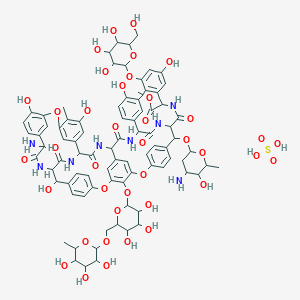
Ristocetin-Sulfat
- Klicken Sie auf QUICK INQUIRY, um ein Angebot von unserem Expertenteam zu erhalten.
- Mit qualitativ hochwertigen Produkten zu einem WETTBEWERBSFÄHIGEN Preis können Sie sich mehr auf Ihre Forschung konzentrieren.
Übersicht
Beschreibung
Ristocetin ist ein Glykopeptid-Antibiotikum, das aus dem Bakterium Amycolatopsis lurida gewonnen wird. Es wurde ursprünglich zur Behandlung von Staphylokokkeninfektionen eingesetzt, wurde aber später aufgrund seiner Nebenwirkungen, wie Thrombozytopenie und Thrombozytenaggregation, eingestellt . Heute wird es hauptsächlich im Labor verwendet, um Erkrankungen wie die von-Willebrand-Krankheit und das Bernard-Soulier-Syndrom zu diagnostizieren .
Herstellungsmethoden
Ristocetin wird durch Fermentation von Nocardia lurida hergestellt. Diese Bestandteile können durch Papierstreifenelektrophorese und Papierchromatographie getrennt werden . Die Verbindungen sind amphoter, können als freie Basen isoliert und als Sulfate kristallisiert werden . Sie sind in sauren wässrigen Lösungen löslich, aber weniger löslich in neutralen wässrigen Lösungen und im Allgemeinen unlöslich in organischen Lösungsmitteln .
Chemische Reaktionsanalyse
Ristocetin unterliegt verschiedenen chemischen Reaktionen, einschließlich Hydrolyse mit Säure, wodurch Zucker und ein Aminofragment abgespalten werden, wodurch seine Aktivität gegen grampositive Bakterien um das 30-fache erhöht wird . Es enthält Amino- und Phenolgruppe und ist in wässrigen sauren Lösungen stabil . Die Abbauprodukte von Ristocetin behalten keine Kreuzresistenzeigenschaften mit den Stammverbindungen .
Wissenschaftliche Forschungsanwendungen
Ristocetin wird häufig in der wissenschaftlichen Forschung verwendet, insbesondere bei der Untersuchung der Thrombozytenfunktion und der Blutgerinnung. Es induziert die Thrombozytenaggregation, indem es den von-Willebrand-Faktor dazu bringt, an den Thrombozytenrezeptor Glykoprotein Ib (GpIb) zu binden . Diese Eigenschaft macht es wertvoll bei der Diagnose von Blutungsstörungen wie der von-Willebrand-Krankheit und dem Bernard-Soulier-Syndrom . Zusätzlich wird Ristocetin verwendet, um die Interaktion von Thrombozyten mit dem von-Willebrand-Faktor zu untersuchen und die Mechanismen der Thrombozytenaggregation zu untersuchen .
Wirkmechanismus
Ristocetin übt seine Wirkung aus, indem es den von-Willebrand-Faktor dazu bringt, an den Thrombozytenrezeptor Glykoprotein Ib (GpIb) zu binden, was zu einer Thrombozytenaggregation führt . Diese Wechselwirkung ist entscheidend für die Anfangsphase der Thrombozytenaggregation und wird verwendet, um die Funktionalität des von-Willebrand-Faktors in Blutproben zu beurteilen . Der genaue molekulare Mechanismus, durch den Ristocetin diese Bindung induziert, ist nicht vollständig geklärt .
Wirkmechanismus
Target of Action
Ristocetin sulfate primarily targets the Von Willebrand Factor (vWF), a large multimeric adhesive glycoprotein that plays a crucial role in thrombosis and hemostasis . It enhances the binding of vWF to a platelet receptor called glycoprotein Ib/IX .
Mode of Action
Ristocetin sulfate promotes platelet aggregation by enhancing the binding of vWF to the platelet receptor glycoprotein Ib/IX . This interaction is crucial for studying platelet function and the biochemical pathways involved in blood coagulation .
Biochemical Pathways
The primary biochemical pathway affected by Ristocetin sulfate is the blood coagulation pathway . By promoting the interaction of vWF with glycoprotein Ib/IX, it supports the formation of a platelet plug under flow conditions . This process is essential for primary hemostasis .
Pharmacokinetics
It is known that the compound is very stable in aqueous acidic solutions . Its activity does not significantly alter over a medium pH of 5.0 to 7.0, but there is a rapid loss of activity above a pH of 7.5 .
