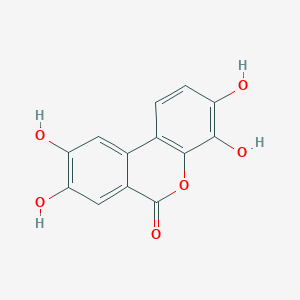
Urolithin D
Übersicht
Beschreibung
Urolithin D is a secondary metabolite derived from ellagic acid and ellagitannins, which are found in various fruits and nuts such as pomegranates, berries, and walnuts . These compounds are transformed into urolithins by the gut microbiota. This compound, along with other urolithins, has garnered attention for its potential health benefits, particularly in the fields of cancer prevention and anti-aging .
Wissenschaftliche Forschungsanwendungen
Urolithin D hat eine breite Palette von Anwendungen in der wissenschaftlichen Forschung:
Chemie: Wird als Modellverbindung verwendet, um den Metabolismus von Ellagitanninen und Ellagisäure zu untersuchen.
Biologie: Untersucht auf seine Rolle bei der Modulation der Darmflora und seine Auswirkungen auf die Gesundheit.
5. Wirkmechanismus
This compound übt seine Wirkungen über verschiedene molekulare Ziele und Pfade aus:
Antikrebs-Mechanismus: Es induziert Apoptose in Krebszellen, indem es Caspasen aktiviert und die Zellproliferation durch Herunterregulierung von Onkogenen hemmt.
Entzündungshemmender Mechanismus: Es moduliert Entzündungspfade, indem es die Produktion von proinflammatorischen Zytokinen hemmt und die Aktivierung von entzündungshemmenden Zytokinen aktiviert.
Antioxidativer Mechanismus: this compound fängt freie Radikale ab und reduziert oxidativen Stress, indem es die Aktivität von antioxidativen Enzymen verstärkt.
Ähnliche Verbindungen:
- Urolithin A
- Urolithin B
- Urolithin C
Vergleich:
- Urolithin A: Bekannt für seine starken entzündungshemmenden und antioxidativen Eigenschaften. Es ist das am besten untersuchte Urolithin für seine Rolle bei der Förderung der mitochondrialen Gesundheit .
- Urolithin B: Zeigt signifikante entzündungshemmende und Antikrebs-Aktivitäten. Es ist das letzte Produkt, das im katabolen Weg von Ellagitanninen gebildet wird .
- Urolithin C: Weniger untersucht als Urolithin A und B, aber es zeigt ebenfalls potenzielle Antikrebs- und antioxidative Eigenschaften .
Einzigartigkeit von this compound: this compound ist einzigartig aufgrund seiner spezifischen molekularen Struktur, die es ihm ermöglicht, mit verschiedenen molekularen Zielstrukturen zu interagieren, verglichen mit anderen Urolithin. Seine besonderen chemischen Eigenschaften machen es zu einer wertvollen Verbindung für die Untersuchung des Metabolismus von Ellagitanninen und die Erforschung neuer therapeutischer Anwendungen .
Wirkmechanismus
Target of Action
Urolithin D is known to interact with several targets in the body. It is a competitive and reversible antagonist of EphA receptors . It also interacts with multiple receptors such as the aryl hydrocarbon receptor, estrogen and androgen receptors . These receptors play a crucial role in various cellular processes, including cell proliferation, differentiation, and apoptosis.
Mode of Action
This compound interacts with its targets and induces several changes in cellular processes. It mediates its cancer-preventive activities through cell cycle arrest, aromatase inhibition, induction of apoptosis, tumor suppression, promotion of autophagy, and senescence, transcriptional regulation of oncogenes, and growth factor receptors . It also inhibits 17β-hydroxysteroid dehydrogenases (17β-HSD1), an enzyme involved in dihydrotestosterone (DHT) inactivation and in the conversion of inactive estrone (E1) to estradiol (E2), which is critical for estradiol synthesis .
