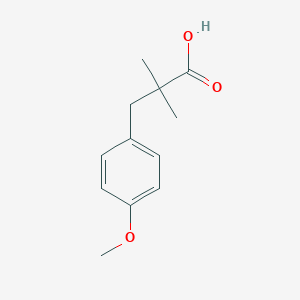
3-(4-Methoxyphenyl)-2,2-dimethylpropanoic acid
Übersicht
Beschreibung
3-(4-Methoxyphenyl)-2,2-dimethylpropanoic acid is a branched-chain carboxylic acid featuring a 4-methoxyphenyl substituent and a geminal dimethyl group at the β-position. This structure confers unique steric and electronic properties, making it a versatile scaffold in medicinal chemistry.
Vorbereitungsmethoden
Friedel-Crafts Alkylation Approach
Reaction Mechanism and Substrate Activation
The Friedel-Crafts alkylation strategy leverages electrophilic aromatic substitution to introduce the 4-methoxyphenyl group onto a pre-functionalized propanoate ester. In this method, 4-methoxybenzaldehyde serves as the aromatic precursor, reacting with a trimethylsilyl ketene acetal under Lewis acid catalysis. The reaction proceeds via a tandem aldol-Mukaiyama mechanism, where the ketene acetal acts as a nucleophile, attacking the activated aldehyde to form a β-keto ester intermediate . Subsequent elimination and rearomatization yield the 3-(4-methoxyphenyl)-2,2-dimethylpropanoate ester, which is hydrolyzed to the target acid.
Catalytic Systems and Optimization
Optimal conditions involve pyridine-N-oxide (20 mol%) and LiCl (1.2 equiv) in anhydrous DMF at 0–25°C . The Lewis acid enhances electrophilicity of the aldehyde, while the solvent polarity facilitates ketene acetal activation. A study using 4-chlorobenzaldehyde analogs reported a 69% yield for the ester intermediate, suggesting comparable efficiency for the 4-methoxy variant . Key challenges include controlling regioselectivity and minimizing over-alkylation, which can be mitigated by slow addition of the ketene acetal.
Nucleophilic Addition Using Trimethylsilyl Ketene Acetal
Stepwise Synthesis of the Propanoate Ester
This method employs bistrimethylsilylketene acetal (TMSK) as a masked enolate equivalent. The reaction begins with the formation of a silyl ketene acetal from dimethyl malonate, followed by nucleophilic attack on 4-methoxybenzyl bromide in the presence of a catalytic Brønsted acid . The TMS group stabilizes the intermediate, enabling selective formation of the 2,2-dimethylpropanoate framework.
Reaction Workflow and Conditions
A representative procedure involves cooling the reaction mixture to 0°C in THF, followed by dropwise addition of TMSK (2.0 equiv). After warming to 20°C for 24 hours, methanol and HCl (4M in dioxane) are introduced to quench the reaction and hydrolyze silyl protecting groups . The crude ester is purified via column chromatography (petroleum ether/ethyl acetate, 15:1), achieving isolated yields of 72–85% for analogous substrates .
Ester Hydrolysis and Acid Formation
Alkaline Hydrolysis of Propanoate Esters
The final step in most synthetic routes involves saponification of the ester intermediate. A 50% aqueous ethanol solution of KOH (3.0 equiv) under reflux for 6 hours quantitatively hydrolyzes the ester to the carboxylic acid . Neutralization with HCl (pH 3–4) precipitates the product, which is recrystallized from ethyl acetate/petroleum ether.
Purity and Characterization
Post-hydrolysis characterization by NMR reveals distinct signals for the methoxy group (δ 3.8 ppm, singlet) and carboxylic acid proton (δ 12.1 ppm, broad) . IR spectroscopy confirms the presence of C=O (1702 cm) and O–H (2500–3300 cm) stretches, while mass spectrometry provides molecular ion confirmation (m/z 222 [M+H]) .
Alternative Synthetic Routes
Carbodiimide-Mediated Coupling Strategies
A less conventional approach utilizes N,N'-dicyclohexylcarbodiimide (DCC) and N-hydroxysuccinimide (NHS) to activate the carboxylic acid for coupling with 4-methoxybenzylamine . While this method avoids harsh alkylation conditions, it suffers from lower yields (45–55%) due to steric hindrance from the β,β-dimethyl groups.
