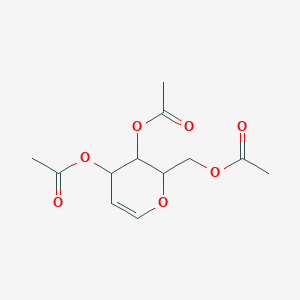
Tri-O-Acetyl-D-Glucal
Übersicht
Beschreibung
It is a white to off-white crystalline powder with the molecular formula C12H16O7 and a molecular weight of 272.25 g/mol . This compound is soluble in chloroform and ethanol but insoluble in water . Tri-O-acetyl-D-glucal is an important building block in organic synthesis, particularly in the synthesis of oligosaccharides and other complex carbohydrates .
Wissenschaftliche Forschungsanwendungen
Tri-O-acetyl-D-glucal has a wide range of applications in scientific research:
Wirkmechanismus
Target of Action
Tri-O-acetyl-D-glucal, also known as 3,4,6-tri-O-Acetyl-D-glucal, is primarily used as a building block in the synthesis of oligosaccharides . It is a derivative of D-(+)-glucose and is used in the synthesis of a variety of compounds .
Mode of Action
This compound can undergo a Ferrier rearrangement with an alcohol and Lewis acid to give a 2,3-unsaturated product . This rearrangement is facilitated by user-friendly, eco- and environmental-friendly catalysts such as Cu(OTf)2 or Fe(OTf)3 . The catalysts play a dual role in activating the glycal donor through promoting ring-opening of the intramolecularly incorporated donor–acceptor cyclopropane (DAC) of CCBz and directing the following α-face-preferential nucleophilic attack of the incoming acceptor mediated by H-bond interactions .
Biochemical Pathways
The compound is involved in the synthesis of cyclic ketene acetals and the enantioselective synthesis of spliceostatin A and thailanstatin A derivatives with spliceosome inhibitory activity . It is also used in the synthesis of 2,3-unsaturated glycosides .
Pharmacokinetics
It is known to be sparingly soluble in dmso and ethanol, and insoluble in water . This could potentially affect its absorption, distribution, metabolism, and excretion (ADME) properties, and thus its bioavailability.
Result of Action
The result of this compound’s action is the production of a variety of compounds, including cyclic ketene acetals and spliceostatin A and thailanstatin A derivatives . These compounds have various applications, including spliceosome inhibitory activity .
Action Environment
The action of this compound is influenced by environmental factors such as temperature and the presence of catalysts . For example, the compound’s rearrangement is facilitated by catalysts such as Cu(OTf)2 or Fe(OTf)3 . Additionally, its solubility in different solvents could affect its stability and efficacy .
Safety and Hazards
When handling Tri-O-acetyl-D-glucal, it is advised to avoid dust formation and breathing mist, gas, or vapors. Contact with skin and eyes should be avoided. Use personal protective equipment and ensure adequate ventilation. Remove all sources of ignition and evacuate personnel to safe areas. Keep people away from and upwind of spill/leak .
Zukünftige Richtungen
Tri-O-acetyl-D-glucal has been used in various research studies and its potential applications are vast. For instance, it has been used in the synthesis of a 16-membered macrolactone natural product (−)-A26771B , and in the synthesis of poly(lactic acid) materials through copolymerisation . It has also been used in the synthesis of ω-aminoalkyl 2-deoxy- and 2,3-dideoxy-d-hexopyranosides . These studies suggest that this compound could have more potential applications in the future.
Biochemische Analyse
Biochemical Properties
Tri-O-acetyl-D-glucal plays a significant role in biochemical reactions. It is used as a building block for the synthesis of a range of carbohydrates . The glucal double bond in the compound allows other functional groups to be introduced
Molecular Mechanism
It is known that as an alkene, a glycal like this compound can undergo electrophilic addition across the double bond to introduce halogens and epoxides or be used to generate deoxy monosaccharides
Vorbereitungsmethoden
Tri-O-acetyl-D-glucal can be synthesized from D-glucose pentaacetate through a series of chemical reactions. One common method involves the treatment of D-glucose pentaacetate with acetic anhydride and a catalyst such as sulfuric acid . The reaction conditions typically include heating the mixture to a specific temperature and then purifying the product through recrystallization . Industrial production methods may involve similar steps but on a larger scale, with optimizations for yield and purity .
