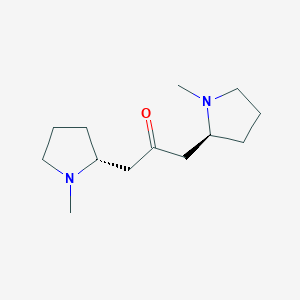
Cuscohygrin
Übersicht
Beschreibung
Cuscohygrine is a bis N-methyl pyrrolidine alkaloid found in coca plants. It can also be extracted from plants of the family Solanaceae, including Atropa belladonna (deadly nightshade) and various Datura species . Cuscohygrine usually occurs along with other, more potent alkaloids such as atropine or cocaine . It was first isolated by Carl Liebermann in 1889 as an alkaloid accompanying cocaine in coca leaves .
Wissenschaftliche Forschungsanwendungen
Cuscohygrine has several scientific research applications. In chemistry, it is used to study the biosynthesis of alkaloids and their chemical properties . In biology, cuscohygrine is used to investigate the metabolic pathways of alkaloids in plants . In medicine, it is studied for its potential pharmacological effects, although it is less potent than other alkaloids like atropine or cocaine . In industry, cuscohygrine is used as a precursor for the synthesis of other chemical compounds .
Wirkmechanismus
Target of Action
Cuscohygrine is a bis N-methyl pyrrolidine alkaloid found in coca plants . It can also be extracted from plants of the family Solanaceae, including Atropa belladonna (deadly nightshade) and various Datura species . Cuscohygrine usually occurs along with other, more potent alkaloids such as atropine or cocaine
Mode of Action
Cuscohygrine has been docked with Acetylcholinesterase (AChE) through hydrogen bonds with Peripheral Anionic Site (PAS) and through hydrophobic interactions with the catalytic triad . This suggests that Cuscohygrine could potentially act as a dual inhibitor of AChE .
Biochemical Pathways
The biosynthesis of Cuscohygrine involves several steps . Ornithine is methylated to N-methylornithine and when decarboxylated, becomes N-methylputrescine . 4-methylaminobutanal is yielded from the oxidation of the primary amino-group . 4-methylaminobutanal then cyclizes to an N-methyl-l-pyrrolinium salt . The condensation of the pyrrolinium salt with acetoacetyl coenzyme A yields hygrine . Finally, the condensation of the hygrine molecule with another molecule of pyrrolidinium salt yields cuscohygrine .
Pharmacokinetics
Computational tools like admetsar30 can be used to predict these properties .
Result of Action
Its potential interaction with ache suggests it could influence neurotransmission .
Biochemische Analyse
Biochemical Properties
Cuscohygrine is involved in various biochemical reactions. It is a simple molecule and its structure has been decided by its degradations and reactions . It is an oil that can be distilled without decomposition only in vacuum . It is soluble in water . It also forms a crystalline trihydrate which melts at 40–41 °C .
Cellular Effects
It is known that Cuscohygrine usually occurs along with other, more potent alkaloids such as atropine or cocaine , which can have significant effects on various types of cells and cellular processes.
Molecular Mechanism
It is known that it is a bis N-methyl pyrrolidine alkaloid , suggesting that it may interact with biomolecules in a similar manner to other pyrrolidine alkaloids.
Temporal Effects in Laboratory Settings
It is known that Cuscohygrine is an oil that can be distilled without decomposition only in vacuum , suggesting that it may be stable over time under certain conditions.
Dosage Effects in Animal Models
It is known that Cuscohygrine usually occurs along with other, more potent alkaloids such as atropine or cocaine , which can have significant effects at different dosages.
Metabolic Pathways
Cuscohygrine is involved in the metabolic pathway of ornithine . Ornithine is methylated to N-methylornithine and when decarboxylated, becomes N-methylputrescine . 4-methylaminobutanal is yielded from the oxidation of the primary amino-group . 4-methylaminobutanal then cyclizes to an N-methyl-l-pyrrolinium salt . The condensation of the pyrrolinium salt with acetoacetyl coenzyme A yields hygrine . Finally, the condensation of the hygrine molecule with another molecule of pyrrolidinium salt yields cuscohygrine .
Transport and Distribution
It is known that Cuscohygrine is soluble in water , suggesting that it may be able to move freely within the aqueous environment of the cell.
Subcellular Localization
Given its solubility in water , it is likely that it can be found throughout the cell.
Vorbereitungsmethoden
Cuscohygrine is biogenetically formed by the addition of the anion of N-methyl-Δ′-pyrrolinium cation to another molecule of N-methyl-Δ′-pyrrolinium cation . The synthetic route involves the condensation of the pyrrolinium salt with acetoacetyl coenzyme A to yield hygrine, which then condenses with another molecule of pyrrolidinium salt to yield cuscohygrine .
