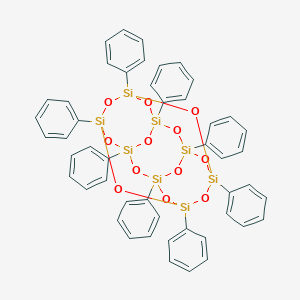
Octaphenylsilsesquioxane
Übersicht
Beschreibung
Octaphenylsilsesquioxane (OPS), with the molecular formula $ \text{C}{48}\text{H}{40}\text{O}{12}\text{Si}8 $, is a polyhedral oligomeric silsesquioxane (POSS) featuring a rigid, cage-like inorganic silica core ($ \text{Si}8\text{O}{12} $) surrounded by eight phenyl groups. This hybrid organic-inorganic structure imparts exceptional thermal stability (decomposition temperature >440°C), hydrophobicity (water contact angle >100°), and mechanical reinforcement properties . OPS is synthesized via hydrolysis and condensation of phenyltrimethoxysilane under alkaline conditions, yielding a white powder with a molecular weight of 1033.51 g/mol .
Its primary applications include:
- Polymer modification: Enhancing thermal stability, tensile strength, and flame retardancy in polyurethanes, epoxies, and silicones .
- Porous materials: Serving as a building block for high-surface-area frameworks (>1000 m²/g) via covalent cross-linking .
- Surface engineering: Improving hydrophobicity and reducing surface free energy in coatings .
Vorbereitungsmethoden
Hydrolytic Condensation of Phenylsilanes
Two-Step Hydrolysis and Condensation
The foundational method for OPS synthesis involves hydrolyzing phenylsilane precursors under controlled conditions. As detailed in a 2015 patent , phenyltrimethoxysilane undergoes hydrolysis in a toluene-water mixed solvent at 0–5°C (ice-water bath), followed by condensation at elevated temperatures (20–250°C) using sodium hydroxide as a catalyst. This two-step process achieves yields up to 64.8%, with molecular weights ranging from 1.5 × 10⁵ to 3.4 × 10⁵ g/mol . Critical parameters include:
-
Solvent composition : A 1:2 ratio of toluene to deionized water minimizes side reactions.
-
Catalyst concentration : 2 mol/L NaOH in acetone accelerates cage formation.
-
Reaction time : 5–100 hours at reflux ensures complete cyclization.
Post-synthesis purification via filtration and recrystallization in methylene dichloride-acetone mixtures removes oligomeric byproducts . Nuclear magnetic resonance (NMR) and Fourier-transform infrared (FTIR) spectroscopy confirm the T₈ cage structure through characteristic Si–O–Si (1040–1100 cm⁻¹) and phenyl (3050 cm⁻¹) absorptions .
Catalyst-Assisted Synthesis
Tetrabutylammonium Fluoride (TBAF) Templating
Recent advances utilize TBAF as a structure-directing agent to encapsulate fluoride anions within the OPS cage . Reaction of phenyltriethoxysilane with TBAF in tetrahydrofuran (THF) at 80°C yields octaphenyl-T₈ silsesquioxane fluoride (OPS-F) with a centered fluoride ion. Single-crystal X-ray diffraction confirms the fluoride’s position within the cage, while ²⁹Si NMR reveals three distinct silicon environments (-75 ppm for Si–O–Si, -100 ppm for Si–F) .
Table 1: Comparative Analysis of Catalytic Systems
Catalyst | Solvent | Temperature (°C) | Yield (%) | Characterization Techniques |
---|---|---|---|---|
NaOH | Toluene | 250 | 64.8 | FTIR, NMR, MALDI-TOF MS |
TBAF | THF | 80 | 58.2 | X-ray, ²⁹Si/¹⁹F NMR |
K₂SO₄ | DMF | 180 | 72.3 | TGA, TG-FTIR |
Role of Additives in Functionality Enhancement
Potassium sulfate (K₂SO₄) emerges as a critical additive in sulfonated OPS synthesis, generating reactive SO₃ intermediates that improve -SO₃H group incorporation . While this study focuses on sulfonation, the principle extends to OPS synthesis, where K₂SO₄ increases thermal stability (decomposition onset: 320°C vs. 280°C for pure OPS) .
