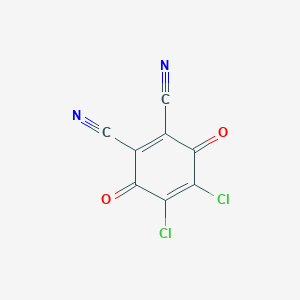
2,3-Dichlor-5,6-dicyan-1,4-benzochinon
Übersicht
Beschreibung
2,3-Dichloro-5,6-dicyanobenzoquinone is a chemical compound known for its strong oxidizing properties. It is a yellow to orange crystalline powder with the molecular formula C8Cl2N2O2 and a molar mass of 227.00 g/mol . This compound is widely used in organic chemistry due to its ability to facilitate various oxidative reactions.
Wissenschaftliche Forschungsanwendungen
2,3-Dichlor-5,6-dicyanobenzochinon hat eine breite Palette von Anwendungen in der wissenschaftlichen Forschung:
Chemie: Wird als Entschützungsreagenz für Thioacetale, Acetale und Ketale verwendet.
Industrie: Wird bei der Herstellung von hochwertigen Chemikalien und fortschrittlichen Materialien verwendet.
5. Wirkmechanismus
Der Wirkmechanismus von 2,3-Dichlor-5,6-dicyanobenzochinon beinhaltet die Entfernung von Wasserstoffatomen aus organischen Molekülen, was zur Bildung oxidierter Produkte führt. Dieser Prozess wird durch das hohe Reduktionspotenzial der Verbindung erleichtert, das es ihr ermöglicht, Elektronen vom Substrat aufzunehmen . Das resultierende Hydrochinon ist in typischen Reaktionslösungsmitteln schwer löslich, was die Aufarbeitung erleichtert .
Ähnliche Verbindungen:
1,4-Benzochinon: Ein schwächeres Oxidationsmittel im Vergleich zu 2,3-Dichlor-5,6-dicyanobenzochinon.
Chloranil: Ein weiteres Chinonderivat mit ähnlichen oxidierenden Eigenschaften, aber geringerer Effizienz.
Einzigartigkeit: 2,3-Dichlor-5,6-dicyanobenzochinon ist aufgrund seines hohen Reduktionspotenzials und seiner Fähigkeit, eine breite Palette von Oxidationsreaktionen unter milden Bedingungen zu ermöglichen, einzigartig . Seine Vielseitigkeit und Effizienz machen es zu einem wertvollen Reagenz sowohl in der akademischen als auch in der industriellen Forschung .
Wirkmechanismus
Target of Action
DDQ is a chemical reagent that primarily targets organic molecules, particularly alcohols, phenols, and steroid ketones . It is used for the dehydrogenation of these compounds, effectively removing pairs of hydrogen atoms .
Mode of Action
DDQ operates through a mechanism of hydride transfer reactions . It has three accessible oxidation states: quinone (oxidized), semiquinone (one-electron-reduced), and hydroquinone (two-electron-reduced) . The stoichiometry of its action is illustrated by the conversion of tetralin to naphthalene .
Biochemical Pathways
DDQ is involved in the functionalization of activated C–H bonds and the dehydrogenation of saturated C–C, C–O, and C–N bonds . It is also used in oxidative couplings and cyclization reactions . The resulting hydroquinone is poorly soluble in typical reaction solvents, which facilitates workup .
Pharmacokinetics
It’s important to note that ddq decomposes in water but is stable in aqueous mineral acid .
Result of Action
The action of DDQ results in the dehydrogenation of target molecules, effectively removing pairs of hydrogen atoms . This can lead to the formation of new compounds, such as the conversion of tetralin to naphthalene . It is also used in the synthesis of 1,2-benzisoxazoles .
Action Environment
DDQ’s action can be influenced by environmental factors. For instance, it decomposes in water, indicating that it is less stable and potentially less effective in aqueous environments . It is stable in aqueous mineral acid, suggesting that acidic conditions can preserve its reactivity .
Biochemische Analyse
Biochemical Properties
2,3-Dichloro-5,6-dicyano-1,4-benzoquinone is known to mediate hydride transfer reactions and shows three accessible oxidation states: quinone (oxidized), semiquinone (one-electron-reduced), and hydroquinone (two-electron-reduced) . It is used as a reagent for oxidative couplings and cyclization reactions
Cellular Effects
Given its role in oxidative reactions, it may influence cell function by altering redox states and potentially impacting cell signaling pathways, gene expression, and cellular metabolism .
