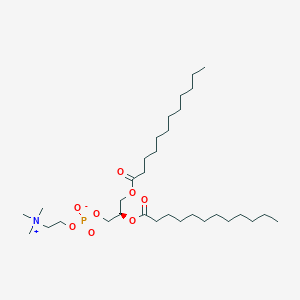
1,2-Dilauroyl-sn-glycero-3-phosphocholine
Übersicht
Beschreibung
1,2-Dilauroyl-sn-glycero-3-Phosphocholin, allgemein als DLPC bezeichnet, ist ein Phospholipid, das zur Klasse der Glycerophosphocholine gehört. Es besteht aus einem Glycerin-Rückgrat, zwei Laurinsäure-(Dodecansäure-)Ketten und einer Phosphocholin-Kopfgruppe. DLPC ist bekannt für seine Rolle bei der Bildung von Lipiddoppelschichten und wird in der biochemischen und biophysikalischen Forschung häufig eingesetzt.
Wirkmechanismus
Target of Action
DLPC, also known as 1,2-Dilauroyl-sn-glycero-3-phosphocholine, is primarily an agonist ligand for the Liver Receptor Homolog-1 (LRH-1) . LRH-1 is a nuclear receptor that plays a crucial role in regulating gene expression involved in cholesterol and bile acid metabolism, as well as in reproductive processes .
Mode of Action
As an LRH-1 agonist, DLPC binds to the LRH-1 receptor, activating it . This activation leads to changes in the transcription of genes regulated by LRH-1, thereby influencing various metabolic processes .
Biochemical Pathways
The activation of LRH-1 by DLPC impacts several biochemical pathways. One of the key pathways influenced is the phosphatidylcholine pathway, which plays a significant role in lipid metabolism . The activation of LRH-1 can lead to enhanced insulin sensitivity and lipolysis, which are crucial for managing obesity .
Pharmacokinetics
Dlpc is a phospholipid and is commonly used in the generation of micelles and liposomes , which are known to enhance the bioavailability of drugs.
Result of Action
The activation of LRH-1 by DLPC can lead to enhanced insulin sensitivity and increased lipolysis in adipose tissue . This can potentially help in managing conditions like obesity and type 2 diabetes .
Action Environment
The action of DLPC can be influenced by various environmental factors. For instance, the formation of micelles and liposomes, which enhances the bioavailability of DLPC, can be affected by factors such as temperature and pH . .
Biochemische Analyse
Biochemical Properties
DLPC plays a significant role in biochemical reactions. It interacts with various enzymes, proteins, and other biomolecules. For instance, DLPC is an LRH-1 agonist ligand . The nature of these interactions is often determined by the specific biochemical context in which they occur.
Cellular Effects
DLPC has profound effects on various types of cells and cellular processes. It influences cell function, including impacts on cell signaling pathways, gene expression, and cellular metabolism . For example, DLPC treatment can increase insulin sensitivity in muscle cells treated with palmitate ester .
Molecular Mechanism
At the molecular level, DLPC exerts its effects through various mechanisms. These include binding interactions with biomolecules, enzyme inhibition or activation, and changes in gene expression . For instance, DLPC can increase the expression level of peroxisome proliferator-activated receptor alpha (PPARα) in C2C12 myocytes .
Temporal Effects in Laboratory Settings
Over time in laboratory settings, the effects of DLPC can change. This includes information on the product’s stability, degradation, and any long-term effects on cellular function observed in in vitro or in vivo studies .
Dosage Effects in Animal Models
The effects of DLPC can vary with different dosages in animal models. This could include any threshold effects observed in these studies, as well as any toxic or adverse effects at high doses .
Metabolic Pathways
DLPC is involved in various metabolic pathways. It interacts with enzymes or cofactors, and can also affect metabolic flux or metabolite levels .
Transport and Distribution
DLPC is transported and distributed within cells and tissues. This could include any transporters or binding proteins that it interacts with, as well as any effects on its localization or accumulation .
Subcellular Localization
The subcellular localization of DLPC and any effects on its activity or function could include any targeting signals or post-translational modifications that direct it to specific compartments or organelles .
Vorbereitungsmethoden
Synthetische Wege und Reaktionsbedingungen
DLPC kann durch Veresterung von Glycerin mit Laurinsäure, gefolgt von der Phosphorylierung des resultierenden Diglycerids mit Phosphocholin, synthetisiert werden. Die Reaktion beinhaltet typischerweise die Verwendung von Katalysatoren und spezifischen Reaktionsbedingungen, um eine hohe Ausbeute und Reinheit zu gewährleisten. Der Prozess kann wie folgt zusammengefasst werden:
Veresterung: Glycerin reagiert mit Laurinsäure in Gegenwart eines Katalysators wie Schwefelsäure zu 1,2-Dilauroyl-sn-glycerol.