Biochemical Pathways
This compound affects several biochemical pathways. It is involved in the PI3K-Akt and Wnt/β-catenin pathways . These pathways play a crucial role in cell survival, growth, and proliferation. This compound’s interaction with these pathways leads to the inhibition of cell proliferation and induction of apoptosis, contributing to its anticancer effects .
Pharmacokinetics
The pharmacokinetics of this compound is yet to be fully understood. Studies on urolithin a, a closely related compound, suggest that these compounds have low aqueous solubility and a short elimination half-life . This could potentially impact the bioavailability of this compound. Efforts are being made to improve the bioavailability of these compounds, such as the development of PEGylated liposomes .
Result of Action
The action of this compound at the molecular and cellular level results in several effects. It has been found to inhibit cell proliferation and induce apoptosis in cancer cells . It also modulates the expression of various oncogenes and growth factor receptors, leading to tumor suppression .
Action Environment
The action of this compound can be influenced by various environmental factors. For instance, the composition and activity of an individual’s intestinal microbiota play a pivotal role in the metabolism and absorption of this compound . This suggests that the gut microbiome could potentially influence the efficacy and stability of this compound.
Biochemische Analyse
Biochemical Properties
Urolithin D interacts with various enzymes, proteins, and other biomolecules in the body. It is known to mediate its cancer-preventive activities through cell cycle arrest, aromatase inhibition, induction of apoptosis, tumor suppression, promotion of autophagy, and senescence, transcriptional regulation of oncogenes, and growth factor receptors .
Cellular Effects
This compound has been shown to have significant effects on various types of cells and cellular processes. For instance, it has been found to inhibit cell proliferation and induce apoptosis in prostate cancer cell lines . It also influences cell function by impacting cell signaling pathways, gene expression, and cellular metabolism .
Molecular Mechanism
At the molecular level, this compound exerts its effects through binding interactions with biomolecules, enzyme inhibition or activation, and changes in gene expression . Its anticancer properties are mediated through cell cycle arrest, aromatase inhibition, induction of apoptosis, tumor suppression, promotion of autophagy, and senescence, transcriptional regulation of oncogenes, and growth factor receptors .
Temporal Effects in Laboratory Settings
In laboratory settings, the effects of this compound change over time. It has been observed that Urolithin B, a closely related compound, is more stable under conditions of the gastrointestinal tract compared to Urolithin A and ellagic acid
Dosage Effects in Animal Models
The effects of this compound vary with different dosages in animal models. High concentrations of Urolithins have shown cytotoxic activity .
Metabolic Pathways
This compound is involved in various metabolic pathways. It is produced in the gut through the microbial action on ellagitannins and ellagic acid-rich foods
Transport and Distribution
This compound is transported and distributed within cells and tissues. Studies have shown that Urolithin A, a related compound, can be metabolized to form glucuronides in a time-dependent manner
Vorbereitungsmethoden
Synthetische Wege und Reaktionsbedingungen: Die Synthese von Urolithin D beinhaltet typischerweise die Reaktion von 2-Brom-4,5-Dimethoxybenzoesäure mit Pyrogallol in Gegenwart von Kaliumhydroxid . Die Mischung wird erhitzt, gefolgt von der Zugabe einer Kupfersulfatlösung. Der resultierende Niederschlag wird filtriert, gewaschen und getrocknet. Weitere Schritte umfassen die Verwendung von Eisessig und Bromwasserstoffsäure, gefolgt von Säulenchromatographie unter Verwendung von Kieselgel .
Industrielle Produktionsverfahren: Die industrielle Produktion von this compound kann unter Verwendung ähnlicher synthetischer Wege mit Modifikationen zur Optimierung von Ausbeute und Reinheit im größeren Maßstab durchgeführt werden. Die Verwendung von Säulenchromatographie auf Kieselgel ist eine übliche Methode zur Reinigung der Verbindung in größerem Maßstab .
Analyse Chemischer Reaktionen
Arten von Reaktionen: Urolithin D unterliegt verschiedenen chemischen Reaktionen, darunter:
Oxidation: this compound kann oxidiert werden, um Chinone und andere oxidierte Derivate zu bilden.
Reduktion: Es kann reduziert werden, um Dihydroderivate zu bilden.