Molecular Mechanism
The molecular mechanism of action of 2,3-Dichloro-5,6-dicyano-1,4-benzoquinone involves the removal of pairs of hydrogen atoms from organic molecules
Temporal Effects in Laboratory Settings
It is known that the compound decomposes in water but is stable in aqueous mineral acid . This suggests that its effects may change over time depending on the environmental conditions.
Metabolic Pathways
Given its role in oxidative reactions, it may interact with enzymes or cofactors involved in redox reactions .
Vorbereitungsmethoden
Synthetic Routes and Reaction Conditions: The synthesis of 2,3-dichloro-5,6-dicyanobenzoquinone typically involves the cyanation of chloranil. The process was first reported in 1906 by J. Thiele and F. Günther, involving a six-step preparation . A more efficient single-step chlorination from 2,3-dicyanohydroquinone was later developed in 1965 .
Industrial Production Methods: In industrial settings, 2,3-dichloro-5,6-dicyanobenzoquinone is produced by reacting 2,3-dicyanohydroquinone with hydrochloric acid and nitric acid at around 35°C. The reaction mixture is then filtered, washed with carbon tetrachloride, and dried to obtain the final product .
Analyse Chemischer Reaktionen
Reaktionstypen: 2,3-Dichlor-5,6-dicyanobenzochinon unterliegt verschiedenen Reaktionstypen, darunter:
Häufige Reagenzien und Bedingungen:
Oxidationsreaktionen: Häufig in Lösungsmitteln wie Benzol, Methanol, Essigsäure und Dioxan durchgeführt.
Substitutionsreaktionen: Oft in Gegenwart von Triphenylphosphin und Acetonitril durchgeführt.
Hauptprodukte:
Dehydrierungsprodukte: Hydrochinone und Naphthalinderivate.
Substitutionsprodukte: Verschiedene Nitrile und funktionalisierte organische Verbindungen.
Vergleich Mit ähnlichen Verbindungen
1,4-Benzoquinone: A weaker oxidant compared to 2,3-dichloro-5,6-dicyanobenzoquinone.
Chloranil: Another quinone derivative with similar oxidizing properties but lower efficiency.
Uniqueness: 2,3-Dichloro-5,6-dicyanobenzoquinone is unique due to its high reduction potential and ability to facilitate a wide range of oxidative reactions under mild conditions . Its versatility and efficiency make it a valuable reagent in both academic and industrial research .
Eigenschaften
IUPAC Name |
4,5-dichloro-3,6-dioxocyclohexa-1,4-diene-1,2-dicarbonitrile | |
---|---|---|
Source | PubChem | |
URL | https://pubchem.ncbi.nlm.nih.gov | |
Description | Data deposited in or computed by PubChem | |
InChI |
InChI=1S/C8Cl2N2O2/c9-5-6(10)8(14)4(2-12)3(1-11)7(5)13 | |
Source | PubChem | |
URL | https://pubchem.ncbi.nlm.nih.gov | |
Description | Data deposited in or computed by PubChem | |
InChI Key |
HZNVUJQVZSTENZ-UHFFFAOYSA-N | |
Source | PubChem | |
URL | https://pubchem.ncbi.nlm.nih.gov | |
Description | Data deposited in or computed by PubChem | |
Canonical SMILES |
C(#N)C1=C(C(=O)C(=C(C1=O)Cl)Cl)C#N | |
Source | PubChem | |
URL | https://pubchem.ncbi.nlm.nih.gov | |
Description | Data deposited in or computed by PubChem | |
Molecular Formula |
C8Cl2N2O2 | |
Source | PubChem | |
URL | https://pubchem.ncbi.nlm.nih.gov | |
Description | Data deposited in or computed by PubChem | |
DSSTOX Substance ID |
DTXSID7052577 | |
Record name | 2,3-Dichloro-5,6-dicyano-p-benzoquinone | |
Source | EPA DSSTox | |
URL | https://comptox.epa.gov/dashboard/DTXSID7052577 | |
Description | DSSTox provides a high quality public chemistry resource for supporting improved predictive toxicology. | |
Molecular Weight |
227.00 g/mol | |
Source | PubChem | |
URL | https://pubchem.ncbi.nlm.nih.gov | |
Description | Data deposited in or computed by PubChem | |
Physical Description |
Yellow to orange solid; [Merck Index] Dark yellow powder; [Alfa Aesar MSDS] | |
Record name | Dichlorodicyanobenzoquinone | |
Source | Haz-Map, Information on Hazardous Chemicals and Occupational Diseases | |
URL | https://haz-map.com/Agents/13765 | |
Description | Haz-Map® is an occupational health database designed for health and safety professionals and for consumers seeking information about the adverse effects of workplace exposures to chemical and biological agents. | |
