis. The trimethylsilyl groups shield the functional groups from unwanted reactions, allowing for selective transformations. The compound can also participate in radical reactions, where it acts as a radical initiator or stabilizer .
Vergleich Mit ähnlichen Verbindungen
Comparison with Structurally Similar Compounds
Trimethylsilyl (E)-4-bromobut-2-enoate
Key Differences :
- Substituents : The bromo analog (CAS: 88239-39-8) features a bromine atom at position 4 instead of a TMS group. Bromine’s electronegativity and leaving-group ability contrast with the inert, sterically bulky TMS group in the target compound .
- Reactivity : The bromo compound is more reactive in nucleophilic substitution or elimination reactions, whereas the TMS groups in the target compound likely stabilize the structure against hydrolysis or oxidation.
- Physicochemical Properties :
*Calculated based on structural inference.
Silylated Amino Acids (e.g., N,O-BIS-(trimethylsilyl) isoleucine)
Key Differences :
- Backbone Structure: Silylated amino acids like N,O-BIS-(trimethylsilyl) isoleucine (C₁₂H₂₉O₂NSi₂) feature amino acid cores, whereas the target compound is based on a but-2-enoate ester .
- Applications: Amino acid derivatives are primarily used for GC-MS analysis to enhance volatility. The target compound’s non-polar backbone and dual TMS groups may make it suitable for specialized synthetic protocols or hydrophobic reaction environments.
Methyl Esters of Sulfonylurea Herbicides (e.g., Metsulfuron-methyl)
Key Differences :
- Ester Stability : Methyl esters (e.g., metsulfuron-methyl) are prone to hydrolysis under acidic or basic conditions, while silyl esters like the target compound are more resistant due to the TMS group’s shielding effect .
- Functionality: Sulfonylurea herbicides act as acetyl-CoA carboxylase inhibitors, whereas the target compound’s role is likely confined to synthetic or analytical chemistry due to its non-bioactive structure.
Research Findings and Implications
- Steric Effects : The dual TMS groups in the target compound likely reduce reactivity at the carbonyl center, making it a stable intermediate in multi-step syntheses.
- Lipophilicity: Increased hydrophobicity compared to bromo or methyl analogs may enhance solubility in non-polar solvents, useful in organometallic reactions .
- Analytical Utility: Similar to silylated amino acids, the compound could serve as a derivatization agent for GC-MS, though its retention behavior would differ due to the non-polar backbone .
Biologische Aktivität
Trimethylsilyl 3-methyl-4-(trimethylsilyl)but-2-enoate is a compound of interest in organic chemistry and medicinal research due to its unique structural properties and potential biological activities. This article explores its biological activity, synthesis, and relevant research findings, supported by data tables and case studies.
Chemical Structure and Properties
This compound is characterized by its silyl ether functional groups, which enhance its stability and reactivity in various chemical reactions. The general structure can be represented as follows:
This compound is often synthesized for use in organic transformations and has been studied for its potential applications in medicinal chemistry.
Antiviral Activity
Recent studies have highlighted the antiviral potential of compounds similar to this compound. For instance, derivatives of this compound have shown promising activity against viral proteases, which are critical for viral replication. A notable example includes the inhibition of papain-like protease (PLpro) in SARS-CoV-2, where compounds exhibiting similar structural motifs demonstrated significant antiviral activity with an effective concentration (EC50) comparable to established antiviral drugs like remdesivir .
Anticancer Properties
The anticancer potential of trimethylsilyl derivatives has also been explored. Compounds with similar silyl modifications have been reported to exhibit selective cytotoxicity against various cancer cell lines, including multiple myeloma. The mechanism involves the inhibition of proteasomal subunits, leading to apoptosis in cancer cells. For example, studies show that certain silyl-containing compounds can inhibit the β2 and β5 subunits of the proteasome, which is crucial for maintaining cellular protein homeostasis .