Analyse Chemischer Reaktionen
Tri-O-acetyl-D-glucal undergoes various chemical reactions, including:
Electrophilic Addition: As an alkene, it can undergo electrophilic addition reactions to introduce halogens and epoxides.
Ferrier Rearrangement: This reaction involves the use of an alcohol and a Lewis acid to produce a 2,3-unsaturated product.
Glycosylation: It is extensively used as a glycosylating agent in the synthesis of 2-deoxy sugars and oligosaccharides.
Common reagents used in these reactions include halogens, alcohols, and Lewis acids. The major products formed from these reactions are often derivatives of the original compound, such as 2-deoxy-D-glucose .
Vergleich Mit ähnlichen Verbindungen
Tri-O-acetyl-D-glucal is unique due to its high reactivity and versatility as a building block in organic synthesis. Similar compounds include:
- 1,2-Dideoxy-3,4,6-tri-O-acetyl-D-arabino-1-hexenopyranose
- 3,4,6-Tri-O-acetyl-1,5-anhydro-2-deoxy-D-arabino-hex-1-enitol
These compounds share similar structural features and reactivity but may differ in their specific applications and the types of products they form.
Eigenschaften
IUPAC Name |
(3,4-diacetyloxy-3,4-dihydro-2H-pyran-2-yl)methyl acetate | |
---|---|---|
Details | Computed by LexiChem 2.6.6 (PubChem release 2019.06.18) | |
Source | PubChem | |
URL | https://pubchem.ncbi.nlm.nih.gov | |
Description | Data deposited in or computed by PubChem | |
InChI |
InChI=1S/C12H16O7/c1-7(13)17-6-11-12(19-9(3)15)10(4-5-16-11)18-8(2)14/h4-5,10-12H,6H2,1-3H3 | |
Details | Computed by InChI 1.0.5 (PubChem release 2019.06.18) | |
Source | PubChem | |
URL | https://pubchem.ncbi.nlm.nih.gov | |
Description | Data deposited in or computed by PubChem | |
InChI Key |
LLPWGHLVUPBSLP-UHFFFAOYSA-N | |
Details | Computed by InChI 1.0.5 (PubChem release 2019.06.18) | |
Source | PubChem | |
URL | https://pubchem.ncbi.nlm.nih.gov | |
Description | Data deposited in or computed by PubChem | |
Canonical SMILES |
CC(=O)OCC1C(C(C=CO1)OC(=O)C)OC(=O)C | |
Details | Computed by OEChem 2.1.5 (PubChem release 2019.06.18) | |
Source | PubChem | |
URL | https://pubchem.ncbi.nlm.nih.gov | |
Description | Data deposited in or computed by PubChem | |
Molecular Formula |
C12H16O7 | |
Details | Computed by PubChem 2.1 (PubChem release 2019.06.18) | |
Source | PubChem | |
URL | https://pubchem.ncbi.nlm.nih.gov | |
Description | Data deposited in or computed by PubChem | |
DSSTOX Substance ID |
DTXSID90863045 | |
Record name | 1,3,4-Tri-O-acetyl-2,6-anhydro-5-deoxyhex-5-enitol | |
Source | EPA DSSTox | |
URL | https://comptox.epa.gov/dashboard/DTXSID90863045 | |
Description | DSSTox provides a high quality public chemistry resource for supporting improved predictive toxicology. | |
Molecular Weight |
272.25 g/mol | |
Details | Computed by PubChem 2.1 (PubChem release 2021.05.07) | |
Source | PubChem | |
URL | https://pubchem.ncbi.nlm.nih.gov | |
Description | Data deposited in or computed by PubChem | |
CAS No. |
2873-29-2, 4098-06-0 | |
Record name | 3,4,6-Tri-O-acetylglucal | |
Source | ChemIDplus | |
URL | https://pubchem.ncbi.nlm.nih.gov/substance/?source=chemidplus&sourceid=0002873292 | |
Description | ChemIDplus is a free, web search system that provides access to the structure and nomenclature authority files used for the identification of chemical substances cited in National Library of Medicine (NLM) databases, including the TOXNET system. | |
Record name | 2,6-Anhydro-5-deoxy-D-arabino-hex-5-enitol triacetate | |
Source | ChemIDplus | |
URL | https://pubchem.ncbi.nlm.nih.gov/substance/?source=chemidplus&sourceid=0004098060 | |
Description | ChemIDplus is a free, web search system that provides access to the structure and nomenclature authority files used for the identification of chemical substances cited in National Library of Medicine (NLM) databases, including the TOXNET system. | |
Record name | 2,6-anhydro-5-deoxy-D-arabino-hex-5-enitol triacetate | |
Source | European Chemicals Agency (ECHA) | |
URL | https://echa.europa.eu/substance-information/-/substanceinfo/100.021.690 | |
