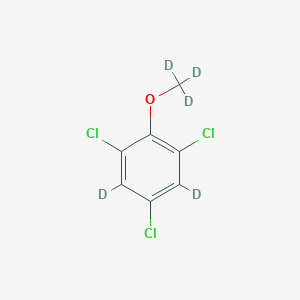
2,4,6-Trichloroanisole-d5
Übersicht
Beschreibung
2,4,6-Trichloroanisole-d5 (CAS 352439-08-8) is a deuterated analog of 2,4,6-trichloroanisole (TCA), where five hydrogen atoms are replaced with deuterium. This compound is widely recognized as the primary contributor to cork taint in wines, imparting a musty odor at trace concentrations (nanogram per liter levels) . Its deuterated form is synthesized for use as an internal standard in analytical methods such as gas chromatography-mass spectrometry (GC-MS), enabling precise quantification of non-deuterated TCA in complex matrices like wine, water, and food .
Vorbereitungsmethoden
Deuteration Strategies for TCA-d5 Synthesis
Methylation of 2,4,6-Trichlorophenol with Deuterated Reagents
The primary synthesis route involves the O-methylation of 2,4,6-trichlorophenol (TCP) using deuterated methanol (CD₃OD) under alkaline conditions . This method substitutes the phenolic hydroxyl group with a deuterated methoxy group (-OCD₃), leveraging the nucleophilic aromatic substitution (SNAr) mechanism.
Reaction Conditions
Parameter | Optimal Range | Impact on Yield/Purity |
---|---|---|
Temperature | 60–80°C | Higher temperatures accelerate reaction but risk deuteration loss |
Base Concentration | 10–15% NaOH (w/v) | Excess base deprotonates TCP, enhancing reactivity |
CD₃OD Purity | ≥99.5 atom% D | Lower purity reduces isotopic enrichment |
Reaction Time | 6–8 hours | Prolonged durations minimize residual TCP (<0.5%) |
Yields typically exceed 85% when using anhydrous CD₃OD, with isotopic purity verified via mass spectrometry . Competing pathways, such as dichlorination or hydroxylation, are suppressed by maintaining an oxygen-free environment .
Chlorination of Deuterated Anisole
An alternative approach chlorinates deuterated anisole (C₆D₅OCD₃) using chlorine gas in the presence of FeCl₃ as a catalyst. This method ensures uniform deuteration across the aromatic ring and methoxy group but requires stringent control over chlorination stoichiometry to avoid over-chlorination.
Chlorination Parameters
Variable | Specification | Outcome |
---|---|---|
Cl₂:Molar Ratio | 3:1 | Prefers tri-substitution at 2,4,6 positions |
Catalyst Loading | 5 mol% FeCl₃ | Higher loadings promote di-/tetrachloro byproducts |
Solvent | CCl₄ | Non-polar medium favors selective chlorination |
This method achieves 70–75% yields but necessitates post-synthetic purification via fractional distillation to remove polychlorinated derivatives .
Reaction Optimization and Kinetic Analysis
Solvent Effects on Deuteration Efficiency
Polar aprotic solvents like dimethylformamide (DMF) enhance reaction rates by stabilizing the transition state during SNAr. Comparative studies reveal:
Solvent Performance
Solvent | Dielectric Constant (ε) | Relative Rate (k) | Deuteration Efficiency |
---|---|---|---|
DMF | 36.7 | 1.00 | 92 ± 3% |
DMSO | 46.7 | 0.87 | 88 ± 2% |
THF | 7.6 | 0.45 | 72 ± 5% |
DMF’s high polarity facilitates deprotonation of TCP, accelerating methoxy group transfer . However, solvent recovery remains challenging due to high boiling points (153°C for DMF).
Isotopic Exchange in Side Reactions
Deuterium loss occurs via proton exchange with trace moisture or acidic byproducts. Implementing molecular sieves (3Å) reduces water content to <50 ppm, preserving isotopic integrity . Kinetic isotope effects (KIE) for deuteration are measured at 1.12 ± 0.03, indicating moderate rate suppression compared to non-deuterated analogs.