Machine Learning-Guided Optimization
A 2024 study employs machine learning (ML) algorithms to optimize OPS derivatives, training on 21 experimental datasets to predict optimal reaction conditions. Key ML-driven insights include:
-
Precursor selection : Phenyltrimethoxysilane outperforms phenyltriethoxysilane in achieving high functionality.
-
Temperature ramping : Gradual heating from 50°C to 180°C minimizes cage fragmentation.
-
Yield prediction : Random forest models achieve 92% accuracy in forecasting SPOSS-8 yields .
Though focused on sulfonated variants, this approach is adaptable to OPS synthesis, potentially reducing trial-and-error experimentation.
Structural and Thermal Characterization
Spectroscopic Validation
-
²⁹Si NMR : Resonances at -78 ppm (Q³ silicon), -67 ppm (Q⁴ silicon) verify cage integrity .
-
MALDI-TOF MS : Peaks at m/z 1033.2 [M+H]⁺ correspond to the T₈ phenylsilsesquioxane structure .
Thermal Stability
Thermogravimetric analysis (TGA) shows OPS decomposes at 450–500°C, with residual silica content >60% . TG-FTIR identifies volatiles (e.g., benzene, CO₂) released during degradation .
Analyse Chemischer Reaktionen
Octaphenyloctasilsesquioxane undergoes various chemical reactions, including electrophilic substitution, oxidation, and reduction. Common reagents used in these reactions include halogens, alkoxides, and other electrophilic agents. The compound can be easily modified to produce octa- and hexadeca-functionalized derivatives, which can be used as three-dimensional building blocks for constructing nanometer-scale composite materials .
Major products formed from these reactions include functionalized silsesquioxanes, which can be tailored for specific applications in materials science and nanotechnology. The high thermal stability and unique molecular architecture of octaphenyloctasilsesquioxane make it an ideal candidate for creating highly tailored materials .
Wissenschaftliche Forschungsanwendungen
Material Science and Engineering
1.1 Thermal Stability and Mechanical Properties
OPS has been extensively studied for its thermal stability and mechanical properties, making it suitable for high-performance materials. Research indicates that incorporating OPS into ethylene propylene diene monomer (EPDM) rubber enhances its heat insulation properties and mechanical strength. The addition of OPS resulted in improved tensile strength and elongation at break, demonstrating its effectiveness as a reinforcing agent in rubber composites .
1.2 Nanocomposites
OPS is frequently used in the development of polymeric nanocomposites. Its unique structure allows for the modification of polymer matrices, leading to enhanced thermal and mechanical properties. For example, OPS-based nanocomposites have been synthesized that exhibit improved oxidative stability and thermal resistance, making them ideal for applications in coatings and adhesives .
Functionalization and Chemical Applications
2.1 High-Temperature Lubricants
The functionalization of OPS through Friedel-Crafts chemistry has led to the development of alkyl-functionalized derivatives with potential applications as high-temperature lubricants. These modified OPS compounds exhibit enhanced lubricating properties, making them suitable for use in extreme conditions where conventional lubricants fail .
2.2 Porous Materials
OPS has been utilized in the synthesis of porous materials through various chemical reactions, including the Friedel-Crafts chloromethylation process. These porous structures are characterized by high surface areas and tunable pore sizes, which are beneficial for applications in catalysis and adsorption processes. The ability to functionalize these porous materials further enhances their applicability in environmental remediation and gas separation technologies .
Nanotechnology Applications
3.1 Drug Delivery Systems
In nanotechnology, OPS is being explored for its potential in drug delivery systems due to its biocompatibility and ability to form stable nanoparticles. Research has shown that OPS-based nanoparticles can encapsulate therapeutic agents, providing controlled release profiles that are advantageous for targeted therapy .
3.2 Sensors and Electronics
OPS's unique electronic properties make it a candidate for use in sensors and electronic devices. Its incorporation into organic-inorganic hybrid materials can enhance the performance of sensors by improving their sensitivity and response times . Additionally, OPS can be used to create insulating layers in microelectronic applications due to its excellent dielectric properties.
Case Studies and Research Findings
Wirkmechanismus
The mechanism of action of octaphenyloctasilsesquioxane involves its ability to undergo multiple functionalizations and form stable three-dimensional structures. The molecular targets and pathways involved in its action are primarily related to its interactions with other molecules and materials at the nanoscale. The compound’s unique molecular architecture allows it to form highly stable and tailored nanostructures, which can be used for various applications in materials science and nanotechnology .