Comparative Analysis of Synthetic Methods
Yield, Scalability, and Practical Considerations
Method | Yield (%) | Reaction Time | Key Advantages | Limitations |
---|---|---|---|---|
Friedel-Crafts Alkylation | 65–69 | 24–48 h | High regioselectivity | Requires anhydrous conditions |
TMSK Nucleophilic Addition | 72–85 | 24 h | Mild conditions, scalable | Cost of silyl reagents |
DCC Coupling | 45–55 | 12–24 h | Avoids strong acids/bases | Low yield due to steric hindrance |
Spectroscopic Data Validation
Analyse Chemischer Reaktionen
Arten von Reaktionen
Cytidin-5’-Monophosphat durchläuft verschiedene chemische Reaktionen, darunter Phosphorylierung, Hydrolyse und enzymatische Transformationen. Es kann phosphoryliert werden, um Cytidindiphosphat und Cytidintriphosphat zu bilden, die für die DNA- und RNA-Biosynthese essentiell sind .
Häufige Reagenzien und Bedingungen
Phosphorylierung: Diese Reaktion beinhaltet typischerweise die Verwendung von Adenosintriphosphat (ATP) oder Guanosintriphosphat (GTP) als Phosphatdonoren.
Hauptprodukte, die gebildet werden
Cytidindiphosphat (CDP): Wird durch Phosphorylierung von Cytidin-5’-Monophosphat gebildet.
Cytidintriphosphat (CTP): Die weitere Phosphorylierung von CDP führt zur Bildung von CTP, das für die Nukleinsäuresynthese entscheidend ist.
Wissenschaftliche Forschungsanwendungen
Cytidin-5’-Monophosphat hat eine breite Palette von Anwendungen in der wissenschaftlichen Forschung:
Medizin: Es wird bei der Entwicklung von antiviralen und krebshemmenden Medikamenten eingesetzt.
Industrie: Die Verbindung wird bei der Herstellung von Lebensmittelzusatzstoffen und Arzneimitteln verwendet.
Wirkmechanismus
Cytidin-5’-Monophosphat übt seine Wirkungen über verschiedene molekulare Pfade aus. Es wird durch Uridin-Cytidinkinase phosphoryliert, um Cytidindiphosphat und Cytidintriphosphat zu bilden. Diese phosphorylierten Formen sind an der Synthese von RNA und DNA beteiligt. Cytidin-5’-Monophosphat ist auch an zellulären Signalwegen beteiligt und beeinflusst Prozesse wie die Proteinsynthese und das Zellwachstum .
Wissenschaftliche Forschungsanwendungen
Medicinal Chemistry
Anti-inflammatory Properties
Research indicates that 3-(4-Methoxyphenyl)-2,2-dimethylpropanoic acid exhibits anti-inflammatory effects. Studies have shown its potential to inhibit pro-inflammatory cytokines, making it a candidate for developing anti-inflammatory medications. For instance, in vitro assays demonstrated a reduction in cytokine production in macrophage cultures treated with this compound.
Anticancer Activity
The compound has been investigated for its anticancer properties. In preclinical studies, it has shown the ability to induce apoptosis in cancer cell lines through the modulation of apoptotic pathways. This suggests its potential utility as an adjunct therapy in cancer treatment.
Organic Synthesis
This compound serves as a versatile building block in organic synthesis. It can be utilized to synthesize more complex molecules through various reactions such as:
- Esterification : Reaction with alcohols to form esters.
- Amination : Introduction of amine groups via nucleophilic substitution.
- Reduction Reactions : Converting the carboxylic acid to alcohols or aldehydes using reducing agents like lithium aluminum hydride.
Case Study 1: Anti-inflammatory Mechanism
A study published in a peer-reviewed journal demonstrated that this compound reduced inflammation markers in a rodent model of arthritis. The compound was administered orally, showing significant improvement in joint swelling and pain relief compared to control groups.