Analyse Chemischer Reaktionen
Cuscohygrine undergoes various chemical reactions, including reduction and oxidation. Reduction of cuscohygrine with sodium and ethanol produces a mixture of two epimeric meso alcohols, α- and β-dihydrocuscohygrine . Common reagents used in these reactions include sodium, ethanol, and acetoacetyl coenzyme A . The major products formed from these reactions are dihydrocuscohygrine and hygrine .
Vergleich Mit ähnlichen Verbindungen
Cuscohygrine is similar to other alkaloids such as hygrine and tropine . it is unique in its bis N-methyl pyrrolidine structure, which distinguishes it from other alkaloids . Hygrine, for example, is a simple molecule with a single N-methyl pyrrolidine ring, while cuscohygrine has two such rings . Tropine, on the other hand, has a different structure and is more potent than cuscohygrine .
Biologische Aktivität
Cuscohygrine is a natural alkaloid predominantly found in the leaves of the coca plant (Erythroxylum coca). It is structurally related to other alkaloids such as hygrine and cocaine. While cuscohygrine has been primarily recognized for its role in forensic toxicology as a biomarker for distinguishing between coca leaf consumption and cocaine abuse, emerging research suggests potential biological activities that warrant further exploration.
Antifungal and Antimicrobial Properties
Research indicates that alkaloids, including cuscohygrine, exhibit significant antifungal properties. A study highlighted the effectiveness of various natural alkaloids against fungal pathogens, suggesting that cuscohygrine could play a role in developing antifungal agents. The exact mechanisms remain under investigation, but it is hypothesized that these compounds may disrupt fungal cell membranes or interfere with metabolic pathways essential for fungal growth .
Potential Antiviral Activity
Recent docking studies have proposed that cuscohygrine may possess antiviral properties, particularly against SARS-CoV-2. This potential is attributed to its interaction with viral proteins, although direct empirical evidence is still lacking. The need for further studies to validate these findings and explore the antiviral mechanisms of cuscohygrine is evident .
Pharmacokinetics and Detection
Cuscohygrine has been studied for its pharmacokinetic properties, particularly in the context of coca leaf consumption. Analytical methods such as liquid chromatography-tandem mass spectrometry (LC-MS/MS) have been developed to detect cuscohygrine in biological matrices like oral fluid. These studies demonstrate that cuscohygrine can be reliably detected up to three hours after consumption of coca tea, which has implications for forensic toxicology and drug testing protocols .
Case Study: Coca Leaf Consumption
A study involving volunteers consuming coca tea revealed that cuscohygrine levels could be detected in oral fluid samples using optimized solid-phase extraction methods. This research underscores the importance of cuscohygrine as a biomarker in differentiating legal coca use from illicit cocaine consumption. The findings suggest a need for routine analyses in forensic settings to monitor coca alkaloids effectively .
Comparative Analysis of Alkaloids
Table 1 summarizes the biological activities and characteristics of several key alkaloids found in Erythroxylum coca, including cuscohygrine:
Alkaloid | Antifungal Activity | Antiviral Activity | Forensic Marker | Notes |
---|---|---|---|---|
Cuscohygrine | Yes | Proposed | Yes | Detected in oral fluids |
Hygrine | Yes | Limited evidence | Yes | Similar detection capabilities |
Cocaine | Limited | Not applicable | Yes | Major psychoactive component |
Future Directions in Research
The potential health benefits of cuscohygrine are still largely unexplored. Future research should focus on:
- In vitro and in vivo studies : To establish the efficacy of cuscohygrine against specific pathogens.
- Mechanistic studies : To understand how cuscohygrine interacts at the molecular level with viral and fungal targets.
- Clinical trials : To assess safety and efficacy in humans, particularly regarding its antiviral potential.