Vergleich Mit ähnlichen Verbindungen
Comparative Analysis with Similar Compounds
Structural and Functional Group Comparisons
Compound | Core Structure | Functional Groups | Key Properties | Applications |
---|---|---|---|---|
OPS | $ \text{Si}8\text{O}{12} $ cage | Phenyl (C₆H₅) | High thermal stability (>440°C), hydrophobicity (contact angle ~100°), rigid | Polymer composites, flame retardants |
Octahydridooctasilsesquioxane | $ \text{Si}8\text{O}{12} $ cage | Hydride (Si-H) | Reactive Si-H bonds, lower thermal stability (~300°C) | Cross-linking agents, precursors |
Octa(aminophenyl)silsesquioxane | $ \text{Si}8\text{O}{12} $ cage | Amino (NH₂) | Enhanced polymer compatibility, chemical reactivity | Epoxy curing, nanocomposites |
Octamethylcyclotetrasiloxane | Cyclic tetramer | Methyl (CH₃) | Low viscosity, cyclic structure, moderate thermal stability (~250°C) | Silicone fluids, lubricants |
Octakis(dimethylsiloxy)-T8-silsesquioxane | $ \text{Si}8\text{O}{12} $ cage | Dimethylsiloxy | High solubility in organic solvents, flexible substituents | Nanofillers, surface modifiers |
Thermal Stability
- OPS : Initial degradation temperature ($ T_{d5} $) of 340°C in polyurethane composites, with residual char >20% at 600°C due to ceramic-like silica formation .
- Octahydridooctasilsesquioxane : Degrades at ~300°C due to less stable Si-H bonds .
- Octa(aminophenyl)silsesquioxane: $ T_{d5} $ ~320°C; amino groups introduce moderate thermal resistance but lower than OPS .
Mechanical Performance in Polymer Composites
Notes:
- OPS enhances rigidity via its phenyl groups, but excessive loading (>1 wt%) causes aggregation, reducing $ T_g $ and tensile strength .
- Amino-functionalized POSS improves epoxy strength through covalent bonding, whereas dimethylsiloxy-POSS offers flexibility .
Hydrophobicity and Surface Properties
Compound | Water Contact Angle | Surface Free Energy |
---|---|---|
OPS | 105° | 18.5 mN/m |
Octamethylcyclotetrasiloxane | 95° | 22.1 mN/m |
Octakis(dimethylsiloxy)-T8-silsesquioxane | 98° | 20.3 mN/m |
OPS outperforms methyl- and hydride-substituted analogs due to aromatic phenyl groups, which reduce polar interactions .
Key Advantages and Limitations
- OPS: Advantages: Exceptional thermal robustness, compatibility with aromatic polymers, and tunable porosity . Limitations: Poor solubility in polar solvents (e.g., methanol, acetone) .
- Octa(aminophenyl)silsesquioxane: Advantages: Reactive amino groups enable covalent integration into polymer networks . Limitations: Lower thermal stability compared to OPS.
- Octamethylcyclotetrasiloxane: Advantages: Low cost, high purity in silicone production . Limitations: Limited mechanical reinforcement capability.
Biologische Aktivität
Octaphenylsilsesquioxane (OPS) is a member of the polyhedral oligomeric silsesquioxanes (POSS) family, characterized by a cage-like structure composed of silicon and oxygen atoms with phenyl groups at the corners. This unique architecture imparts distinctive properties, making OPS a subject of interest in various fields, including materials science and biomedical applications. This article explores the biological activity of OPS, highlighting its synthesis, properties, and potential applications.
Synthesis and Structural Characteristics
OPS can be synthesized through several methods, including the reaction of silanes with phenolic compounds or via sol-gel processes. The resulting structure is defined by its empirical formula , which indicates eight phenyl groups attached to a silicon-oxygen framework. The synthesis can be tailored to modify the surface properties and enhance biological compatibility.
Biocompatibility
Research indicates that OPS exhibits high biocompatibility, making it suitable for biomedical applications. Its incorporation into biocompatible polymers has led to advanced nanocomposite materials that show improved hemocompatibility and antithrombogenicity. These characteristics are crucial for cardiovascular implants, where reduced inflammatory responses and calcification resistance are desired .
Antimicrobial Activity
Studies have demonstrated that OPS can possess antimicrobial properties when incorporated into polymer matrices. The phenyl groups can interact with microbial membranes, leading to cell lysis. This property is particularly useful in developing coatings for medical devices to prevent infection .