Description | The European Chemicals Agency (ECHA) is an agency of the European Union which is the driving force among regulatory authorities in implementing the EU's groundbreaking chemicals legislation for the benefit of human health and the environment as well as for innovation and competitiveness. | |
Explanation | Use of the information, documents and data from the ECHA website is subject to the terms and conditions of this Legal Notice, and subject to other binding limitations provided for under applicable law, the information, documents and data made available on the ECHA website may be reproduced, distributed and/or used, totally or in part, for non-commercial purposes provided that ECHA is acknowledged as the source: "Source: European Chemicals Agency, http://echa.europa.eu/". Such acknowledgement must be included in each copy of the material. ECHA permits and encourages organisations and individuals to create links to the ECHA website under the following cumulative conditions: Links can only be made to webpages that provide a link to the Legal Notice page. | |
Record name | 1,5-anhydro-2-deoxy-D-arabino-hex-1-enitol triacetate | |
Source | European Chemicals Agency (ECHA) | |
URL | https://echa.europa.eu/substance-information/-/substanceinfo/100.018.827 | |
Description | The European Chemicals Agency (ECHA) is an agency of the European Union which is the driving force among regulatory authorities in implementing the EU's groundbreaking chemicals legislation for the benefit of human health and the environment as well as for innovation and competitiveness. | |
Explanation | Use of the information, documents and data from the ECHA website is subject to the terms and conditions of this Legal Notice, and subject to other binding limitations provided for under applicable law, the information, documents and data made available on the ECHA website may be reproduced, distributed and/or used, totally or in part, for non-commercial purposes provided that ECHA is acknowledged as the source: "Source: European Chemicals Agency, http://echa.europa.eu/". Such acknowledgement must be included in each copy of the material. ECHA permits and encourages organisations and individuals to create links to the ECHA website under the following cumulative conditions: Links can only be made to webpages that provide a link to the Legal Notice page. | |
Retrosynthesis Analysis
AI-Powered Synthesis Planning: Our tool employs the Template_relevance Pistachio, Template_relevance Bkms_metabolic, Template_relevance Pistachio_ringbreaker, Template_relevance Reaxys, Template_relevance Reaxys_biocatalysis model, leveraging a vast database of chemical reactions to predict feasible synthetic routes.
One-Step Synthesis Focus: Specifically designed for one-step synthesis, it provides concise and direct routes for your target compounds, streamlining the synthesis process.
Accurate Predictions: Utilizing the extensive PISTACHIO, BKMS_METABOLIC, PISTACHIO_RINGBREAKER, REAXYS, REAXYS_BIOCATALYSIS database, our tool offers high-accuracy predictions, reflecting the latest in chemical research and data.
Strategy Settings
Precursor scoring | Relevance Heuristic |
---|---|
Min. plausibility | 0.01 |
Model | Template_relevance |
Template Set | Pistachio/Bkms_metabolic/Pistachio_ringbreaker/Reaxys/Reaxys_biocatalysis |
Top-N result to add to graph | 6 |
Feasible Synthetic Routes
Q1: What is the molecular formula and weight of Tri-O-acetyl-D-glucal?
A1: this compound has the molecular formula C12H16O8 and a molecular weight of 288.25 g/mol.
Q2: Are there any notable spectroscopic characteristics of this compound?
A2: While specific spectroscopic data isn't extensively detailed in the provided abstracts, the presence of characteristic peaks in 1H NMR and 13C NMR spectra can help confirm the structure and purity of the compound. For instance, the anomeric configuration of synthesized 2-deoxy-α-glycosides can be assigned by NMR analysis of their dihydroderivatives. []
Q3: How is this compound employed in organic synthesis?