Result of Action
The primary result of Ristocetin sulfate’s action is the promotion of platelet aggregation . This is achieved through its interaction with vWF, leading to the formation of a platelet plug that is crucial for blood coagulation . It is worth noting that ristocetin sulfate is no longer used clinically because it caused thrombocytopenia and platelet agglutination .
Action Environment
The action of Ristocetin sulfate is influenced by the pH of its environment . As mentioned earlier, it is stable in aqueous acidic solutions and its activity does not significantly alter over a medium pH of 5.0 to 7.0 . There is a rapid loss of activity above a ph of 75 . This suggests that the efficacy and stability of Ristocetin sulfate are highly dependent on the pH of its environment .
Biochemische Analyse
Biochemical Properties
Ristocetin Sulfate plays a significant role in biochemical reactions, particularly those involving platelet aggregation. It interacts with the von Willebrand factor (vWF) and the platelet receptor glycoprotein Ib (GpIb). The interaction between Ristocetin Sulfate and vWF promotes the binding of vWF to GpIb, leading to platelet aggregation .
Cellular Effects
Ristocetin Sulfate has profound effects on various types of cells, especially platelets. It activates the von Willebrand factor that interacts with glycoprotein (GP) Ib/IX/V, which generates thromboxane A2 via phospholipase A2 activation, resulting in the release of the soluble CD40 ligand (sCD40L) from human platelets . This process influences cell function, including impact on cell signaling pathways, gene expression, and cellular metabolism.
Temporal Effects in Laboratory Settings
In laboratory settings, the effects of Ristocetin Sulfate can change over time. For instance, Ristocetin-induced platelet aggregation (RIPA) is used as an in vitro test to determine the presence and integrity of the platelet glycoprotein (GP) Ibα-V-IX complex and von Willebrand factor (VWF) interaction . This test measures platelet aggregation with the help of von Willebrand factor (vWF) and Ristocetin Sulfate added in a graded fashion .
Dosage Effects in Animal Models
While specific studies on the dosage effects of Ristocetin Sulfate in animal models are limited, it is generally understood that the effects of any compound can vary with different dosages in animal models . This could include any threshold effects observed in these studies, as well as any toxic or adverse effects at high doses.
Metabolic Pathways
It is known that Ristocetin Sulfate activates the von Willebrand factor, which interacts with glycoprotein (GP) Ib/IX/V, leading to the generation of thromboxane A2 via phospholipase A2 activation .
Transport and Distribution
It is known that Ristocetin Sulfate promotes the interaction of vWF with GpIb, leading to platelet aggregation .
Subcellular Localization
It is known that Ristocetin Sulfate promotes the interaction of vWF with GpIb, leading to platelet aggregation . This suggests that Ristocetin Sulfate may be localized in areas where platelet aggregation occurs.
Vorbereitungsmethoden
Ristocetin is produced through the fermentation of Nocardia lurida. These components can be separated using paper strip electrophoresis and paper chromatography . The compounds are amphoteric, can be isolated as free bases, and crystallized as sulfates . They are soluble in acidic aqueous solutions but less soluble in neutral aqueous solutions and generally insoluble in organic solvents .
Analyse Chemischer Reaktionen
Ristocetin undergoes various chemical reactions, including hydrolysis with acid, which splits off sugars and an amino fragment, increasing its activity against Gram-positive bacteria up to 30-fold . It contains amino and phenolic groups and is stable in aqueous acidic solutions . The degradation products of ristocetin do not retain cross-resistance properties with the parent compounds .
Vergleich Mit ähnlichen Verbindungen
Ristocetin ähnelt anderen Glykopeptid-Antibiotika wie Vancomycin und Teicoplanin. Es ist einzigartig in seiner Fähigkeit, die Thrombozytenaggregation durch seine Wechselwirkung mit dem von-Willebrand-Faktor zu induzieren . Im Gegensatz zu Ristocetin werden Vancomycin und Teicoplanin hauptsächlich zur Behandlung bakterieller Infektionen eingesetzt und haben nicht die gleichen diagnostischen Anwendungen . Die vier nichtribosomalen Peptidsynthetase-Proteine im Ristocetin-Gencluster haben eine Domänenorganisation und eine vorhergesagte Adenylierungsdomänenspezifität, die denen der Teicoplanin-nichtribosomalen Peptidsynthetase ähneln .
Biologische Aktivität
Ristocetin sulfate, a glycopeptide antibiotic originally derived from Nocardia lurida, has garnered attention not only for its antibacterial properties but also for its significant role in hematology, particularly in the diagnosis of von Willebrand disease (VWD). This article explores the biological activity of ristocetin sulfate, focusing on its mechanisms, applications in clinical diagnostics, and relevant research findings.