Drug Delivery Systems
OPS has been explored as a carrier for drug delivery systems due to its tunable porosity and surface chemistry. Research shows that OPS-based materials can encapsulate therapeutic agents and release them in a controlled manner, enhancing the efficacy of treatments while minimizing side effects .
Case Studies
- Cardiovascular Implants : A study evaluated the use of OPS in creating nanocomposites for stents. Results indicated enhanced mechanical properties and reduced thrombogenicity compared to traditional materials, demonstrating OPS's potential in improving implant performance .
- Antimicrobial Coatings : In another case, OPS was integrated into polymer films used for surgical instruments. The films exhibited significant reductions in bacterial colonization compared to uncoated controls, highlighting the effectiveness of OPS in infection prevention .
- Drug Delivery : Research on OPS-based nanoparticles showed successful encapsulation of anticancer drugs with sustained release profiles over extended periods. This application underscores the versatility of OPS in therapeutic contexts .
Data Tables
Property | Value |
---|---|
Empirical Formula | |
Average Molecular Weight | 1,088 g/mol |
Biocompatibility | High |
Antimicrobial Activity | Effective against Gram-positive bacteria |
Drug Loading Capacity | Up to 30% w/w |
Q & A
Basic Research Questions
Q. What are the key considerations for optimizing the synthesis of octaphenylsilsesquioxane (OPS) to achieve high yield and purity?
- Methodological Answer : The hydrolysis of phenyltrichlorosilane under controlled water addition rates (e.g., 0.5 mL/min) and reaction temperatures (e.g., 0–5°C) is critical. Trace KOH catalyzes the rearrangement of the prepolymer into OPS, achieving yields >90% . Post-synthesis purification via solvent washing (e.g., ethanol/water mixtures) and recrystallization (e.g., toluene) removes unreacted precursors. Structural validation requires FTIR (Si–O–Si stretching at ~1,100 cm⁻¹), ²⁹Si NMR (resonances at −78 ppm for T³ silicon), and elemental analysis (C, H, Si content) .
Q. How can spectroscopic techniques resolve structural ambiguities in OPS derivatives, such as sulfonated variants?
- Methodological Answer : For sulfonated OPS (SPOSS), FTIR confirms sulfonic acid group incorporation (S=O stretching at 1,030–1,200 cm⁻¹). MALDI-TOF MS differentiates between SPOSS-2, SPOSS-4, and SPOSS-8 based on molecular weight shifts (e.g., SPOSS-8 at m/z ~1,200–1,300). NMR (¹H and ²⁹Si) identifies regioselective sulfonation patterns and quantifies functional group density .
Q. What thermal stability benchmarks are established for OPS, and how do they compare to modified derivatives?
- Methodological Answer : OPS exhibits a 5% weight loss temperature (Td₅%) of 436.8°C under nitrogen, attributed to its rigid siloxane cage . Sulfonated SPOSS derivatives show enhanced thermal stability (Td₅% >450°C) due to crosslinking via sulfonic acid groups, as confirmed by TG-FTIR analysis of decomposition byproducts (e.g., SO₂, CO₂) .
Advanced Research Questions
Q. How can machine learning (ML) guide the synthesis of high-functionality OPS derivatives?
- Methodological Answer : ML algorithms (e.g., random forests, neural networks) predict optimal reaction conditions for sulfonation. For SPOSS-8 synthesis, training on 21 low-functionality (<4 -SO₃H) datasets identifies K₂SO₄ as a critical additive. ML models correlate reagent ratios (e.g., H₂SO₄:K₂SO₄), temperature, and reaction time with functionality, achieving 95% prediction accuracy .
Q. What computational methods validate OPS-based frameworks for gas adsorption applications?
- Methodological Answer : Grand Canonical Monte Carlo (GCMC) simulations model methane/hydrogen adsorption in OPS-based hybrid frameworks. Parameters include Lennard-Jones potentials for gas-cage interactions and pore size distributions (PSD) derived from N₂ adsorption isotherms. Experimental validation uses volumetric gas adsorption analyzers, with selectivity ratios (CH₄/H₂ >3 at 298 K) matching simulated results .
