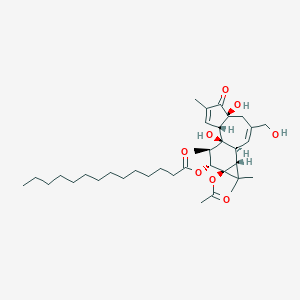
4α-Phorbol-12-myristat-13-acetat
Übersicht
Beschreibung
Phorbol 12-myristate 13-acetate (PMA; ) is a phorbol ester that is commonly used to activate certain types of PKC, including group A (α, βI, βII, γ) and group B (δ, ε, η, θ) isoforms. 4α-PMA is a structural analog of PMA that does not activate PKC. It is used as a negative control for PMA signaling through PKC.
Wissenschaftliche Forschungsanwendungen
Immunologie
4α-TPA: wurde gezeigt, dass es die Differenzierung von voraktivierten B-Zellen zu regulatorischen B-Zellen mit einem Granzym B+-Phänotyp verstärkt, was für Autoimmun- und Hyperentzündungszustände von Bedeutung sein könnte . Diese Verbindung wirkt als ein starker Enhancer der IL-21-induzierten Differenzierung, was ihr Potenzial als sichere Alternative für die Ex-vivo-Herstellung von regulatorischen B-Zellen nahelegt .
Neurowissenschaften
In der Neurowissenschaft wird 4α-TPA als negative Kontrolle für die Aktivierung der Proteinkinase C (PKC) durch Phorbolester verwendet, was entscheidend ist, um neuronale Signalwege zu verstehen . Es wurde auch an der Induktion von Nervenwachstumsfaktor beteiligt, der für das Wachstum und Überleben von Neuronen unerlässlich ist .
Dermatologie
4α-TPA: dient als negative Kontrolle in der dermatologischen Forschung, insbesondere in Studien, die die PKC-Aktivierung betreffen. PKC spielt eine Rolle bei verschiedenen Hauterkrankungen, und 4α-TPA hilft bei der Analyse der beteiligten Signalwege .
Pharmakologie
In pharmakologischen Studien wird 4α-TPA verwendet, um die Aktivierung von TRPV4-Kanälen zu verstehen und als Werkzeug für Struktur-Aktivitäts-Untersuchungen . Es liefert Einblicke in die molekularen Wechselwirkungen und die Auswirkungen von Verbindungen auf diese Ionenkanäle.
Biochemie
4α-TPA: wird in der biochemischen Forschung verwendet, um die Aktivierung von PKC-Isoformen und ihre Rolle in zellulären Prozessen wie Apoptose und Zellproliferation zu untersuchen . Es hilft bei der Aufklärung der biochemischen Pfade, die diesen grundlegenden Zellfunktionen zugrunde liegen.
Toxikologie
Bei toxikologischen Beurteilungen ist 4α-TPA eine Referenzverbindung, die zur Bewertung der nicht-tumorfördernden Aktivitäten von Phorbolestern verwendet wird . Es hilft beim Verständnis der toxikologischen Profile verwandter Verbindungen.
Immunologie - Makrophagen-Differenzierung
4α-TPA: wird in immunologischen Studien häufig verwendet, um THP-1-Monozyten zu Makrophagen zu differenzieren, wodurch ein Modell für die Untersuchung von Immunantworten bereitgestellt wird . Es ist entscheidend für die Untersuchung der
Wirkmechanismus
Target of Action
The primary target of 4alpha-Phorbol 12-myristate 13-acetate (4|A-TPA) is Protein Kinase C (PKC) . PKC is a family of protein kinase enzymes that are involved in controlling the function of other proteins through the phosphorylation of hydroxyl groups of serine and threonine amino acid residues .
Mode of Action
4|A-TPA binds to the C1 domain of PKC , inducing membrane translocation and enzyme activation . This interaction results in the activation of certain types of PKC, including group A (α, βI, βII, γ) and group B (δ, ε, η, θ) isoforms .
Biochemical Pathways
The activation of PKC by 4|A-TPA affects various biochemical pathways. For instance, it has been shown to enhance IL-21-induced differentiation of pre-activated B cells into GrB+ regulatory B cells . It also regulates various processes such as gene transcription, cellular growth and differentiation, apoptosis, the immune response, and receptor desensitization .
Pharmacokinetics
It’s known that the compound is soluble in dmso, ethanol, and methanol , which could influence its bioavailability.