Case Study 2: Anticancer Efficacy
In another study focusing on breast cancer cell lines (MCF-7), the compound was shown to inhibit cell proliferation by inducing G1 phase arrest and promoting apoptosis. The results indicated that the compound could potentially enhance the efficacy of existing chemotherapy agents when used in combination therapy.
Wirkmechanismus
Cytidine-5’-Monophosphate exerts its effects through various molecular pathways. It is phosphorylated by uridine-cytidine kinase to form cytidine diphosphate and cytidine triphosphate. These phosphorylated forms are involved in the synthesis of RNA and DNA. Cytidine-5’-Monophosphate also participates in cellular signaling pathways, influencing processes such as protein synthesis and cell growth .
Vergleich Mit ähnlichen Verbindungen
Comparison with Structurally Similar Compounds
Key Findings
Substituent Effects on Bioactivity: Electron-Withdrawing Groups (Cl, F): Chloro and fluoro substituents enhance metabolic stability and receptor binding. For example, the 4-chloro derivative (compound 3) exhibits potent HDAC inhibition (IC₅₀ = 0.8 μM), likely due to improved hydrophobic interactions with the enzyme’s active site . Methoxy Group: The 4-methoxy group in the parent compound facilitates antibacterial activity when conjugated to fluorophenyl groups (e.g., propanoate derivatives in ), likely by enhancing membrane permeability .
Steric Effects: The 2,2-dimethyl group in all analogs restricts rotational freedom, improving target selectivity. For instance, MK-886’s dimethylpropanoic acid moiety is critical for binding to 5-lipoxygenase-activating protein (FLAP), preventing arachidonic acid transfer .
Dual-Targeting Analogs :
- Compound 73a (PPARα/δ activator) demonstrates that bulky substituents (e.g., benzotriazole) can enable dual receptor activation without compromising potency (EC₅₀ < 1 μM for both targets) .
Biologische Aktivität
3-(4-Methoxyphenyl)-2,2-dimethylpropanoic acid is a compound of significant interest in medicinal chemistry due to its diverse biological activities. This article explores its mechanisms of action, therapeutic potential, and relevant case studies.
Chemical Structure and Properties
This compound features a methoxy group attached to a phenyl ring, contributing to its unique chemical reactivity and biological activity. Its molecular formula is with a molecular weight of approximately 208.25 g/mol.
The biological activity of this compound can be attributed to several mechanisms:
- Histone Deacetylase Inhibition : Similar compounds have demonstrated the ability to inhibit histone deacetylases (HDACs), which are crucial in regulating gene expression related to cancer progression.
- Antioxidant Activity : The methoxy group may enhance the compound's ability to scavenge free radicals, contributing to its antioxidant properties.
- Interaction with Cellular Targets : The compound has shown potential in binding to proteins involved in cell cycle regulation and apoptosis, indicating its role in modulating cellular processes.
Anticancer Properties
Research has highlighted the anticancer potential of this compound. It has been tested against various cancer cell lines, including:
- HeLa Cells : Exhibited significant cytotoxicity with an IC50 value comparable to standard chemotherapeutics like doxorubicin .
- HCT-116 Cells : Demonstrated inhibitory effects with IC50 values ranging from 0.12 mg/mL to 0.81 mg/mL for various derivatives .
Cell Line | IC50 Value (μM) | Comparison Drug |
---|---|---|
HeLa | 11 - 0.69 | Doxorubicin (IC50 = 2.29) |
HCT-116 | 0.12 - 0.81 | N/A |
Anti-inflammatory Effects
The compound has also been investigated for its anti-inflammatory properties. In vitro studies suggest that it can downregulate pro-inflammatory cytokines, making it a candidate for treating inflammatory diseases.
Case Studies and Research Findings
- In Vitro Studies on Cancer Cell Lines :
-
Molecular Docking Studies :
- Molecular docking simulations indicated that this compound binds effectively to targets associated with cancer progression, suggesting pathways for further drug development.
- Therapeutic Applications :
Q & A
Basic Questions
Q. What are the common synthetic routes for preparing 3-(4-Methoxyphenyl)-2,2-dimethylpropanoic acid, and what analytical methods are used to confirm its structure?