Explanation | Copyright (c) 2022 Haz-Map(R). All rights reserved. Unless otherwise indicated, all materials from Haz-Map are copyrighted by Haz-Map(R). No part of these materials, either text or image may be used for any purpose other than for personal use. Therefore, reproduction, modification, storage in a retrieval system or retransmission, in any form or by any means, electronic, mechanical or otherwise, for reasons other than personal use, is strictly prohibited without prior written permission. | |
CAS No. |
84-58-2 | |
Record name | 2,3-Dichloro-5,6-dicyano-1,4-benzoquinone | |
Source | CAS Common Chemistry | |
URL | https://commonchemistry.cas.org/detail?cas_rn=84-58-2 | |
Description | CAS Common Chemistry is an open community resource for accessing chemical information. Nearly 500,000 chemical substances from CAS REGISTRY cover areas of community interest, including common and frequently regulated chemicals, and those relevant to high school and undergraduate chemistry classes. This chemical information, curated by our expert scientists, is provided in alignment with our mission as a division of the American Chemical Society. | |
Explanation | The data from CAS Common Chemistry is provided under a CC-BY-NC 4.0 license, unless otherwise stated. | |
Record name | Dichlorodicyanobenzoquinone | |
Source | ChemIDplus | |
URL | https://pubchem.ncbi.nlm.nih.gov/substance/?source=chemidplus&sourceid=0000084582 | |
Description | ChemIDplus is a free, web search system that provides access to the structure and nomenclature authority files used for the identification of chemical substances cited in National Library of Medicine (NLM) databases, including the TOXNET system. | |
Record name | 2,3-dichloro-5,6-dicyanobenzoquinone | |
Source | DrugBank | |
URL | https://www.drugbank.ca/drugs/DB15684 | |
Description | The DrugBank database is a unique bioinformatics and cheminformatics resource that combines detailed drug (i.e. chemical, pharmacological and pharmaceutical) data with comprehensive drug target (i.e. sequence, structure, and pathway) information. | |
Explanation | Creative Common's Attribution-NonCommercial 4.0 International License (http://creativecommons.org/licenses/by-nc/4.0/legalcode) | |
Record name | Dichlorodicyanoquinone | |
Source | DTP/NCI | |
URL | https://dtp.cancer.gov/dtpstandard/servlet/dwindex?searchtype=NSC&outputformat=html&searchlist=401087 | |
Description | The NCI Development Therapeutics Program (DTP) provides services and resources to the academic and private-sector research communities worldwide to facilitate the discovery and development of new cancer therapeutic agents. | |
Explanation | Unless otherwise indicated, all text within NCI products is free of copyright and may be reused without our permission. Credit the National Cancer Institute as the source. | |
Record name | 1,4-Cyclohexadiene-1,2-dicarbonitrile, 4,5-dichloro-3,6-dioxo- | |
Source | EPA Chemicals under the TSCA | |
URL | https://www.epa.gov/chemicals-under-tsca | |
Description | EPA Chemicals under the Toxic Substances Control Act (TSCA) collection contains information on chemicals and their regulations under TSCA, including non-confidential content from the TSCA Chemical Substance Inventory and Chemical Data Reporting. | |
Record name | 2,3-Dichloro-5,6-dicyano-p-benzoquinone | |
Source | EPA DSSTox | |
URL | https://comptox.epa.gov/dashboard/DTXSID7052577 | |
Description | DSSTox provides a high quality public chemistry resource for supporting improved predictive toxicology. | |
Record name | 4,5-dichloro-3,6-dioxocyclohexa-1,4-diene-1,2-dicarbonitrile | |
Source | European Chemicals Agency (ECHA) | |
URL | https://echa.europa.eu/substance-information/-/substanceinfo/100.001.402 | |