Substitution: this compound kann Substitutionsreaktionen eingehen, insbesondere an den Hydroxylgruppen.
Häufige Reagenzien und Bedingungen:
Oxidation: Häufige Oxidationsmittel umfassen Kaliumpermanganat und Wasserstoffperoxid.
Reduktion: Reduktionsmittel wie Natriumborhydrid werden verwendet.
Substitution: Reagenzien wie Essigsäureanhydrid und Schwefelsäure werden für Acetylierungsreaktionen verwendet.
Wichtigste gebildete Produkte:
Oxidation: Chinone und andere oxidierte Derivate.
Reduktion: Dihydroderivate.
Substitution: Acetylierte Derivate.
Vergleich Mit ähnlichen Verbindungen
- Urolithin A
- Urolithin B
- Urolithin C
Comparison:
- Urolithin A: Known for its strong anti-inflammatory and antioxidant properties. It is the most studied urolithin for its role in promoting mitochondrial health .
- Urolithin B: Exhibits significant anti-inflammatory and anticancer activities. It is the last product formed in the catabolic pathway of ellagitannins .
- Urolithin C: Less studied compared to Urolithin A and B, but it also shows potential anticancer and antioxidant properties .
Uniqueness of Urolithin D: this compound is unique due to its specific molecular structure, which allows it to interact with different molecular targets compared to other urolithins. Its distinct chemical properties make it a valuable compound for studying the metabolism of ellagitannins and exploring new therapeutic applications .
Eigenschaften
IUPAC Name |
3,4,8,9-tetrahydroxybenzo[c]chromen-6-one | |
---|---|---|
Source | PubChem | |
URL | https://pubchem.ncbi.nlm.nih.gov | |
Description | Data deposited in or computed by PubChem | |
InChI |
InChI=1S/C13H8O6/c14-8-2-1-5-6-3-9(15)10(16)4-7(6)13(18)19-12(5)11(8)17/h1-4,14-17H | |
Source | PubChem | |
URL | https://pubchem.ncbi.nlm.nih.gov | |
Description | Data deposited in or computed by PubChem | |
InChI Key |
NEZDQSKPNPRYAW-UHFFFAOYSA-N | |
Source | PubChem | |
URL | https://pubchem.ncbi.nlm.nih.gov | |
Description | Data deposited in or computed by PubChem | |
Canonical SMILES |
C1=CC(=C(C2=C1C3=CC(=C(C=C3C(=O)O2)O)O)O)O | |
Source | PubChem | |
URL | https://pubchem.ncbi.nlm.nih.gov | |
Description | Data deposited in or computed by PubChem | |
Molecular Formula |
C13H8O6 | |
Source | PubChem | |
URL | https://pubchem.ncbi.nlm.nih.gov | |
Description | Data deposited in or computed by PubChem | |
DSSTOX Substance ID |
DTXSID70156886 | |
Record name | 6H-Dibenzo(b,d)pyran-6-one, 3,4,8,9-tetrahydroxy- | |
Source | EPA DSSTox | |
URL | https://comptox.epa.gov/dashboard/DTXSID70156886 | |
Description | DSSTox provides a high quality public chemistry resource for supporting improved predictive toxicology. | |
Molecular Weight |
260.20 g/mol | |
Source | PubChem | |
URL | https://pubchem.ncbi.nlm.nih.gov | |
Description | Data deposited in or computed by PubChem | |
Physical Description |
Solid | |
Record name | Urolithin D | |
Source | Human Metabolome Database (HMDB) | |
URL | http://www.hmdb.ca/metabolites/HMDB0029219 | |
Description | The Human Metabolome Database (HMDB) is a freely available electronic database containing detailed information about small molecule metabolites found in the human body. | |
Explanation | HMDB is offered to the public as a freely available resource. Use and re-distribution of the data, in whole or in part, for commercial purposes requires explicit permission of the authors and explicit acknowledgment of the source material (HMDB) and the original publication (see the HMDB citing page). We ask that users who download significant portions of the database cite the HMDB paper in any resulting publications. | |
CAS No. |
131086-98-1 | |
Record name | 6H-Dibenzo(b,d)pyran-6-one, 3,4,8,9-tetrahydroxy- | |