A3: this compound serves as a key starting material for synthesizing various carbohydrate derivatives, particularly deoxy sugars and 2,3-unsaturated glycosides. Its reactivity stems from the presence of the glycal double bond and the three acetyl protecting groups. [, , , , , , , , , , , ]
Q4: What is the Ferrier rearrangement, and how does it apply to this compound?
A4: The Ferrier rearrangement is a key reaction involving this compound. It involves the Lewis acid-catalyzed rearrangement of glycals, such as this compound, to 2,3-unsaturated glycosides in the presence of alcohols or other nucleophiles. [, , , , , , , , , , , ]
Q5: Can you elaborate on the regio- and stereoselectivity observed in reactions involving this compound?
A5: Reactions involving this compound often exhibit high regio- and stereoselectivity. For instance, hydroformylation reactions primarily yield 2-formyl derivatives, indicating regioselectivity. Additionally, the Ferrier rearrangement frequently favors the formation of α-anomers over β-anomers, showcasing stereoselectivity. [, , ]
Q6: What are some examples of Lewis acid catalysts used in Ferrier rearrangements with this compound?
A6: Various Lewis acids effectively catalyze the Ferrier rearrangement of this compound. Examples include boron trifluoride diethyl etherate (BF3•OEt2), indium(III) chloride (InCl3), zinc chloride (ZnCl2), Copper(II) Triflate (Cu(OTf)2) and scandium(III) trifluoromethanesulfonate [Sc(OTf)3]. [, , , , ]
Q7: Beyond the Ferrier rearrangement, what other reactions can this compound undergo?
A7: Apart from the Ferrier rearrangement, this compound participates in diverse reactions, including photochemical additions with lactonitrile, [, , ] halogenation reactions, [, , ] and ring-contraction reactions with hydrogen fluoride. []
Q8: How is this compound used in the synthesis of biologically relevant molecules?
A8: this compound acts as a starting point for synthesizing complex natural products and biologically active compounds. It is used in the synthesis of D-allosamine derivatives, [] exo-brevicomin, [] (-)-Balanol [] and the D-, E-, F-, and I-ring parts of ciguatoxin. [] Its versatility allows for the construction of various structural motifs present in these molecules.
Q9: Has computational chemistry been applied to studying this compound?
A11: While the abstracts don't delve into detailed computational studies, computational tools likely play a role in understanding the reaction mechanisms, transition states, and stereochemical outcomes of reactions involving this compound, as demonstrated by the computational analysis in the reaction with triazolinediones. []
Q10: How does the structure of this compound influence its reactivity and applications?
A12: The presence of the double bond in the glycal moiety and the three acetyl protecting groups are crucial for its reactivity and applications. The double bond enables participation in reactions like the Ferrier rearrangement and electrophilic additions. The acetyl groups serve as protecting groups, allowing for selective transformations at other positions in the molecule. Modifications to the structure, such as different protecting groups or substitutions on the ring, can significantly influence its reactivity and applications. [, , ]
Q11: What analytical methods are commonly employed to characterize and quantify this compound?
A13: Common analytical techniques like NMR spectroscopy, mass spectrometry, and chromatography are likely employed for characterization and quantification. []
Haftungsausschluss und Informationen zu In-Vitro-Forschungsprodukten
Bitte beachten Sie, dass alle Artikel und Produktinformationen, die auf BenchChem präsentiert werden, ausschließlich zu Informationszwecken bestimmt sind. Die auf BenchChem zum Kauf angebotenen Produkte sind speziell für In-vitro-Studien konzipiert, die außerhalb lebender Organismen durchgeführt werden. In-vitro-Studien, abgeleitet von dem lateinischen Begriff "in Glas", beinhalten Experimente, die in kontrollierten Laborumgebungen unter Verwendung von Zellen oder Geweben durchgeführt werden. Es ist wichtig zu beachten, dass diese Produkte nicht als Arzneimittel oder Medikamente eingestuft sind und keine Zulassung der FDA für die Vorbeugung, Behandlung oder Heilung von medizinischen Zuständen, Beschwerden oder Krankheiten erhalten haben. Wir müssen betonen, dass jede Form der körperlichen Einführung dieser Produkte in Menschen oder Tiere gesetzlich strikt untersagt ist. Es ist unerlässlich, sich an diese Richtlinien zu halten, um die Einhaltung rechtlicher und ethischer Standards in Forschung und Experiment zu gewährleisten.