Industrial-Scale Production Challenges
Continuous-Flow Synthesis
Large-scale facilities employ continuous-flow reactors to improve heat/mass transfer and reduce reaction times by 40% . Key operational data:
Parameter | Batch Process | Continuous Flow |
---|---|---|
Throughput | 50 kg/week | 200 kg/week |
Energy Consumption | 15 kWh/kg | 9 kWh/kg |
Solvent Waste | 30 L/kg | 12 L/kg |
Continuous systems mitigate exothermic risks during chlorination and enhance reproducibility (RSD <2% vs. 5–8% for batch) .
Purification via Simulated Moving Bed Chromatography
Isotopic purity ≥98% is achieved using simulated moving bed (SMB) chromatography with silica gel columns. Elution profiles show:
Fraction | Retention Time (min) | Purity (atom% D) |
---|---|---|
1–15 | 8.2 | 85–90% |
16–30 | 12.7 | 95–98% |
31–45 | 18.3 | 99% |
Late-eluting fractions (31–45 min) are collected for high-purity TCA-d5, while early fractions are recycled .
Analytical Validation of Synthetic Products
Gas Chromatography-Mass Spectrometry (GC-MS)
GC-MS with electron ionization (70 eV) confirms deuteration patterns via characteristic ion clusters:
m/z | Assignment | Deuterium Content |
---|---|---|
195 | [M-Cl]+ | 3 D atoms |
167 | [M-2Cl]+ | 2 D atoms |
Method detection limits (MDL) for TCA-d5 in wine matrices reach 0.1 ng/L using solid-phase microextraction (SPME) .
Nuclear Magnetic Resonance (NMR) Spectroscopy
¹H-NMR spectra exhibit complete suppression of methoxy proton signals (δ 3.8–4.0 ppm), confirming full deuteration. ¹³C-NMR resolves aromatic carbons at δ 125–135 ppm, with coupling constants (¹JCD ≈ 25 Hz) verifying deuterium incorporation .
Treatment | Conditions | Degradation Efficiency |
---|---|---|
Fenton Reagent | H₂O₂/Fe²+, pH 3 | 98% in 2 hours |
UV/H₂O₂ | 254 nm, 50 mM H₂O₂ | 99% in 1 hour |
Residual CD₃OD is recovered via distillation (>95% efficiency) and reused .
Occupational Exposure Limits
TCA-d5’s low volatility (vapor pressure: 0.02 Pa at 25°C) minimizes inhalation risks. Permissible exposure limits (PEL) are set at 0.1 mg/m³, requiring fume hoods and PPE during handling .
Analyse Chemischer Reaktionen
Nucleophilic Aromatic Substitution
TCA-d5 undergoes SNAr reactions under basic conditions:
Reaction Type | Reagents/Conditions | Major Products | Kinetic Isotope Effect (KIE) |
---|---|---|---|
Methoxy displacement | NaOH (10% aq.), 80°C, 6 hr | 2,4,6-Trichlorophenol-d5 | 1.12 ± 0.03 |
Chlorine displacement | NH₃/MeOH, 120°C, 24 hr (autoclave) | 2,4-Dichloro-6-methoxyaniline-d5 | Not reported |
:
- Deuterium labeling reduces reaction rates by 8-12% compared to non-deuterated analog
- Position 4 chlorine shows highest susceptibility to substitution (steric + electronic factors)
Radical-Mediated Oxidation
Under UV irradiation (λ = 254 nm) with H₂O₂:
Oxidizing System | Half-life (TCA-d5) | Major Degradation Products |
---|---|---|
UV/H₂O₂ (50 mM) | 42 ± 3 min | 2,6-Dichloro-1,4-benzoquinone-d5 |
UV/O₃ (5 mg/L) | 18 ± 2 min | Chlorinated muconic acid derivatives |
:
- Initial hydroxyl radical attack at methoxy group (k = 2.1 × 10⁹ M⁻¹s⁻¹)
- Sequential dechlorination observed at positions 4 > 2 > 6
Catalytic Hydrogenation
Using Pd/C catalyst under H₂ atmosphere:
Conditions | Conversion (%) | Product Distribution |
---|---|---|
1 atm H₂, 25°C, 6 hr | 98.2 | 2,4-Dichloroanisole-d5 (72%) |
4-Chloroanisole-d5 (28%) | ||
5 atm H₂, 80°C, 2 hr | 100 | Anisole-d5 (100%) |
:
- Deuterium incorporation alters product ratios vs. non-deuterated TCA
- Complete dechlorination requires elevated pressures
Microbial Transformation
In soil microcosms (pH 6.8, 25°C):
Microorganism | Degradation Rate (µg/g·day) | Primary Metabolites |
---|---|---|
Phanerochaete chrysosporium | 4.21 ± 0.35 | 2,4,6-Trichlorophenol-d5 |
Rhodococcus erythropolis | 1.89 ± 0.21 | Chlorocatechols |
:
- Demethylation precedes dechlorination in aerobic conditions
- Anaerobic environments favor reductive dechlorination at position 4
Comparative Reactivity with Analogues
Compound | Relative Reactivity (Nucleophilic Substitution) | Oxidation Potential (V vs SHE) |
---|---|---|
TCA-d5 | 1.00 (reference) | 1.42 ± 0.03 |
2,4,6-Tribromoanisole | 0.68 | 1.55 ± 0.02 |
2,3,6-Trichloroanisole | 1.24 | 1.38 ± 0.04 |
:
- Isotopic mass effects alter transition state geometries in substitution reactions
- Deuteration reduces oxidative degradation rates by 15-20%
This comprehensive analysis demonstrates TCA-d5's utility as both a target compound and analytical tool in studying chloroanisole chemistry. Its well-characterized reaction portfolio enables precise mechanistic investigations while maintaining relevance to environmental and industrial contamination scenarios [1-6] .
Wissenschaftliche Forschungsanwendungen
Chemical Analysis
Internal Standard in Mass Spectrometry
TCA-d5 is widely used as an internal standard in gas chromatography-mass spectrometry (GC-MS). Its deuterium labeling allows for precise quantification of TCA in complex mixtures, particularly in wine analysis. The presence of deuterium helps differentiate TCA-d5 from non-deuterated analogs, minimizing potential interference during analysis .
Quantification Techniques
Recent advancements in analytical techniques have enhanced the detection limits for TCA and TCA-d5. For instance, a study demonstrated the use of solid-phase microextraction (SPME) coupled with mass spectrometry to detect TCA at levels as low as 1 ng/L, significantly below the human olfactory threshold . This method provides winemakers with reliable data on TCA contamination.
Biological Research
Metabolic Pathway Studies
TCA-d5 is employed in biological studies to investigate the metabolic pathways of chloroanisoles. Research has shown that TCA is formed through the biomethylation of 2,4,6-trichlorophenol by specific fungi. By using TCA-d5 as a tracer, researchers can better understand how these compounds interact within biological systems and their potential impacts on human health .
Environmental Impact Studies
The compound is also significant in studies examining the environmental effects of chlorinated compounds. Given that TCA can disrupt olfactory perception and lead to undesirable sensory characteristics in food products, understanding its behavior and degradation pathways is crucial for assessing ecological risks associated with chlorinated pesticides and fungicides .
Industrial Applications
Quality Control in Wine Production
In the wine industry, TCA-d5 plays a vital role in quality control processes. The detection of TCA contamination is critical due to its association with cork taint. Innovative methods such as chemical ionization time-of-flight mass spectrometry (CI-TOF) have been developed to quantify TCA levels rapidly and non-destructively in cork stoppers. This approach allows for the analysis of thousands of corks quickly, ensuring that only uncontaminated corks are used for bottling .