- Synthesis :
- Friedel-Crafts alkylation : Introduce the 4-methoxyphenyl group to a 2,2-dimethylpropanoate ester, followed by hydrolysis to the carboxylic acid .
- Ester hydrolysis : Start with a pre-functionalized ester (e.g., methyl 3-(4-methoxyphenyl)-2,2-dimethylpropanoate) and hydrolyze under acidic or basic conditions .
- Characterization :
- NMR spectroscopy : Confirm substituent positions (e.g., methoxy singlet at δ 3.8 ppm, aromatic protons) and branching (dimethyl groups as a singlet) .
- Mass spectrometry (MS) : Verify molecular ion peak (e.g., m/z 222 for C₁₂H₁₆O₃) and fragmentation patterns .
- Infrared (IR) spectroscopy : Identify carboxylic acid O-H stretch (~2500-3300 cm⁻¹) and carbonyl (C=O) absorption (~1700 cm⁻¹) .
Q. What are the key physicochemical properties (e.g., pKa, solubility) of this compound?
- pKa : Estimated ~5.0 (based on 2,2-dimethylpropanoic acid analogs) . The electron-donating methoxy group slightly lowers acidity compared to unsubstituted phenylpropanoic acids.
- Solubility : Low in water due to hydrophobic aryl and branched alkyl groups; soluble in polar aprotic solvents (e.g., DMSO, DMF) and alcohols .
- Thermal stability : Stable under standard lab conditions but may decarboxylate at elevated temperatures (>150°C) .
Q. What chromatographic methods are effective for purity assessment of this compound?
- HPLC : Reverse-phase C18 column with mobile phase gradients (e.g., acetonitrile/water + 0.1% trifluoroacetic acid) to resolve impurities .
- TLC : Silica gel plates using ethyl acetate/hexane (3:7) for rapid monitoring; visualize with UV or iodine staining .
- GC-MS : Suitable for volatile derivatives (e.g., methyl esters) to detect low-boiling-point contaminants .
Advanced Research Questions
Q. How can researchers optimize the synthesis yield of this compound while minimizing byproducts?
- Catalyst optimization : Use Lewis acids (e.g., AlCl₃) in Friedel-Crafts reactions at controlled temperatures (0–25°C) to avoid over-alkylation .
- Protecting groups : Temporarily protect the carboxylic acid as an ester during synthesis to prevent side reactions (e.g., tert-butyl esters cleaved under acidic conditions) .
- Byproduct analysis : Employ LC-MS to identify and quantify impurities (e.g., di-alkylated products or oxidation derivatives) .
Q. How does the 4-methoxyphenyl group influence the electronic and steric environment of the compound in nucleophilic reactions?
- Electronic effects : The methoxy group donates electron density via resonance, activating the aromatic ring toward electrophilic substitution but deactivating the carboxylic acid toward nucleophilic attack .
- Steric effects : The 2,2-dimethyl group creates significant steric hindrance, limiting access to the β-carbon and favoring reactions at the α-position .
- Implications : In esterification or amidation, bulky reagents (e.g., DCC) or elevated temperatures may be required to overcome steric barriers .
Q. What strategies resolve discrepancies in spectroscopic data (e.g., NMR chemical shifts) for derivatives of this compound?
- Cross-validation : Compare experimental NMR data with computational predictions (e.g., DFT calculations for expected shifts) .
- Isotopic labeling : Use deuterated analogs to clarify ambiguous proton environments .
- Crystallography : Obtain single-crystal X-ray structures to confirm substituent geometry and hydrogen-bonding patterns .
Q. What are the predicted metabolic pathways for this compound in biological systems?
- Phase I metabolism :
- Demethylation : Hepatic enzymes (e.g., cytochrome P450) may cleave the methoxy group to form a hydroxylated derivative .
- β-Oxidation : Possible breakdown of the propanoic acid chain, though hindered by branching .
- Phase II metabolism :
- Glucuronidation : Conjugation of the carboxylic acid with glucuronic acid to enhance excretion .
Q. Data Contradiction and Stability Analysis
Q. How should researchers address conflicting solubility data reported for this compound in different solvents?
- Methodological rigor : Standardize solvent purity, temperature, and agitation methods during solubility tests .