Ristocetin sulfate functions primarily by inducing platelet aggregation through its interaction with von Willebrand factor (VWF) and the glycoprotein Ib (GpIb) complex on platelets. The binding of ristocetin alters the electrostatic forces between VWF and GpIb, facilitating platelet agglutination under high shear conditions typical of capillary circulation. This mechanism is critical for understanding its diagnostic utility in bleeding disorders.
Key Mechanism Highlights:
- Induces Platelet Aggregation : Ristocetin sulfate promotes the binding of VWF to GpIb, crucial for platelet function.
- Diagnostic Tool : It is employed in assays to diagnose various types of VWD by evaluating platelet response to ristocetin.
Clinical Applications
Ristocetin sulfate is predominantly used in laboratory settings to assess platelet function and diagnose bleeding disorders. The most notable application is the Ristocetin-Induced Platelet Aggregation (RIPA) test, which evaluates the ability of VWF to mediate platelet aggregation.
RIPA Test Overview:
- Purpose : To diagnose types 2B and platelet-type von Willebrand disease.
- Methodology : Platelet-rich plasma (PRP) is exposed to varying concentrations of ristocetin, with aggregation measured via light transmission aggregometry.
- Interpretation :
- Enhanced agglutination at low concentrations indicates Type 2B VWD or platelet-type VWD.
- Normal or reduced responses suggest other forms of VWD or intrinsic platelet defects.
Test Type | Description | Clinical Relevance |
---|---|---|
RIPA | Measures platelet agglutination in response to ristocetin | Diagnoses VWD types |
Low-dose RIPA | Uses lower concentrations of ristocetin | Differentiates between VWD types |
Research Findings
Numerous studies have investigated the biological activity and clinical implications of ristocetin sulfate. Below are summarized findings from key research articles:
- Biosynthetic Studies :
- Platelet Aggregation Studies :
- Immunoinhibition Studies :
Case Studies
Several case studies have illustrated the practical applications of ristocetin sulfate in clinical settings:
- Case Study 1 : A patient diagnosed with Type 2B VWD exhibited enhanced platelet agglutination at low doses of ristocetin during a RIPA test, confirming the diagnosis and guiding treatment decisions.
- Case Study 2 : In a cohort study involving patients with intrinsic platelet defects, those who did not respond to ristocetin were further evaluated for underlying conditions, demonstrating the test's utility in differential diagnosis .
Eigenschaften
CAS-Nummer |
11140-99-1 |
---|---|
Molekularformel |
C95H112N8O48S |
Molekulargewicht |
2166.0 g/mol |
IUPAC-Name |
methyl 22-amino-2-(4-amino-5-hydroxy-6-methyloxan-2-yl)oxy-64-[3-[4,5-dihydroxy-6-(hydroxymethyl)-3-(3,4,5-trihydroxyoxan-2-yl)oxyoxan-2-yl]oxy-4,5-dihydroxy-6-[(3,4,5-trihydroxy-6-methyloxan-2-yl)oxymethyl]oxan-2-yl]oxy-18,26,31,44,49-pentahydroxy-30-methyl-21,35,38,54,56,59-hexaoxo-47-[3,4,5-trihydroxy-6-(hydroxymethyl)oxan-2-yl]oxy-7,13,28-trioxa-20,36,39,53,55,58-hexazaundecacyclo[38.14.2.23,6.214,17.219,34.18,12.123,27.129,33.141,45.010,37.046,51]hexahexaconta-3(66),4,6(65),8,10,12(64),14(63),15,17(62),23(61),24,26,29(60),30,32,41(57),42,44,46(51),47,49-henicosaene-52-carboxylate;sulfuric acid |