Phosphorylierung: Das Diglycerid wird dann mit Phosphocholinchlorid in Gegenwart einer Base wie Triethylamin phosphoryliert, um DLPC zu erhalten.
Industrielle Produktionsverfahren
Die industrielle Produktion von DLPC beinhaltet ähnliche Synthesewege, jedoch in größerem Maßstab. Der Prozess ist auf Effizienz, Ausbeute und Reinheit optimiert. Hochleistungsflüssigchromatographie (HPLC) wird häufig verwendet, um das Endprodukt zu reinigen und sicherzustellen, dass es die erforderlichen Standards für pharmazeutische und Forschungsanwendungen erfüllt .
Chemische Reaktionsanalyse
Arten von Reaktionen
DLPC unterliegt verschiedenen chemischen Reaktionen, darunter:
Oxidation: DLPC kann, insbesondere an den ungesättigten Fettsäureketten, oxidiert werden, was zur Bildung von Peroxiden und anderen Oxidationsprodukten führt.
Hydrolyse: Die Esterbindungen in DLPC können durch Enzyme wie Phospholipasen hydrolysiert werden, was zur Freisetzung von Laurinsäure und Glycerophosphocholin führt.
Substitution: DLPC kann an Substitutionsreaktionen teilnehmen, bei denen die Phosphocholin-Kopfgruppe durch andere funktionelle Gruppen ersetzt wird.
Häufige Reagenzien und Bedingungen
Oxidation: Reagenzien wie Wasserstoffperoxid oder molekularer Sauerstoff in Gegenwart von Katalysatoren.
Hydrolyse: Enzyme wie Phospholipase A2 unter physiologischen Bedingungen.
Substitution: Verschiedene Nucleophile unter kontrollierten pH- und Temperaturbedingungen.
Hauptprodukte
Oxidation: Peroxide und Aldehyde.
Hydrolyse: Laurinsäure und Glycerophosphocholin.
Substitution: Modifizierte Phospholipide mit unterschiedlichen Kopfgruppen
Wissenschaftliche Forschungsanwendungen
DLPC hat eine breite Palette von Anwendungen in der wissenschaftlichen Forschung:
Chemie: Wird als Standard in der Massenspektrometrie zur Quantifizierung von Phospholipiden verwendet.
Biologie: Wird hinsichtlich seiner Rolle in der Zellmembranstruktur und -funktion untersucht. Es wird auch zur Herstellung von Liposomen für die Wirkstoffabgabe verwendet.
Medizin: Wird auf sein Potenzial zur Behandlung von Erkrankungen wie Fettembolie und zur Senkung von Cholesterin- und Triglyceridspiegeln untersucht.
Industrie: Wird aufgrund seiner Biokompatibilität und seiner oberflächenaktiven Eigenschaften in der Formulierung von Kosmetika und Arzneimitteln eingesetzt
Wirkmechanismus
DLPC entfaltet seine Wirkungen hauptsächlich durch seine Einarbeitung in Zellmembranen, wo es die Membranfluidität und -permeabilität beeinflusst. Es kann auch als Signalmolekül fungieren und verschiedene zelluläre Signalwege modulieren. So konnte gezeigt werden, dass DLPC die Braunisierung von Fettgewebe durch Lipidperoxidation vermittelte p38-Signalübertragung verstärkt, was Auswirkungen auf die Behandlung von Fettleibigkeit haben kann .
Analyse Chemischer Reaktionen
Types of Reactions
DLPC undergoes various chemical reactions, including:
Oxidation: DLPC can undergo oxidation, particularly at the unsaturated fatty acid chains, leading to the formation of peroxides and other oxidative products.
Hydrolysis: The ester bonds in DLPC can be hydrolyzed by enzymes such as phospholipases, resulting in the release of lauric acid and glycerophosphocholine.
Substitution: DLPC can participate in substitution reactions where the phosphocholine head group is replaced by other functional groups.
Common Reagents and Conditions
Oxidation: Reagents such as hydrogen peroxide or molecular oxygen in the presence of catalysts.
Hydrolysis: Enzymes like phospholipase A2 under physiological conditions.
Substitution: Various nucleophiles under controlled pH and temperature conditions.
Major Products
Oxidation: Peroxides and aldehydes.
Hydrolysis: Lauric acid and glycerophosphocholine.
Substitution: Modified phospholipids with different head groups
Wissenschaftliche Forschungsanwendungen
DLPC has a wide range of applications in scientific research:
Chemistry: Used as a standard in mass spectrometry for the quantification of phospholipids.
Biology: Studied for its role in cell membrane structure and function. It is also used in the preparation of liposomes for drug delivery.