Synthesis and Structure-Activity Relationship (SAR)
The synthesis of this compound typically involves the use of trimethylsilyl reagents in conjunction with various coupling strategies. The structure-activity relationship indicates that modifications to the silyl groups can significantly influence biological activity. For instance, variations in substitution patterns on the but-2-enoate moiety can enhance or diminish inhibitory effects against specific biological targets.
Table 1: Summary of Biological Activities
Case Studies
- Antiviral Efficacy : A study evaluated a series of trimethylsilyl compounds against SARS-CoV-2 PLpro, revealing that certain derivatives exhibited potent antiviral activity with minimal cytotoxic effects on uninfected cells .
- Cancer Cell Line Testing : Research conducted on various cancer cell lines demonstrated that trimethylsilyl derivatives induced apoptosis through proteasome inhibition, with enhanced effects observed in multiple myeloma cells compared to solid tumors .
Q & A
Basic Research Questions
Q. What are the optimal synthetic routes for preparing Trimethylsilyl 3-methyl-4-(trimethylsilyl)but-2-enoate, and how can its purity be verified?
- Methodological Answer : Synthesis typically involves silylation of the corresponding enoic acid using trimethylsilyl chloride in the presence of a base (e.g., triethylamine). Protecting group strategies, such as sequential silylation at the 1- and 4-positions, are critical to avoid side reactions. Purity verification requires gas chromatography-mass spectrometry (GC-MS) to confirm molecular weight and detect impurities, coupled with H and C NMR to validate structural integrity. For silylated compounds, ensure anhydrous conditions during synthesis to prevent hydrolysis .
Q. How should this compound be stored to maintain stability, and what degradation products are likely?
- Methodological Answer : Store the compound in airtight containers under inert gas (argon/nitrogen) at temperatures below -20°C to prevent hydrolysis or oxidation. Degradation products may include desilylated enoic acids or ketones, detectable via GC-MS by comparing retention times and fragmentation patterns to reference standards. Regular stability testing under varying conditions (humidity, temperature) is advised to establish shelf-life .
Advanced Research Questions
Q. What factors influence the dimerization kinetics of this compound, and how can product distribution be controlled?
- Methodological Answer : Dimerization is influenced by steric effects from the α-methyl and trimethylsilyl groups, solvent polarity, and temperature. For example, non-polar solvents like toluene favor [4+2] cycloaddition, while polar solvents may promote alternative pathways. Control product distribution by adjusting reaction time and monitoring via H NMR for intermediate trapping. Computational modeling (DFT) can predict transition states to optimize conditions for selective dimer formation .
Q. How can researchers resolve contradictions in spectroscopic data for silylated intermediates, such as unexpected downfield shifts in C NMR?
- Methodological Answer : Discrepancies in C NMR shifts often arise from steric strain or electronic effects of adjacent silyl groups. Cross-validate using 2D NMR techniques (e.g., HSQC, HMBC) to confirm connectivity. Compare data with structurally analogous compounds (e.g., Methyl 4-((trimethylsilyl)ethynyl)benzoate, which exhibits similar deshielding patterns) to distinguish artifacts from true structural features .
Q. What computational methods are effective in predicting the reactivity of this compound in nucleophilic environments?
- Methodological Answer : Density Functional Theory (DFT) calculations (e.g., B3LYP/6-31G*) can model the electron-withdrawing effects of silyl groups on the enoate’s LUMO energy, predicting reactivity toward nucleophiles. Solvent effects are incorporated via PCM models. Validate predictions with kinetic studies (e.g., monitoring reaction rates via UV-Vis spectroscopy) .
Q. Data Contradiction and Experimental Design
Q. How should researchers address inconsistent yields in silylation reactions involving this compound?
- Methodological Answer : Inconsistent yields may stem from residual moisture or incomplete base activation. Implement rigorous drying protocols (e.g., molecular sieves, flame-dried glassware) and quantify base equivalents via titration. Use in situ FTIR to monitor silylation progress (disappearance of -OH stretches at ~3200 cm). If side products dominate, employ preparative HPLC to isolate the target compound and characterize by high-resolution MS .