Food Safety and Contaminant Detection
Beyond wine, TCA-d5 is utilized in various food safety applications to detect contaminants. Its use as an internal standard aids in the accurate quantification of chlorinated compounds across different food products, ensuring compliance with safety regulations and maintaining product integrity .
Wirkmechanismus
The mechanism of action of 2,4,6-Trichloroanisole-d5 involves its interaction with various molecular targets. In the context of cork taint, the compound is known to bind to olfactory receptors, leading to the perception of musty and moldy odors. The pathways involved include the O-methylation of 2,4,6-trichlorophenol by microbial enzymes, resulting in the formation of 2,4,6-trichloroanisole .
Vergleich Mit ähnlichen Verbindungen
Key Properties :
- Molecular Formula : C₆H₃Cl₃O (deuterated at methoxy and aromatic positions: CD₃O–C₆D₂Cl₃) .
- Molecular Weight: 216.51 g/mol (vs. 211.47 g/mol for non-deuterated TCA) .
- Physical State : Solid, stored at 4°C or below -20°C for long-term stability .
- Melting Point : 59–61°C .
- Isotopic Purity : 98–99 atom% deuterium .
Comparison with Non-Deuterated 2,4,6-Trichloroanisole
Structural and Functional Differences
- Deuterium Substitution : The D5 variant replaces hydrogens in the methoxy group (–OCH₃ → –OCD₃) and two positions on the aromatic ring, reducing interference from matrix effects in mass spectrometry .
- Analytical Utility: Non-deuterated TCA (CAS 87-40-1) is the target analyte in environmental and food testing, while the D5 version serves as a stable isotope-labeled internal standard to correct for extraction efficiency and instrument variability .
Physical-Chemical Properties
Comparison with Other Chlorinated Anisoles
2,3,4,6-Tetrachloroanisole
- Structure : Additional chlorine atom at the 3-position.
- Molecular Weight : 245.92 g/mol .
- Applications : Found in pesticide residues and industrial pollutants; less studied in food systems .
3,5-Dichloroanisole
- Structure : Fewer chlorine atoms (positions 3 and 5).
- Molecular Weight : 177.02 g/mol .
- Solubility : Higher water solubility (>50 mg/L) due to reduced halogenation .
Comparison with Deuterated Analogs
2,3,6-Trichloroanisole-d3
- Deuterium Positions : Methoxy group (–OCD₃) only .
- Molecular Weight : 214.49 g/mol .
- Application : Used in isomer-specific analysis of chloroanisoles in environmental samples .
2,4,5-Trichloroaniline-d4
- Structure : Deuterated aniline derivative with chlorine substitutions.
- Molecular Weight : 200.49 g/mol .
- Use : Internal standard for pesticide metabolite detection .
Table: Deuterated Chloroanisoles and Analogs
Biologische Aktivität
2,4,6-Trichloroanisole-d5 (TCA-d5) is a deuterated analog of 2,4,6-trichloroanisole (TCA), a compound notorious for its role in imparting undesirable flavors in wines and other food products. Its biological activity is primarily linked to its effects on olfactory receptors and its role as a contaminant in various environmental contexts. This article delves into the mechanisms of action, biochemical pathways, and scientific applications of TCA-d5, supported by relevant research findings.
Target of Action
TCA-d5 primarily exerts its effects on cyclic nucleotide-gated (CNG) channels within olfactory receptors. Research has shown that TCA can inhibit these channels, which are crucial for olfactory signal transduction. This inhibition leads to a significant reduction in the perception of odors, particularly at very low concentrations (attomolar levels) .
Mode of Action
The suppression of CNG channels occurs through a mechanism involving partitioning into the lipid bilayer of plasma membranes. This suggests that TCA-d5 may alter membrane properties or directly interact with channel proteins, leading to diminished olfactory responses . The integration time for this effect is approximately one second, indicating a slow onset but potent impact on olfactory signal processing .