Medicine: Investigated for its potential in treating conditions like fat embolism and lowering cholesterol and triglyceride levels.
Industry: Utilized in the formulation of cosmetics and pharmaceuticals due to its biocompatibility and surfactant properties
Vergleich Mit ähnlichen Verbindungen
DLPC wird oft mit anderen Phosphatidylcholinen verglichen, wie z. B.:
1,2-Dimyristoyl-sn-glycero-3-Phosphocholin (DMPC): Ähnlich in der Struktur, jedoch mit Myristinsäureketten anstelle von Laurinsäure.
1,2-Dipalmitoyl-sn-glycero-3-Phosphocholin (DPPC): Enthält Palmitinsäureketten und hat eine höhere Schmelztemperatur.
1,2-Distearoyl-sn-glycero-3-Phosphocholin (DSPC): Besteht aus Stearinsäureketten und ist bei höheren Temperaturen stabiler.
DLPC ist aufgrund seiner kürzeren Laurinsäureketten einzigartig, was zu einer niedrigeren Schmelztemperatur und anderen biophysikalischen Eigenschaften im Vergleich zu seinen längerkettigen Gegenstücken führt .
Eigenschaften
IUPAC Name |
[(2R)-2,3-di(dodecanoyloxy)propyl] 2-(trimethylazaniumyl)ethyl phosphate | |
---|---|---|
Source | PubChem | |
URL | https://pubchem.ncbi.nlm.nih.gov | |
Description | Data deposited in or computed by PubChem | |
InChI |
InChI=1S/C32H64NO8P/c1-6-8-10-12-14-16-18-20-22-24-31(34)38-28-30(29-40-42(36,37)39-27-26-33(3,4)5)41-32(35)25-23-21-19-17-15-13-11-9-7-2/h30H,6-29H2,1-5H3/t30-/m1/s1 | |
Source | PubChem | |
URL | https://pubchem.ncbi.nlm.nih.gov | |
Description | Data deposited in or computed by PubChem | |
InChI Key |
IJFVSSZAOYLHEE-SSEXGKCCSA-N | |
Source | PubChem | |
URL | https://pubchem.ncbi.nlm.nih.gov | |
Description | Data deposited in or computed by PubChem | |
Canonical SMILES |
CCCCCCCCCCCC(=O)OCC(COP(=O)([O-])OCC[N+](C)(C)C)OC(=O)CCCCCCCCCCC | |
Source | PubChem | |
URL | https://pubchem.ncbi.nlm.nih.gov | |
Description | Data deposited in or computed by PubChem | |
Isomeric SMILES |
CCCCCCCCCCCC(=O)OC[C@H](COP(=O)([O-])OCC[N+](C)(C)C)OC(=O)CCCCCCCCCCC | |
Source | PubChem | |
URL | https://pubchem.ncbi.nlm.nih.gov | |
Description | Data deposited in or computed by PubChem | |
Molecular Formula |
C32H64NO8P | |
Source | PubChem | |
URL | https://pubchem.ncbi.nlm.nih.gov | |
Description | Data deposited in or computed by PubChem | |
DSSTOX Substance ID |
DTXSID10904060 | |
Record name | Dilauroyl phosphatidylcholine | |
Source | EPA DSSTox | |
URL | https://comptox.epa.gov/dashboard/DTXSID10904060 | |
Description | DSSTox provides a high quality public chemistry resource for supporting improved predictive toxicology. | |
Molecular Weight |
621.8 g/mol | |
Source | PubChem | |
URL | https://pubchem.ncbi.nlm.nih.gov | |
Description | Data deposited in or computed by PubChem | |
CAS No. |
18194-25-7 | |
Record name | 1,2-Dilauroyl-sn-glycero-3-phosphocholine | |
Source | CAS Common Chemistry | |
URL | https://commonchemistry.cas.org/detail?cas_rn=18194-25-7 | |
Description | CAS Common Chemistry is an open community resource for accessing chemical information. Nearly 500,000 chemical substances from CAS REGISTRY cover areas of community interest, including common and frequently regulated chemicals, and those relevant to high school and undergraduate chemistry classes. This chemical information, curated by our expert scientists, is provided in alignment with our mission as a division of the American Chemical Society. | |
Explanation | The data from CAS Common Chemistry is provided under a CC-BY-NC 4.0 license, unless otherwise stated. | |
Record name | L-alpha-Dilauroyl-sn-glycero-3-phosphocholine | |
Source | ChemIDplus | |
URL | https://pubchem.ncbi.nlm.nih.gov/substance/?source=chemidplus&sourceid=0018194257 | |
Description | ChemIDplus is a free, web search system that provides access to the structure and nomenclature authority files used for the identification of chemical substances cited in National Library of Medicine (NLM) databases, including the TOXNET system. | |
Record name | Dilauroyl phosphatidylcholine | |
Source | EPA DSSTox | |
URL | https://comptox.epa.gov/dashboard/DTXSID10904060 | |
Description | DSSTox provides a high quality public chemistry resource for supporting improved predictive toxicology. | |
Record name | (R)-(7-lauroyl-4-oxido-10-oxo-3,5,9-trioxa-4-phosphahenicosyl)trimethylammonium 4-oxide | |