Eigenschaften
IUPAC Name |
1,3-bis(1-methylpyrrolidin-2-yl)propan-2-one | |
---|---|---|
Details | Computed by Lexichem TK 2.7.0 (PubChem release 2021.05.07) | |
Source | PubChem | |
URL | https://pubchem.ncbi.nlm.nih.gov | |
Description | Data deposited in or computed by PubChem | |
InChI |
InChI=1S/C13H24N2O/c1-14-7-3-5-11(14)9-13(16)10-12-6-4-8-15(12)2/h11-12H,3-10H2,1-2H3 | |
Details | Computed by InChI 1.0.6 (PubChem release 2021.05.07) | |
Source | PubChem | |
URL | https://pubchem.ncbi.nlm.nih.gov | |
Description | Data deposited in or computed by PubChem | |
InChI Key |
ZEBIACKKLGVLFZ-UHFFFAOYSA-N | |
Details | Computed by InChI 1.0.6 (PubChem release 2021.05.07) | |
Source | PubChem | |
URL | https://pubchem.ncbi.nlm.nih.gov | |
Description | Data deposited in or computed by PubChem | |
Canonical SMILES |
CN1CCCC1CC(=O)CC2CCCN2C | |
Details | Computed by OEChem 2.3.0 (PubChem release 2021.05.07) | |
Source | PubChem | |
URL | https://pubchem.ncbi.nlm.nih.gov | |
Description | Data deposited in or computed by PubChem | |
Molecular Formula |
C13H24N2O | |
Details | Computed by PubChem 2.1 (PubChem release 2021.05.07) | |
Source | PubChem | |
URL | https://pubchem.ncbi.nlm.nih.gov | |
Description | Data deposited in or computed by PubChem | |
Molecular Weight |
224.34 g/mol | |
Details | Computed by PubChem 2.1 (PubChem release 2021.05.07) | |
Source | PubChem | |
URL | https://pubchem.ncbi.nlm.nih.gov | |
Description | Data deposited in or computed by PubChem | |
Physical Description |
Solid | |
Record name | Cuscohygrine | |
Source | Human Metabolome Database (HMDB) | |
URL | http://www.hmdb.ca/metabolites/HMDB0030290 | |
Description | The Human Metabolome Database (HMDB) is a freely available electronic database containing detailed information about small molecule metabolites found in the human body. | |
Explanation | HMDB is offered to the public as a freely available resource. Use and re-distribution of the data, in whole or in part, for commercial purposes requires explicit permission of the authors and explicit acknowledgment of the source material (HMDB) and the original publication (see the HMDB citing page). We ask that users who download significant portions of the database cite the HMDB paper in any resulting publications. | |
CAS No. |
454-14-8 | |
Record name | Cuscohygrine | |
Source | Human Metabolome Database (HMDB) | |
URL | http://www.hmdb.ca/metabolites/HMDB0030290 | |
Description | The Human Metabolome Database (HMDB) is a freely available electronic database containing detailed information about small molecule metabolites found in the human body. | |
Explanation | HMDB is offered to the public as a freely available resource. Use and re-distribution of the data, in whole or in part, for commercial purposes requires explicit permission of the authors and explicit acknowledgment of the source material (HMDB) and the original publication (see the HMDB citing page). We ask that users who download significant portions of the database cite the HMDB paper in any resulting publications. | |
Melting Point |
40 - 41 °C | |
Record name | Cuscohygrine | |
Source | Human Metabolome Database (HMDB) | |
URL | http://www.hmdb.ca/metabolites/HMDB0030290 | |
Description | The Human Metabolome Database (HMDB) is a freely available electronic database containing detailed information about small molecule metabolites found in the human body. | |
Explanation | HMDB is offered to the public as a freely available resource. Use and re-distribution of the data, in whole or in part, for commercial purposes requires explicit permission of the authors and explicit acknowledgment of the source material (HMDB) and the original publication (see the HMDB citing page). We ask that users who download significant portions of the database cite the HMDB paper in any resulting publications. | |
Retrosynthesis Analysis
AI-Powered Synthesis Planning: Our tool employs the Template_relevance Pistachio, Template_relevance Bkms_metabolic, Template_relevance Pistachio_ringbreaker, Template_relevance Reaxys, Template_relevance Reaxys_biocatalysis model, leveraging a vast database of chemical reactions to predict feasible synthetic routes.
One-Step Synthesis Focus: Specifically designed for one-step synthesis, it provides concise and direct routes for your target compounds, streamlining the synthesis process.
Accurate Predictions: Utilizing the extensive PISTACHIO, BKMS_METABOLIC, PISTACHIO_RINGBREAKER, REAXYS, REAXYS_BIOCATALYSIS database, our tool offers high-accuracy predictions, reflecting the latest in chemical research and data.
Strategy Settings
Precursor scoring | Relevance Heuristic |
---|---|
Min. plausibility | 0.01 |
Model | Template_relevance |
Template Set | Pistachio/Bkms_metabolic/Pistachio_ringbreaker/Reaxys/Reaxys_biocatalysis |
Top-N result to add to graph | 6 |
Feasible Synthetic Routes
Q1: What is the molecular formula and weight of cuscohygrine?
A1: Cuscohygrine has a molecular formula of C13H24N2O and a molecular weight of 224.34 g/mol.
Q2: Are there any spectroscopic data available to characterize cuscohygrine?
A2: Researchers often utilize techniques like gas chromatography-mass spectrometry (GC-MS) and high-performance liquid chromatography (HPLC) to analyze and quantify cuscohygrine in plant extracts and biological samples [, , , , , , , ]. Additionally, infrared (IR) spectroscopy can be used to confirm the presence of characteristic functional groups within the molecule [].
Q3: How is cuscohygrine synthesized in plants?