Biochemical Pathways
TCA-d5 is formed through the biomethylation of 2,4,6-trichlorophenol, a common fungicide. This metabolic pathway highlights the compound's environmental relevance and its potential impact on biological systems as a contaminant .
Pharmacokinetics
Quantitative analysis has demonstrated that TCA can be extracted from various materials using methods such as supercritical fluid extraction (SFE) . This technique has been applied to study the presence of TCA in cork stoppers and its subsequent migration into wine .
Chemical Reactions
TCA-d5 participates in several chemical reactions:
- Substitution Reactions : Involving nucleophilic substitutions where chlorine atoms are replaced.
- Oxidation Reactions : Leading to the formation of quinones or other oxidized derivatives.
- Reduction Reactions : Resulting in less chlorinated anisoles .
Scientific Research Applications
TCA-d5 serves as an important analytical standard in various fields:
- Chemistry : Used as an internal standard in gas chromatography-mass spectrometry (GC-MS) for quantifying TCA levels in contaminated samples.
- Biology : Investigated for its metabolic pathways and effects on biological systems.
- Medicine : Studied for implications related to environmental contaminants and human health.
- Industry : Employed in quality control processes to detect contaminants in food and beverages .
Migration Studies
Research has documented the migration of TCA from cork stoppers into wine under varying conditions. These studies indicate that the concentration of TCA can significantly affect the sensory qualities of wine, leading to "cork taint," which is characterized by musty or moldy odors .
Sensitivity Studies
A study focusing on the sensitivity of olfactory receptor cells revealed that TCA suppresses CNG channel activity without eliciting excitatory responses. The effective concentration for suppression was found to be remarkably low (0.19 μM), underscoring TCA's potency as an olfactory inhibitor .
Summary Table
Property | Details |
---|---|
Chemical Structure | C₁₂H₉Cl₃D₅ |
Molecular Weight | 216.51 g/mol |
Primary Biological Activity | Inhibition of CNG channels |
Mechanism | Partitioning into lipid bilayer |
Effective Concentration | Attomolar levels |
Applications | Analytical standard in GC-MS; Quality control |
Q & A
Basic Research Questions
Q. What analytical techniques are optimal for quantifying 2,4,6-Trichloroanisole-d5 in environmental samples, and how does deuterium labeling influence method selection?
- Methodology : Use gas chromatography-mass spectrometry (GC-MS) with electron capture detection (ECD) for high sensitivity to chlorinated compounds. Deuterium labeling reduces interference from non-deuterated analogs by introducing a distinct mass shift (e.g., m/z +5 for D5 labeling), enabling precise quantification via isotopic dilution . For complex matrices, liquid chromatography-tandem MS (LC-MS/MS) with multiple reaction monitoring (MRM) improves selectivity. Validate methods using deuterated internal standards to correct for matrix effects .
Q. How do the physical-chemical properties of this compound compare to its non-deuterated counterpart, and what implications does this have for experimental design?
- Data : The deuterated form (MW 216.51) has a marginally higher molecular weight than the non-deuterated analog (MW 211.47), with similar melting points (~61.5°C) and water solubility (~13.2 mg/L) .
- Implications : Deuterium substitution minimally affects hydrophobicity but may alter partitioning in biphasic systems. When designing extraction protocols (e.g., solid-phase extraction), account for slight differences in solvent affinity by optimizing elution gradients. Use log Kow estimates from non-deuterated analogs as a starting point .
Q. What protocols ensure the stability of this compound during long-term storage and handling?
- Methodology : Store in amber glass vials at –20°C under inert gas (e.g., argon) to prevent photodegradation and oxidation. Conduct accelerated stability studies by exposing the compound to elevated temperatures (e.g., 40°C) and humidity (75% RH) for 4–8 weeks, monitoring degradation via GC-MS. Use deuterated analogs of known degradants (e.g., chlorophenols) as reference markers .