Source | European Chemicals Agency (ECHA) | |
URL | https://echa.europa.eu/substance-information/-/substanceinfo/100.038.246 | |
Description | The European Chemicals Agency (ECHA) is an agency of the European Union which is the driving force among regulatory authorities in implementing the EU's groundbreaking chemicals legislation for the benefit of human health and the environment as well as for innovation and competitiveness. | |
Explanation | Use of the information, documents and data from the ECHA website is subject to the terms and conditions of this Legal Notice, and subject to other binding limitations provided for under applicable law, the information, documents and data made available on the ECHA website may be reproduced, distributed and/or used, totally or in part, for non-commercial purposes provided that ECHA is acknowledged as the source: "Source: European Chemicals Agency, http://echa.europa.eu/". Such acknowledgement must be included in each copy of the material. ECHA permits and encourages organisations and individuals to create links to the ECHA website under the following cumulative conditions: Links can only be made to webpages that provide a link to the Legal Notice page. | |
Record name | 1,2-DILAUROYL-SN-GLYCERO-3-PHOSPHOCHOLINE | |
Source | FDA Global Substance Registration System (GSRS) | |
URL | https://gsrs.ncats.nih.gov/ginas/app/beta/substances/31NE1DVE91 | |
Description | The FDA Global Substance Registration System (GSRS) enables the efficient and accurate exchange of information on what substances are in regulated products. Instead of relying on names, which vary across regulatory domains, countries, and regions, the GSRS knowledge base makes it possible for substances to be defined by standardized, scientific descriptions. | |
Explanation | Unless otherwise noted, the contents of the FDA website (www.fda.gov), both text and graphics, are not copyrighted. They are in the public domain and may be republished, reprinted and otherwise used freely by anyone without the need to obtain permission from FDA. Credit to the U.S. Food and Drug Administration as the source is appreciated but not required. | |
Retrosynthesis Analysis
AI-Powered Synthesis Planning: Our tool employs the Template_relevance Pistachio, Template_relevance Bkms_metabolic, Template_relevance Pistachio_ringbreaker, Template_relevance Reaxys, Template_relevance Reaxys_biocatalysis model, leveraging a vast database of chemical reactions to predict feasible synthetic routes.
One-Step Synthesis Focus: Specifically designed for one-step synthesis, it provides concise and direct routes for your target compounds, streamlining the synthesis process.
Accurate Predictions: Utilizing the extensive PISTACHIO, BKMS_METABOLIC, PISTACHIO_RINGBREAKER, REAXYS, REAXYS_BIOCATALYSIS database, our tool offers high-accuracy predictions, reflecting the latest in chemical research and data.
Strategy Settings
Precursor scoring | Relevance Heuristic |
---|---|
Min. plausibility | 0.01 |
Model | Template_relevance |
Template Set | Pistachio/Bkms_metabolic/Pistachio_ringbreaker/Reaxys/Reaxys_biocatalysis |
Top-N result to add to graph | 6 |
Feasible Synthetic Routes
Haftungsausschluss und Informationen zu In-Vitro-Forschungsprodukten
Bitte beachten Sie, dass alle Artikel und Produktinformationen, die auf BenchChem präsentiert werden, ausschließlich zu Informationszwecken bestimmt sind. Die auf BenchChem zum Kauf angebotenen Produkte sind speziell für In-vitro-Studien konzipiert, die außerhalb lebender Organismen durchgeführt werden. In-vitro-Studien, abgeleitet von dem lateinischen Begriff "in Glas", beinhalten Experimente, die in kontrollierten Laborumgebungen unter Verwendung von Zellen oder Geweben durchgeführt werden. Es ist wichtig zu beachten, dass diese Produkte nicht als Arzneimittel oder Medikamente eingestuft sind und keine Zulassung der FDA für die Vorbeugung, Behandlung oder Heilung von medizinischen Zuständen, Beschwerden oder Krankheiten erhalten haben. Wir müssen betonen, dass jede Form der körperlichen Einführung dieser Produkte in Menschen oder Tiere gesetzlich strikt untersagt ist. Es ist unerlässlich, sich an diese Richtlinien zu halten, um die Einhaltung rechtlicher und ethischer Standards in Forschung und Experiment zu gewährleisten.