InChI |
InChI=1S/C95H110N8O44.H2O4S/c1-30-47(109)18-37-20-49(30)139-50-19-35(9-16-46(50)108)59(97)84(125)102-64-68(113)33-5-11-40(12-6-33)137-52-21-38-22-53(81(52)145-95-83(76(121)72(117)56(143-95)29-134-91-78(123)73(118)67(112)32(3)136-91)147-94-82(75(120)71(116)55(27-105)142-94)146-92-77(122)69(114)48(110)28-133-92)138-41-13-7-34(8-14-41)80(144-57-25-44(96)66(111)31(2)135-57)65-89(130)101-63(90(131)132-4)43-23-39(106)24-51(140-93-79(124)74(119)70(115)54(26-104)141-93)58(43)42-17-36(10-15-45(42)107)60(85(126)103-65)98-87(128)62(38)99-86(127)61(37)100-88(64)129;1-5(2,3)4/h5-24,31-32,44,48,54-57,59-80,82-83,91-95,104-124H,25-29,96-97H2,1-4H3,(H,98,128)(H,99,127)(H,100,129)(H,101,130)(H,102,125)(H,103,126);(H2,1,2,3,4) |
InChI-Schlüssel |
HHRPQUHYQBGHHF-UHFFFAOYSA-N |
SMILES |
CC1C(C(CC(O1)OC2C3C(=O)NC(C4=C(C(=CC(=C4)O)OC5C(C(C(C(O5)CO)O)O)O)C6=C(C=CC(=C6)C(C(=O)N3)NC(=O)C7C8=CC(=C(C(=C8)OC9=CC=C2C=C9)OC1C(C(C(C(O1)COC1C(C(C(C(O1)C)O)O)O)O)O)O)OC1=CC=C(C=C1)C(C1C(=O)NC(C2=CC(=C(C(=C2)OC2=C(C=CC(=C2)C(C(=O)N1)N)O)C)O)C(=O)N7)O)O)C(=O)OC)N)O.OS(=O)(=O)O |
Isomerische SMILES |
C[C@H]1[C@@H]([C@@H](C[C@@H](O1)O[C@H]2[C@H]3C(=O)N[C@@H](C4=C(C(=CC(=C4)O)O[C@H]5[C@H]([C@H]([C@@H]([C@H](O5)CO)O)O)O)C6=C(C=CC(=C6)[C@H](C(=O)N3)NC(=O)[C@H]7C8=CC(=C(C(=C8)OC9=CC=C2C=C9)O[C@H]1[C@@H]([C@H]([C@@H]([C@H](O1)CO[C@H]1[C@@H]([C@@H]([C@H]([C@@H](O1)C)O)O)O)O)O)O[C@@H]1[C@H]([C@H]([C@@H]([C@H](O1)CO)O)O)O[C@H]1[C@H]([C@@H]([C@@H](CO1)O)O)O)OC1=CC=C(C=C1)[C@H]([C@@H]1C(=O)N[C@@H](C2=CC(=C(C(=C2)OC2=C(C=CC(=C2)[C@H](C(=O)N1)[NH3+])O)C)O)C(=O)N7)O)O)C(=O)OC)[NH3+])O.[O-]S(=O)(=O)[O-] |
Kanonische SMILES |
CC1C(C(CC(O1)OC2C3C(=O)NC(C4=C(C(=CC(=C4)O)OC5C(C(C(C(O5)CO)O)O)O)C6=C(C=CC(=C6)C(C(=O)N3)NC(=O)C7C8=CC(=C(C(=C8)OC9=CC=C2C=C9)OC1C(C(C(C(O1)COC1C(C(C(C(O1)C)O)O)O)O)O)OC1C(C(C(C(O1)CO)O)O)OC1C(C(C(CO1)O)O)O)OC1=CC=C(C=C1)C(C1C(=O)NC(C2=CC(=C(C(=C2)OC2=C(C=CC(=C2)C(C(=O)N1)N)O)C)O)C(=O)N7)O)O)C(=O)OC)N)O.OS(=O)(=O)O |
Aussehen |
Light tan powder |
Synonyme |
Ristocetin Sulfate; Ristomycin Sulfate |
Herkunft des Produkts |
United States |
Haftungsausschluss und Informationen zu In-Vitro-Forschungsprodukten
Bitte beachten Sie, dass alle Artikel und Produktinformationen, die auf BenchChem präsentiert werden, ausschließlich zu Informationszwecken bestimmt sind. Die auf BenchChem zum Kauf angebotenen Produkte sind speziell für In-vitro-Studien konzipiert, die außerhalb lebender Organismen durchgeführt werden. In-vitro-Studien, abgeleitet von dem lateinischen Begriff "in Glas", beinhalten Experimente, die in kontrollierten Laborumgebungen unter Verwendung von Zellen oder Geweben durchgeführt werden. Es ist wichtig zu beachten, dass diese Produkte nicht als Arzneimittel oder Medikamente eingestuft sind und keine Zulassung der FDA für die Vorbeugung, Behandlung oder Heilung von medizinischen Zuständen, Beschwerden oder Krankheiten erhalten haben. Wir müssen betonen, dass jede Form der körperlichen Einführung dieser Produkte in Menschen oder Tiere gesetzlich strikt untersagt ist. Es ist unerlässlich, sich an diese Richtlinien zu halten, um die Einhaltung rechtlicher und ethischer Standards in Forschung und Experiment zu gewährleisten.