Result of Action
The activation of PKC by 4|A-TPA leads to various molecular and cellular effects. For example, it has been shown to enhance the differentiation of pre-activated B cells into GrB+ regulatory B cells . These cells are characterized by the secretion of enzymatically active granzyme B and play a role in immune regulation .
Action Environment
The action, efficacy, and stability of 4|A-TPA can be influenced by various environmental factors. It’s worth noting that the compound has undergone clinical testing in patients with certain hematological diseases, suggesting its potential for therapeutic use .
Safety and Hazards
Zukünftige Richtungen
Research on 4α-PMA is ongoing, and it continues to be a useful tool for structure-activity investigations . For instance, it has been shown to be a reasonably potent activator of TRPV4 channels . Furthermore, this compound has also been shown to increase PGHS-2 mRNA significantly in murine keratinocytes .
Biochemische Analyse
Biochemical Properties
4|A-TPA is known to interact with certain types of PKC, including group A (α, βI, βII, γ) and group B (δ, ε, η, θ) isoforms . Unlike PMA, 4|A-TPA does not activate these PKC isoforms . The nature of these interactions is primarily inhibitory, as 4|A-TPA is used to suppress the activation of PKC .
Cellular Effects
The effects of 4|A-TPA on cells are primarily observed in the context of its role as a negative control for PMA signaling through PKC . For instance, in osteoblasts, treatment with PMA, which activates PKC, suppresses the expression of the Nephronectin gene. This suppression can be inhibited by the PKC signal inhibitor Gö6983 and by transfection with small interfering RNA (siRNA) of PKCα, c-Jun, and c-Fos .
Molecular Mechanism
The molecular mechanism of 4|A-TPA primarily involves its interaction with PKC isoforms . As a structural analog of PMA, 4|A-TPA binds to the same sites on PKC as PMA but does not activate the enzyme . This results in the inhibition of PKC activation and downstream signaling .
Temporal Effects in Laboratory Settings
The effects of 4|A-TPA over time in laboratory settings are primarily observed in the context of its role as a negative control for PMA signaling through PKC . For instance, in osteoblasts, the suppression of Nephronectin gene expression by PMA was noted to be time- and dose-dependent .
Metabolic Pathways
Given its structural similarity to PMA, it may be involved in similar pathways, particularly those involving PKC .
Eigenschaften
IUPAC Name |
[(1S,2S,6S,10S,11R,13S,14R,15R)-13-acetyloxy-1,6-dihydroxy-8-(hydroxymethyl)-4,12,12,15-tetramethyl-5-oxo-14-tetracyclo[8.5.0.02,6.011,13]pentadeca-3,8-dienyl] tetradecanoate | |
---|---|---|
Source | PubChem | |
URL | https://pubchem.ncbi.nlm.nih.gov | |
Description | Data deposited in or computed by PubChem | |
InChI |
InChI=1S/C36H56O8/c1-7-8-9-10-11-12-13-14-15-16-17-18-29(39)43-32-24(3)35(42)27(30-33(5,6)36(30,32)44-25(4)38)20-26(22-37)21-34(41)28(35)19-23(2)31(34)40/h19-20,24,27-28,30,32,37,41-42H,7-18,21-22H2,1-6H3/t24-,27+,28-,30-,32-,34+,35-,36-/m1/s1 | |
Source | PubChem | |
URL | https://pubchem.ncbi.nlm.nih.gov | |
Description | Data deposited in or computed by PubChem | |
InChI Key |
PHEDXBVPIONUQT-LQLWEASQSA-N | |
Source | PubChem | |
URL | https://pubchem.ncbi.nlm.nih.gov | |
Description | Data deposited in or computed by PubChem | |
Canonical SMILES |
CCCCCCCCCCCCCC(=O)OC1C(C2(C(C=C(CC3(C2C=C(C3=O)C)O)CO)C4C1(C4(C)C)OC(=O)C)O)C | |
Source | PubChem | |
URL | https://pubchem.ncbi.nlm.nih.gov | |
Description | Data deposited in or computed by PubChem | |
Isomeric SMILES |
CCCCCCCCCCCCCC(=O)O[C@@H]1[C@H]([C@]2([C@@H](C=C(C[C@@]3([C@H]2C=C(C3=O)C)O)CO)[C@H]4[C@@]1(C4(C)C)OC(=O)C)O)C | |
Source | PubChem | |
URL | https://pubchem.ncbi.nlm.nih.gov | |
Description | Data deposited in or computed by PubChem | |