Description | The European Chemicals Agency (ECHA) is an agency of the European Union which is the driving force among regulatory authorities in implementing the EU's groundbreaking chemicals legislation for the benefit of human health and the environment as well as for innovation and competitiveness. | |
Explanation | Use of the information, documents and data from the ECHA website is subject to the terms and conditions of this Legal Notice, and subject to other binding limitations provided for under applicable law, the information, documents and data made available on the ECHA website may be reproduced, distributed and/or used, totally or in part, for non-commercial purposes provided that ECHA is acknowledged as the source: "Source: European Chemicals Agency, http://echa.europa.eu/". Such acknowledgement must be included in each copy of the material. ECHA permits and encourages organisations and individuals to create links to the ECHA website under the following cumulative conditions: Links can only be made to webpages that provide a link to the Legal Notice page. | |
Record name | 2,3-DICHLORO-5,6-DICYANOBENZOQUINONE | |
Source | FDA Global Substance Registration System (GSRS) | |
URL | https://gsrs.ncats.nih.gov/ginas/app/beta/substances/1H5KD39UH7 | |
Description | The FDA Global Substance Registration System (GSRS) enables the efficient and accurate exchange of information on what substances are in regulated products. Instead of relying on names, which vary across regulatory domains, countries, and regions, the GSRS knowledge base makes it possible for substances to be defined by standardized, scientific descriptions. | |
Explanation | Unless otherwise noted, the contents of the FDA website (www.fda.gov), both text and graphics, are not copyrighted. They are in the public domain and may be republished, reprinted and otherwise used freely by anyone without the need to obtain permission from FDA. Credit to the U.S. Food and Drug Administration as the source is appreciated but not required. | |
Retrosynthesis Analysis
AI-Powered Synthesis Planning: Our tool employs the Template_relevance Pistachio, Template_relevance Bkms_metabolic, Template_relevance Pistachio_ringbreaker, Template_relevance Reaxys, Template_relevance Reaxys_biocatalysis model, leveraging a vast database of chemical reactions to predict feasible synthetic routes.
One-Step Synthesis Focus: Specifically designed for one-step synthesis, it provides concise and direct routes for your target compounds, streamlining the synthesis process.
Accurate Predictions: Utilizing the extensive PISTACHIO, BKMS_METABOLIC, PISTACHIO_RINGBREAKER, REAXYS, REAXYS_BIOCATALYSIS database, our tool offers high-accuracy predictions, reflecting the latest in chemical research and data.
Strategy Settings
Precursor scoring | Relevance Heuristic |
---|---|
Min. plausibility | 0.01 |
Model | Template_relevance |
Template Set | Pistachio/Bkms_metabolic/Pistachio_ringbreaker/Reaxys/Reaxys_biocatalysis |
Top-N result to add to graph | 6 |
Feasible Synthetic Routes
Haftungsausschluss und Informationen zu In-Vitro-Forschungsprodukten
Bitte beachten Sie, dass alle Artikel und Produktinformationen, die auf BenchChem präsentiert werden, ausschließlich zu Informationszwecken bestimmt sind. Die auf BenchChem zum Kauf angebotenen Produkte sind speziell für In-vitro-Studien konzipiert, die außerhalb lebender Organismen durchgeführt werden. In-vitro-Studien, abgeleitet von dem lateinischen Begriff "in Glas", beinhalten Experimente, die in kontrollierten Laborumgebungen unter Verwendung von Zellen oder Geweben durchgeführt werden. Es ist wichtig zu beachten, dass diese Produkte nicht als Arzneimittel oder Medikamente eingestuft sind und keine Zulassung der FDA für die Vorbeugung, Behandlung oder Heilung von medizinischen Zuständen, Beschwerden oder Krankheiten erhalten haben. Wir müssen betonen, dass jede Form der körperlichen Einführung dieser Produkte in Menschen oder Tiere gesetzlich strikt untersagt ist. Es ist unerlässlich, sich an diese Richtlinien zu halten, um die Einhaltung rechtlicher und ethischer Standards in Forschung und Experiment zu gewährleisten.