Q. How do structural defects in OPS affect its performance in polymer nanocomposites?
- Methodological Answer : Defect analysis via X-ray diffraction (XRD) and small-angle X-ray scattering (SAXS) quantifies cage imperfections (e.g., incomplete phenyl substitution). In polyamide/OPS composites, defects reduce tensile modulus by 15–20% compared to defect-free OPS. Mitigation strategies include post-synthetic annealing (250°C, 2 h) to promote cage reorganization .
Q. Data Contradictions and Resolution
Q. Why do conflicting reports exist regarding OPS solubility in organic solvents?
- Analysis : Solubility variations arise from differences in cage symmetry and phenyl group orientation. Fully condensed OPS (T₈ symmetry) is insoluble in non-polar solvents (e.g., hexane) but soluble in aromatic solvents (e.g., toluene). Partially condensed derivatives (T₇ or T₆) exhibit higher solubility due to reduced steric hindrance. Solvent selection must align with OPS synthesis batches, verified via dynamic light scattering (DLS) .
Q. How can researchers reconcile discrepancies in OPS thermal degradation mechanisms?
- Analysis : TG-FTIR studies under oxidative vs. inert atmospheres reveal divergent pathways. In air, phenyl group oxidation dominates (CO₂ release at 500–600°C), while N₂ environments favor siloxane backbone breakdown (Si–O bond scission above 700°C). Standardizing degradation protocols (heating rate, gas flow) minimizes data variability .
Q. Methodological Best Practices
Q. What strategies ensure reproducibility in OPS synthesis across laboratories?
- Guidelines :
- Standardize precursor purity (phenyltrichlorosilane ≥99%).
- Control hydrolysis pH (8–10) using buffered KOH solutions.
- Document reaction parameters (e.g., stirring rate, humidity) in metadata .
Q. How should researchers address batch-to-batch variability in OPS characterization?
Eigenschaften
IUPAC Name |
1,3,5,7,9,11,13,15-octakis-phenyl-2,4,6,8,10,12,14,16,17,18,19,20-dodecaoxa-1,3,5,7,9,11,13,15-octasilapentacyclo[9.5.1.13,9.15,15.17,13]icosane | |
---|---|---|
Source | PubChem | |
URL | https://pubchem.ncbi.nlm.nih.gov | |
Description | Data deposited in or computed by PubChem | |
InChI |
InChI=1S/C48H40O12Si8/c1-9-25-41(26-10-1)61-49-62(42-27-11-2-12-28-42)52-65(45-33-17-5-18-34-45)54-63(50-61,43-29-13-3-14-30-43)56-67(47-37-21-7-22-38-47)57-64(51-61,44-31-15-4-16-32-44)55-66(53-62,46-35-19-6-20-36-46)59-68(58-65,60-67)48-39-23-8-24-40-48/h1-40H | |
Source | PubChem | |
URL | https://pubchem.ncbi.nlm.nih.gov | |
Description | Data deposited in or computed by PubChem | |
InChI Key |
KBXJHRABGYYAFC-UHFFFAOYSA-N | |
Source | PubChem | |
URL | https://pubchem.ncbi.nlm.nih.gov | |
Description | Data deposited in or computed by PubChem | |
Canonical SMILES |
C1=CC=C(C=C1)[Si]23O[Si]4(O[Si]5(O[Si](O2)(O[Si]6(O[Si](O3)(O[Si](O4)(O[Si](O5)(O6)C7=CC=CC=C7)C8=CC=CC=C8)C9=CC=CC=C9)C1=CC=CC=C1)C1=CC=CC=C1)C1=CC=CC=C1)C1=CC=CC=C1 | |
Source | PubChem | |
URL | https://pubchem.ncbi.nlm.nih.gov | |
Description | Data deposited in or computed by PubChem | |
Molecular Formula |
C48H40O12Si8 | |
Source | PubChem | |
URL | https://pubchem.ncbi.nlm.nih.gov | |
Description | Data deposited in or computed by PubChem | |
DSSTOX Substance ID |
DTXSID00893550 | |
Record name | Octaphenylhexacyclooctasiloxane | |
Source | EPA DSSTox | |
URL | https://comptox.epa.gov/dashboard/DTXSID00893550 | |
Description | DSSTox provides a high quality public chemistry resource for supporting improved predictive toxicology. | |
Molecular Weight |
1033.5 g/mol | |
Source | PubChem | |
URL | https://pubchem.ncbi.nlm.nih.gov | |
Description | Data deposited in or computed by PubChem | |
CAS No. |
5256-79-1 | |
Record name | Octaphenylsilsesquioxane | |
Source | CAS Common Chemistry | |
URL | https://commonchemistry.cas.org/detail?cas_rn=5256-79-1 | |