Molecular Formula |
C36H56O8 | |
Source | PubChem | |
URL | https://pubchem.ncbi.nlm.nih.gov | |
Description | Data deposited in or computed by PubChem | |
Molecular Weight |
616.8 g/mol | |
Source | PubChem | |
URL | https://pubchem.ncbi.nlm.nih.gov | |
Description | Data deposited in or computed by PubChem | |
CAS No. |
63597-44-4 | |
Record name | (1aR,1bS,4aS,7aS,7bS,8R,9R,9aS)-9a-(Acetyloxy)-1a,1b,4,4a,5,7a,7b,8,9,9a-decahydro-4a,7b-dihydroxy-3-(hydroxymethyl)-1,1,6,8-tetramethyl-5-oxo-1H-cyclopropa[3,4]benz[1,2-e]azulen-9-yl tetradecanoate | |
Source | CAS Common Chemistry | |
URL | https://commonchemistry.cas.org/detail?cas_rn=63597-44-4 | |
Description | CAS Common Chemistry is an open community resource for accessing chemical information. Nearly 500,000 chemical substances from CAS REGISTRY cover areas of community interest, including common and frequently regulated chemicals, and those relevant to high school and undergraduate chemistry classes. This chemical information, curated by our expert scientists, is provided in alignment with our mission as a division of the American Chemical Society. | |
Explanation | The data from CAS Common Chemistry is provided under a CC-BY-NC 4.0 license, unless otherwise stated. | |
Record name | 4alpha-Phorbol-12-myristate-13-acetate | |
Source | ChemIDplus | |
URL | https://pubchem.ncbi.nlm.nih.gov/substance/?source=chemidplus&sourceid=0063597444 | |
Description | ChemIDplus is a free, web search system that provides access to the structure and nomenclature authority files used for the identification of chemical substances cited in National Library of Medicine (NLM) databases, including the TOXNET system. | |
Retrosynthesis Analysis
AI-Powered Synthesis Planning: Our tool employs the Template_relevance Pistachio, Template_relevance Bkms_metabolic, Template_relevance Pistachio_ringbreaker, Template_relevance Reaxys, Template_relevance Reaxys_biocatalysis model, leveraging a vast database of chemical reactions to predict feasible synthetic routes.
One-Step Synthesis Focus: Specifically designed for one-step synthesis, it provides concise and direct routes for your target compounds, streamlining the synthesis process.
Accurate Predictions: Utilizing the extensive PISTACHIO, BKMS_METABOLIC, PISTACHIO_RINGBREAKER, REAXYS, REAXYS_BIOCATALYSIS database, our tool offers high-accuracy predictions, reflecting the latest in chemical research and data.
Strategy Settings
Precursor scoring | Relevance Heuristic |
---|---|
Min. plausibility | 0.01 |
Model | Template_relevance |
Template Set | Pistachio/Bkms_metabolic/Pistachio_ringbreaker/Reaxys/Reaxys_biocatalysis |
Top-N result to add to graph | 6 |
Feasible Synthetic Routes
Haftungsausschluss und Informationen zu In-Vitro-Forschungsprodukten
Bitte beachten Sie, dass alle Artikel und Produktinformationen, die auf BenchChem präsentiert werden, ausschließlich zu Informationszwecken bestimmt sind. Die auf BenchChem zum Kauf angebotenen Produkte sind speziell für In-vitro-Studien konzipiert, die außerhalb lebender Organismen durchgeführt werden. In-vitro-Studien, abgeleitet von dem lateinischen Begriff "in Glas", beinhalten Experimente, die in kontrollierten Laborumgebungen unter Verwendung von Zellen oder Geweben durchgeführt werden. Es ist wichtig zu beachten, dass diese Produkte nicht als Arzneimittel oder Medikamente eingestuft sind und keine Zulassung der FDA für die Vorbeugung, Behandlung oder Heilung von medizinischen Zuständen, Beschwerden oder Krankheiten erhalten haben. Wir müssen betonen, dass jede Form der körperlichen Einführung dieser Produkte in Menschen oder Tiere gesetzlich strikt untersagt ist. Es ist unerlässlich, sich an diese Richtlinien zu halten, um die Einhaltung rechtlicher und ethischer Standards in Forschung und Experiment zu gewährleisten.