A3: Cuscohygrine biosynthesis originates from the amino acid ornithine. Studies have shown that hygrine, another pyrrolidine alkaloid, serves as the direct precursor to cuscohygrine in plants like Scopolia lurida []. The incorporation of ornithine into the pyrrolidine ring structure of cuscohygrine aligns with the established biogenetic pathways for other related alkaloids [].
Q4: In which plants is cuscohygrine primarily found?
A4: Cuscohygrine is primarily found in plants belonging to the Solanaceae and Erythroxylaceae families. Some notable examples include:
- Erythroxylum coca: Coca leaves, known for their cocaine content, also contain cuscohygrine as a minor alkaloid [, , , , ].
- Atropa belladonna: This plant, also known as deadly nightshade, contains various tropane alkaloids, including cuscohygrine [, ].
- Datura species: Datura plants, known for their psychoactive properties, have also been found to contain cuscohygrine [, ].
- Scopolia species: Like Atropa belladonna, Scopolia species are a source of tropane alkaloids and contain cuscohygrine [, ].
- Convolvulus species: Certain bindweed species like Convolvulus arvensis and Convolvulus lanatus have been reported to contain cuscohygrine [, ].
Q5: How does the content of cuscohygrine vary within a plant?
A5: Research on Erythroxylum coca indicates that the distribution of cuscohygrine, along with other alkaloids like cocaine and methyl ecgonine, is not uniform within the leaf. The highest concentrations of these alkaloids are generally found in the lamina periphery [].
Q6: What are the potential pharmacological applications of cuscohygrine?
A6: While research on cuscohygrine's pharmacological properties is ongoing, some studies suggest potential applications:
- Neurodegenerative disorders: Computational studies have explored the potential of cuscohygrine and other alkaloids from Withania somnifera as inhibitors against targets implicated in neurodegenerative diseases like Alzheimer's disease [, ].
- Acetylcholinesterase inhibition: Research suggests that extracts from Datura metel, which contain cuscohygrine, exhibit acetylcholinesterase inhibitory activity. This property has led to investigations into its potential for managing Alzheimer's disease [].
Q7: Have there been any in vitro or in vivo studies on cuscohygrine's efficacy?
A7: While in silico studies provide valuable insights, further research, including cell-based assays and animal models, is needed to validate these findings and comprehensively evaluate cuscohygrine's efficacy for specific therapeutic applications.
Q8: What are the commonly used analytical methods for the detection and quantification of cuscohygrine?
A8: Researchers utilize various analytical techniques to identify and quantify cuscohygrine:
- Gas Chromatography-Mass Spectrometry (GC-MS): This technique allows for separating, identifying, and quantifying cuscohygrine based on its mass-to-charge ratio [, , , ].
- High-Performance Liquid Chromatography (HPLC): HPLC coupled with UV detection or mass spectrometry provides a sensitive method for analyzing cuscohygrine in complex mixtures like plant extracts [, , , , , ].
- High-Performance Thin-Layer Chromatography (HPTLC): HPTLC, often coupled with densitometry, is a versatile technique used for both qualitative and quantitative analysis of cuscohygrine, particularly in plant materials and formulations [, , ].
Q9: Have these analytical methods been validated for cuscohygrine analysis?
A10: The development and validation of reliable analytical methods are crucial for accurately assessing cuscohygrine levels in various matrices. Researchers have emphasized the importance of validating methods for analyzing coca leaf alkaloids, including cuscohygrine, in biological samples like oral fluid []. This validation process typically involves evaluating parameters like accuracy, precision, specificity, linearity, and limits of detection and quantification to ensure the method's suitability for the intended purpose.
Haftungsausschluss und Informationen zu In-Vitro-Forschungsprodukten
Bitte beachten Sie, dass alle Artikel und Produktinformationen, die auf BenchChem präsentiert werden, ausschließlich zu Informationszwecken bestimmt sind. Die auf BenchChem zum Kauf angebotenen Produkte sind speziell für In-vitro-Studien konzipiert, die außerhalb lebender Organismen durchgeführt werden. In-vitro-Studien, abgeleitet von dem lateinischen Begriff "in Glas", beinhalten Experimente, die in kontrollierten Laborumgebungen unter Verwendung von Zellen oder Geweben durchgeführt werden. Es ist wichtig zu beachten, dass diese Produkte nicht als Arzneimittel oder Medikamente eingestuft sind und keine Zulassung der FDA für die Vorbeugung, Behandlung oder Heilung von medizinischen Zuständen, Beschwerden oder Krankheiten erhalten haben. Wir müssen betonen, dass jede Form der körperlichen Einführung dieser Produkte in Menschen oder Tiere gesetzlich strikt untersagt ist. Es ist unerlässlich, sich an diese Richtlinien zu halten, um die Einhaltung rechtlicher und ethischer Standards in Forschung und Experiment zu gewährleisten.