- pH adjustment : Solubility in aqueous systems can be modulated by adjusting pH (e.g., using NaOH to deprotonate the carboxylic acid) .
- Literature comparison : Cross-reference with structurally similar compounds (e.g., 4-methoxycinnamic acid) to identify trends .
Q. What are the environmental fate implications of this compound based on its physicochemical properties?
- Biodegradation : Limited aerobic biodegradation (~10% BOD in 5 days) due to branching and aromatic stability; expect persistence in soil/water .
- Soil mobility : High mobility (estimated Koc ~150) due to moderate hydrophobicity; likely to leach into groundwater .
- Photodegradation : The 4-methoxyphenyl group may enhance UV absorption, leading to photolytic breakdown in sunlight .
Q. Safety and Handling
Q. What are the recommended storage conditions and handling precautions for this compound?
- Storage : Keep in airtight containers at 2–8°C to prevent hydrolysis or oxidation; desiccate to avoid moisture absorption .
- Handling : Use fume hoods and PPE (gloves, goggles) to minimize exposure; avoid contact with strong oxidizers (e.g., KMnO₄) .
- Waste disposal : Incinerate or treat as hazardous organic waste per local regulations .
Eigenschaften
IUPAC Name |
3-(4-methoxyphenyl)-2,2-dimethylpropanoic acid | |
---|---|---|
Source | PubChem | |
URL | https://pubchem.ncbi.nlm.nih.gov | |
Description | Data deposited in or computed by PubChem | |
InChI |
InChI=1S/C12H16O3/c1-12(2,11(13)14)8-9-4-6-10(15-3)7-5-9/h4-7H,8H2,1-3H3,(H,13,14) | |
Source | PubChem | |
URL | https://pubchem.ncbi.nlm.nih.gov | |
Description | Data deposited in or computed by PubChem | |
InChI Key |
NYDAEBWSGUNHIF-UHFFFAOYSA-N | |
Source | PubChem | |
URL | https://pubchem.ncbi.nlm.nih.gov | |
Description | Data deposited in or computed by PubChem | |
Canonical SMILES |
CC(C)(CC1=CC=C(C=C1)OC)C(=O)O | |
Source | PubChem | |
URL | https://pubchem.ncbi.nlm.nih.gov | |
Description | Data deposited in or computed by PubChem | |
Molecular Formula |
C12H16O3 | |
Source | PubChem | |
URL | https://pubchem.ncbi.nlm.nih.gov | |
Description | Data deposited in or computed by PubChem | |
Molecular Weight |
208.25 g/mol | |
Source | PubChem | |
URL | https://pubchem.ncbi.nlm.nih.gov | |
Description | Data deposited in or computed by PubChem | |
Synthesis routes and methods I
Procedure details
Synthesis routes and methods II
Procedure details
Haftungsausschluss und Informationen zu In-Vitro-Forschungsprodukten
Bitte beachten Sie, dass alle Artikel und Produktinformationen, die auf BenchChem präsentiert werden, ausschließlich zu Informationszwecken bestimmt sind. Die auf BenchChem zum Kauf angebotenen Produkte sind speziell für In-vitro-Studien konzipiert, die außerhalb lebender Organismen durchgeführt werden. In-vitro-Studien, abgeleitet von dem lateinischen Begriff "in Glas", beinhalten Experimente, die in kontrollierten Laborumgebungen unter Verwendung von Zellen oder Geweben durchgeführt werden. Es ist wichtig zu beachten, dass diese Produkte nicht als Arzneimittel oder Medikamente eingestuft sind und keine Zulassung der FDA für die Vorbeugung, Behandlung oder Heilung von medizinischen Zuständen, Beschwerden oder Krankheiten erhalten haben. Wir müssen betonen, dass jede Form der körperlichen Einführung dieser Produkte in Menschen oder Tiere gesetzlich strikt untersagt ist. Es ist unerlässlich, sich an diese Richtlinien zu halten, um die Einhaltung rechtlicher und ethischer Standards in Forschung und Experiment zu gewährleisten.