Description | CAS Common Chemistry is an open community resource for accessing chemical information. Nearly 500,000 chemical substances from CAS REGISTRY cover areas of community interest, including common and frequently regulated chemicals, and those relevant to high school and undergraduate chemistry classes. This chemical information, curated by our expert scientists, is provided in alignment with our mission as a division of the American Chemical Society. | |
Explanation | The data from CAS Common Chemistry is provided under a CC-BY-NC 4.0 license, unless otherwise stated. | |
Record name | Pentacyclo(9.5.1.13,9.15,15.17,13)octasiloxane, 1,3,5,7,9,11,13,15-octaphenyl- | |
Source | ChemIDplus | |
URL | https://pubchem.ncbi.nlm.nih.gov/substance/?source=chemidplus&sourceid=0005256791 | |
Description | ChemIDplus is a free, web search system that provides access to the structure and nomenclature authority files used for the identification of chemical substances cited in National Library of Medicine (NLM) databases, including the TOXNET system. | |
Record name | Pentacyclo[9.5.1.13,9.15,15.17,13]octasiloxane, 1,3,5,7,9,11,13,15-octaphenyl- | |
Source | EPA Chemicals under the TSCA | |
URL | https://www.epa.gov/chemicals-under-tsca | |
Description | EPA Chemicals under the Toxic Substances Control Act (TSCA) collection contains information on chemicals and their regulations under TSCA, including non-confidential content from the TSCA Chemical Substance Inventory and Chemical Data Reporting. | |
Record name | Octaphenylhexacyclooctasiloxane | |
Source | EPA DSSTox | |
URL | https://comptox.epa.gov/dashboard/DTXSID00893550 | |
Description | DSSTox provides a high quality public chemistry resource for supporting improved predictive toxicology. | |
Retrosynthesis Analysis
AI-Powered Synthesis Planning: Our tool employs the Template_relevance Pistachio, Template_relevance Bkms_metabolic, Template_relevance Pistachio_ringbreaker, Template_relevance Reaxys, Template_relevance Reaxys_biocatalysis model, leveraging a vast database of chemical reactions to predict feasible synthetic routes.
One-Step Synthesis Focus: Specifically designed for one-step synthesis, it provides concise and direct routes for your target compounds, streamlining the synthesis process.
Accurate Predictions: Utilizing the extensive PISTACHIO, BKMS_METABOLIC, PISTACHIO_RINGBREAKER, REAXYS, REAXYS_BIOCATALYSIS database, our tool offers high-accuracy predictions, reflecting the latest in chemical research and data.
Strategy Settings
Precursor scoring | Relevance Heuristic |
---|---|
Min. plausibility | 0.01 |
Model | Template_relevance |
Template Set | Pistachio/Bkms_metabolic/Pistachio_ringbreaker/Reaxys/Reaxys_biocatalysis |
Top-N result to add to graph | 6 |
Feasible Synthetic Routes
Haftungsausschluss und Informationen zu In-Vitro-Forschungsprodukten
Bitte beachten Sie, dass alle Artikel und Produktinformationen, die auf BenchChem präsentiert werden, ausschließlich zu Informationszwecken bestimmt sind. Die auf BenchChem zum Kauf angebotenen Produkte sind speziell für In-vitro-Studien konzipiert, die außerhalb lebender Organismen durchgeführt werden. In-vitro-Studien, abgeleitet von dem lateinischen Begriff "in Glas", beinhalten Experimente, die in kontrollierten Laborumgebungen unter Verwendung von Zellen oder Geweben durchgeführt werden. Es ist wichtig zu beachten, dass diese Produkte nicht als Arzneimittel oder Medikamente eingestuft sind und keine Zulassung der FDA für die Vorbeugung, Behandlung oder Heilung von medizinischen Zuständen, Beschwerden oder Krankheiten erhalten haben. Wir müssen betonen, dass jede Form der körperlichen Einführung dieser Produkte in Menschen oder Tiere gesetzlich strikt untersagt ist. Es ist unerlässlich, sich an diese Richtlinien zu halten, um die Einhaltung rechtlicher und ethischer Standards in Forschung und Experiment zu gewährleisten.