Q. What strategies optimize the separation of stereoisomers formed during reactions of this compound?
- Methodological Answer : Chiral stationary phases (e.g., cellulose tris(3,5-dimethylphenylcarbamate)) in HPLC effectively resolve enantiomers. Pre-derivatization with a chiral auxiliary (e.g., Mosher’s acid) enhances separation. Confirm stereochemical assignments via X-ray crystallography or NOESY NMR to correlate spatial proximity of substituents .
Eigenschaften
IUPAC Name |
trimethylsilyl 3-methyl-4-trimethylsilylbut-2-enoate | |
---|---|---|
Source | PubChem | |
URL | https://pubchem.ncbi.nlm.nih.gov | |
Description | Data deposited in or computed by PubChem | |
InChI |
InChI=1S/C11H24O2Si2/c1-10(9-14(2,3)4)8-11(12)13-15(5,6)7/h8H,9H2,1-7H3 | |
Source | PubChem | |
URL | https://pubchem.ncbi.nlm.nih.gov | |
Description | Data deposited in or computed by PubChem | |
InChI Key |
QCGGJRHSTCBUNL-UHFFFAOYSA-N | |
Source | PubChem | |
URL | https://pubchem.ncbi.nlm.nih.gov | |
Description | Data deposited in or computed by PubChem | |
Canonical SMILES |
CC(=CC(=O)O[Si](C)(C)C)C[Si](C)(C)C | |
Source | PubChem | |
URL | https://pubchem.ncbi.nlm.nih.gov | |
Description | Data deposited in or computed by PubChem | |
Molecular Formula |
C11H24O2Si2 | |
Source | PubChem | |
URL | https://pubchem.ncbi.nlm.nih.gov | |
Description | Data deposited in or computed by PubChem | |
DSSTOX Substance ID |
DTXSID40369946 | |
Record name | Trimethylsilyl 3-methyl-4-(trimethylsilyl)but-2-enoate | |
Source | EPA DSSTox | |
URL | https://comptox.epa.gov/dashboard/DTXSID40369946 | |
Description | DSSTox provides a high quality public chemistry resource for supporting improved predictive toxicology. | |
Molecular Weight |
244.48 g/mol | |
Source | PubChem | |
URL | https://pubchem.ncbi.nlm.nih.gov | |
Description | Data deposited in or computed by PubChem | |
CAS No. |
109751-82-8 | |
Record name | Trimethylsilyl 3-methyl-4-(trimethylsilyl)but-2-enoate | |
Source | EPA DSSTox | |
URL | https://comptox.epa.gov/dashboard/DTXSID40369946 | |
Description | DSSTox provides a high quality public chemistry resource for supporting improved predictive toxicology. | |
Haftungsausschluss und Informationen zu In-Vitro-Forschungsprodukten
Bitte beachten Sie, dass alle Artikel und Produktinformationen, die auf BenchChem präsentiert werden, ausschließlich zu Informationszwecken bestimmt sind. Die auf BenchChem zum Kauf angebotenen Produkte sind speziell für In-vitro-Studien konzipiert, die außerhalb lebender Organismen durchgeführt werden. In-vitro-Studien, abgeleitet von dem lateinischen Begriff "in Glas", beinhalten Experimente, die in kontrollierten Laborumgebungen unter Verwendung von Zellen oder Geweben durchgeführt werden. Es ist wichtig zu beachten, dass diese Produkte nicht als Arzneimittel oder Medikamente eingestuft sind und keine Zulassung der FDA für die Vorbeugung, Behandlung oder Heilung von medizinischen Zuständen, Beschwerden oder Krankheiten erhalten haben. Wir müssen betonen, dass jede Form der körperlichen Einführung dieser Produkte in Menschen oder Tiere gesetzlich strikt untersagt ist. Es ist unerlässlich, sich an diese Richtlinien zu halten, um die Einhaltung rechtlicher und ethischer Standards in Forschung und Experiment zu gewährleisten.