Source | ChemIDplus | |
URL | https://pubchem.ncbi.nlm.nih.gov/substance/?source=chemidplus&sourceid=0131086981 | |
Description | ChemIDplus is a free, web search system that provides access to the structure and nomenclature authority files used for the identification of chemical substances cited in National Library of Medicine (NLM) databases, including the TOXNET system. | |
Record name | 6H-Dibenzo(b,d)pyran-6-one, 3,4,8,9-tetrahydroxy- | |
Source | EPA DSSTox | |
URL | https://comptox.epa.gov/dashboard/DTXSID70156886 | |
Description | DSSTox provides a high quality public chemistry resource for supporting improved predictive toxicology. | |
Record name | Urolithin D | |
Source | FDA Global Substance Registration System (GSRS) | |
URL | https://gsrs.ncats.nih.gov/ginas/app/beta/substances/JVT9VXW2DJ | |
Description | The FDA Global Substance Registration System (GSRS) enables the efficient and accurate exchange of information on what substances are in regulated products. Instead of relying on names, which vary across regulatory domains, countries, and regions, the GSRS knowledge base makes it possible for substances to be defined by standardized, scientific descriptions. | |
Explanation | Unless otherwise noted, the contents of the FDA website (www.fda.gov), both text and graphics, are not copyrighted. They are in the public domain and may be republished, reprinted and otherwise used freely by anyone without the need to obtain permission from FDA. Credit to the U.S. Food and Drug Administration as the source is appreciated but not required. | |
Retrosynthesis Analysis
AI-Powered Synthesis Planning: Our tool employs the Template_relevance Pistachio, Template_relevance Bkms_metabolic, Template_relevance Pistachio_ringbreaker, Template_relevance Reaxys, Template_relevance Reaxys_biocatalysis model, leveraging a vast database of chemical reactions to predict feasible synthetic routes.
One-Step Synthesis Focus: Specifically designed for one-step synthesis, it provides concise and direct routes for your target compounds, streamlining the synthesis process.
Accurate Predictions: Utilizing the extensive PISTACHIO, BKMS_METABOLIC, PISTACHIO_RINGBREAKER, REAXYS, REAXYS_BIOCATALYSIS database, our tool offers high-accuracy predictions, reflecting the latest in chemical research and data.
Strategy Settings
Precursor scoring | Relevance Heuristic |
---|---|
Min. plausibility | 0.01 |
Model | Template_relevance |
Template Set | Pistachio/Bkms_metabolic/Pistachio_ringbreaker/Reaxys/Reaxys_biocatalysis |
Top-N result to add to graph | 6 |
Feasible Synthetic Routes
Haftungsausschluss und Informationen zu In-Vitro-Forschungsprodukten
Bitte beachten Sie, dass alle Artikel und Produktinformationen, die auf BenchChem präsentiert werden, ausschließlich zu Informationszwecken bestimmt sind. Die auf BenchChem zum Kauf angebotenen Produkte sind speziell für In-vitro-Studien konzipiert, die außerhalb lebender Organismen durchgeführt werden. In-vitro-Studien, abgeleitet von dem lateinischen Begriff "in Glas", beinhalten Experimente, die in kontrollierten Laborumgebungen unter Verwendung von Zellen oder Geweben durchgeführt werden. Es ist wichtig zu beachten, dass diese Produkte nicht als Arzneimittel oder Medikamente eingestuft sind und keine Zulassung der FDA für die Vorbeugung, Behandlung oder Heilung von medizinischen Zuständen, Beschwerden oder Krankheiten erhalten haben. Wir müssen betonen, dass jede Form der körperlichen Einführung dieser Produkte in Menschen oder Tiere gesetzlich strikt untersagt ist. Es ist unerlässlich, sich an diese Richtlinien zu halten, um die Einhaltung rechtlicher und ethischer Standards in Forschung und Experiment zu gewährleisten.