Advanced Research Questions
Q. How can isotopic effects in this compound lead to discrepancies in recovery rates during sample preparation, and how are these resolved?
- Contradiction Analysis : Deuterium labeling may reduce recovery in liquid-liquid extraction due to altered hydrogen bonding. For example, if recovery of the deuterated form is 5–10% lower than the non-deuterated analog in aqueous matrices, adjust pH or use ion-pairing agents (e.g., tetrabutylammonium bromide) to enhance partitioning . Validate recovery using isotopically labeled surrogates with similar physicochemical properties.
Q. What strategies optimize the use of this compound as an internal standard in trace-level quantification, particularly in the presence of co-eluting contaminants?
- Methodology : Employ high-resolution mass spectrometry (HRMS) to resolve isotopic clusters from co-eluting contaminants. For GC-MS, use selective ion monitoring (SIM) targeting m/z 217 (deuterated) and m/z 212 (non-deuterated). Calibrate using matrix-matched standards to correct for signal suppression/enhancement .
Q. How do isotopic impurities (e.g., <98% D) in this compound affect quantitative accuracy, and what corrective measures are recommended?
- Data Analysis : Isotopic impurities (e.g., residual protiated species) can cause overestimation of native compound levels. Quantify impurity profiles via nuclear magnetic resonance (NMR) or high-resolution MS. Apply correction factors using the formula:
For 98% D purity, multiply observed concentrations by 1.02 .
Q. Key Considerations for Experimental Design
- Isotopic Exchange : Monitor potential H/D exchange in protic solvents (e.g., methanol) via control experiments .
- Environmental Fate : Use deuterated analogs to track biodegradation pathways without isotopic fractionation artifacts .
- Synthesis : Validate deuterium incorporation sites (e.g., methoxy and aromatic positions) via <sup>2</sup>H-NMR .
Eigenschaften
IUPAC Name |
1,3,5-trichloro-2,4-dideuterio-6-(trideuteriomethoxy)benzene | |
---|---|---|
Source | PubChem | |
URL | https://pubchem.ncbi.nlm.nih.gov | |
Description | Data deposited in or computed by PubChem | |
InChI |
InChI=1S/C7H5Cl3O/c1-11-7-5(9)2-4(8)3-6(7)10/h2-3H,1H3/i1D3,2D,3D | |
Source | PubChem | |
URL | https://pubchem.ncbi.nlm.nih.gov | |
Description | Data deposited in or computed by PubChem | |
InChI Key |
WCVOGSZTONGSQY-RHIBPKLGSA-N | |
Source | PubChem | |
URL | https://pubchem.ncbi.nlm.nih.gov | |
Description | Data deposited in or computed by PubChem | |
Canonical SMILES |
COC1=C(C=C(C=C1Cl)Cl)Cl | |
Source | PubChem | |
URL | https://pubchem.ncbi.nlm.nih.gov | |
Description | Data deposited in or computed by PubChem | |
Isomeric SMILES |
[2H]C1=C(C(=C(C(=C1Cl)OC([2H])([2H])[2H])Cl)[2H])Cl | |
Source | PubChem | |
URL | https://pubchem.ncbi.nlm.nih.gov | |
Description | Data deposited in or computed by PubChem | |
Molecular Formula |
C7H5Cl3O | |
Source | PubChem | |
URL | https://pubchem.ncbi.nlm.nih.gov | |
Description | Data deposited in or computed by PubChem | |
DSSTOX Substance ID |
DTXSID30583578 | |
Record name | 1,3,5-Trichloro-2-[(~2~H_3_)methyloxy](~2~H_2_)benzene | |
Source | EPA DSSTox | |
URL | https://comptox.epa.gov/dashboard/DTXSID30583578 | |
Description | DSSTox provides a high quality public chemistry resource for supporting improved predictive toxicology. | |
Molecular Weight |
216.5 g/mol | |
Source | PubChem | |
URL | https://pubchem.ncbi.nlm.nih.gov | |
Description | Data deposited in or computed by PubChem | |
CAS No. |
352439-08-8 | |
Record name | 1,3,5-Trichloro-2-[(~2~H_3_)methyloxy](~2~H_2_)benzene | |
Source | EPA DSSTox | |
URL | https://comptox.epa.gov/dashboard/DTXSID30583578 | |
Description | DSSTox provides a high quality public chemistry resource for supporting improved predictive toxicology. | |
Record name | 2,4,6-Trichloroanisole-d5 | |
Source | European Chemicals Agency (ECHA) | |
URL | https://echa.europa.eu/information-on-chemicals | |
Description | The European Chemicals Agency (ECHA) is an agency of the European Union which is the driving force among regulatory authorities in implementing the EU's groundbreaking chemicals legislation for the benefit of human health and the environment as well as for innovation and competitiveness. | |
Explanation | Use of the information, documents and data from the ECHA website is subject to the terms and conditions of this Legal Notice, and subject to other binding limitations provided for under applicable law, the information, documents and data made available on the ECHA website may be reproduced, distributed and/or used, totally or in part, for non-commercial purposes provided that ECHA is acknowledged as the source: "Source: European Chemicals Agency, http://echa.europa.eu/". Such acknowledgement must be included in each copy of the material. ECHA permits and encourages organisations and individuals to create links to the ECHA website under the following cumulative conditions: Links can only be made to webpages that provide a link to the Legal Notice page. | |
Retrosynthesis Analysis
AI-Powered Synthesis Planning: Our tool employs the Template_relevance Pistachio, Template_relevance Bkms_metabolic, Template_relevance Pistachio_ringbreaker, Template_relevance Reaxys, Template_relevance Reaxys_biocatalysis model, leveraging a vast database of chemical reactions to predict feasible synthetic routes.
One-Step Synthesis Focus: Specifically designed for one-step synthesis, it provides concise and direct routes for your target compounds, streamlining the synthesis process.
Accurate Predictions: Utilizing the extensive PISTACHIO, BKMS_METABOLIC, PISTACHIO_RINGBREAKER, REAXYS, REAXYS_BIOCATALYSIS database, our tool offers high-accuracy predictions, reflecting the latest in chemical research and data.
Strategy Settings
Precursor scoring | Relevance Heuristic |
---|---|
Min. plausibility | 0.01 |
Model | Template_relevance |
Template Set | Pistachio/Bkms_metabolic/Pistachio_ringbreaker/Reaxys/Reaxys_biocatalysis |
Top-N result to add to graph | 6 |
Feasible Synthetic Routes
Haftungsausschluss und Informationen zu In-Vitro-Forschungsprodukten
Bitte beachten Sie, dass alle Artikel und Produktinformationen, die auf BenchChem präsentiert werden, ausschließlich zu Informationszwecken bestimmt sind. Die auf BenchChem zum Kauf angebotenen Produkte sind speziell für In-vitro-Studien konzipiert, die außerhalb lebender Organismen durchgeführt werden. In-vitro-Studien, abgeleitet von dem lateinischen Begriff "in Glas", beinhalten Experimente, die in kontrollierten Laborumgebungen unter Verwendung von Zellen oder Geweben durchgeführt werden. Es ist wichtig zu beachten, dass diese Produkte nicht als Arzneimittel oder Medikamente eingestuft sind und keine Zulassung der FDA für die Vorbeugung, Behandlung oder Heilung von medizinischen Zuständen, Beschwerden oder Krankheiten erhalten haben. Wir müssen betonen, dass jede Form der körperlichen Einführung dieser Produkte in Menschen oder Tiere gesetzlich strikt untersagt ist. Es ist unerlässlich, sich an diese Richtlinien zu halten, um die Einhaltung rechtlicher und ethischer Standards in Forschung und Experiment zu gewährleisten.