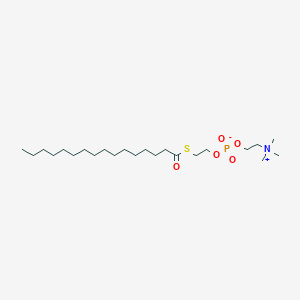
2-Hexadecanoylthio-1-ethylphosphorylcholine
Übersicht
Beschreibung
2-hexadecanoylthio-1-ethylphosphorylcholine is a member of phosphocholines.
Wirkmechanismus
Target of Action
HePC, also known as Thioglycollecithin or 2-Hexadecanoylthio-1-ethylphosphorylcholine, primarily targets the Hepatitis C Virus (HCV) . The main targets of the direct-acting antiviral (DAA) agents, like HePC, are the HCV-encoded proteins that are vital to the replication of the virus .
Mode of Action
HePC interacts with its targets, the HCV-encoded proteins, resulting in disruption of viral replication and infection . This interaction is a part of the mechanism of action of direct-acting antivirals (DAAs), which are medications targeted at specific steps within the HCV life cycle .
Biochemical Pathways
The biochemical pathways affected by HePC involve the replication process of the HCV. The compound disrupts the replication of the virus by targeting specific nonstructural proteins of the virus . This disruption affects the life cycle of the virus, preventing it from multiplying and spreading .
Pharmacokinetics
The pharmacokinetics of similar direct-acting antivirals (daas) used in treating hcv infections have been studied . These DAAs have clinical pharmacokinetic characteristics that describe their absorption, distribution, metabolism, and excretion .
Result of Action
The result of HePC’s action is the disruption of HCV replication, leading to a decrease in the viral load. This disruption can lead to a sustained virologic response, which is associated with lower all-cause mortality and improvements in hepatic and extrahepatic manifestations, cognitive function, physical health, work productivity, and quality of life .
Action Environment
The action, efficacy, and stability of HePC can be influenced by various environmental factors. For instance, the spread of HCV, which HePC targets, is primarily through blood contact . Therefore, behaviors that can spread the virus, such as sharing needles or other paraphernalia used for injection drug use, can influence the action and efficacy of HePC . Furthermore, the presence of other infections or co-morbidities can also impact the effectiveness of HePC .
Biochemische Analyse
Biochemical Properties
2-Hexadecanoylthio-1-ethylphosphorylcholine is a substrate for Type II phospholipase A2 (PLA2) enzymes, such as porcine pancreatic, bee venom, and snake venom PLA2 . This interaction with PLA2 enzymes suggests that this compound plays a role in the hydrolysis of the sn-2 position of phospholipids, a key step in the production of lysophospholipids and free fatty acids .
Cellular Effects
Its role as a substrate for PLA2 enzymes suggests that it may influence cell function through its involvement in lipid metabolism .
Molecular Mechanism
This compound exerts its effects at the molecular level primarily through its interactions with PLA2 enzymes. As a substrate for these enzymes, it participates in the hydrolysis of phospholipids at the sn-2 position .
Metabolic Pathways
This compound is involved in the metabolic pathway of phospholipid hydrolysis, where it serves as a substrate for PLA2 enzymes . The enzymes and cofactors it interacts with in this pathway include PLA2 enzymes such as porcine pancreatic, bee venom, and snake venom PLA2 .
Biologische Aktivität
2-Hexadecanoylthio-1-ethylphosphorylcholine (HEPC) is a phospholipid derivative that has garnered attention in biological research due to its unique properties and potential therapeutic applications. This article delves into the biological activity of HEPC, summarizing key findings from various studies, including its pharmacological effects, mechanisms of action, and potential clinical implications.
Overview of HEPC
HEPC is a synthetic compound that functions as a substrate for specific phospholipases and has been associated with various biological activities. Its structure consists of a long-chain fatty acid (hexadecanoyl), a thioether linkage, and a phosphorylcholine moiety, which contributes to its amphiphilic nature, enabling interactions with cellular membranes.
1. Inflammation Modulation
HEPC has been shown to influence inflammatory processes. In a study examining its effects on hyperalgesia induced by carrageenan injection in rats, HEPC demonstrated an effective dose (ED50) of 1.2 mg/kg without inhibiting cyclooxygenase (COX) activity or interfering with cannabinoid receptor signaling . This suggests that HEPC may modulate pain pathways without traditional anti-inflammatory side effects.
2. Apoptosis and Cancer Research
Research indicates that HEPC acts as a substrate for cytosolic phospholipase A2 (cPLA2), which is involved in apoptosis pathways. In vitro studies have shown that HEPC can influence TNF-α-induced apoptosis in mouse colonocytes, suggesting a potential role in cancer progression and treatment . The modulation of cPLA2 activity by HEPC may have implications for colorectal cancer therapies.
HEPC's biological activity is primarily mediated through its interaction with phospholipases and modulation of lipid signaling pathways. It has been identified as a specific substrate for sPLA2 enzymes, which are crucial in inflammatory responses and cellular signaling . The compound's ability to alter lipid metabolism could be leveraged for therapeutic strategies targeting inflammation and cancer.
Case Study 1: Mortality Risk Profiling in Staphylococcus aureus Bacteremia
In a comprehensive study involving over 200 individuals, HEPC was identified as one of the top-ranked metabolites associated with mortality risk in patients suffering from Staphylococcus aureus bacteremia. The study utilized metabolomics to analyze serum samples and found that elevated levels of HEPC correlated with increased mortality risk, highlighting its potential as a biomarker for severe infections .
Case Study 2: Exercise-Induced Bronchoconstriction
A clinical investigation into the effects of HEPC on exercise-induced bronchoconstriction (EIB) has shown promising results. Patients who continued to experience symptoms despite standard β2-agonist treatment were administered HEPC prior to exercise, demonstrating significant improvement in airway responsiveness . This suggests that HEPC may play a role in managing respiratory conditions.
Data Tables
Property | Value |
---|---|
Molecular Formula | C18H39NO3S |
Molecular Weight | 345.58 g/mol |
ED50 (Hyperalgesia) | 1.2 mg/kg |
Stability | ≥2 years at -20°C |
Purity | ≥98% |
Eigenschaften
IUPAC Name |
2-hexadecanoylsulfanylethyl 2-(trimethylazaniumyl)ethyl phosphate | |
---|---|---|
Source | PubChem | |
URL | https://pubchem.ncbi.nlm.nih.gov | |
Description | Data deposited in or computed by PubChem | |
InChI |
InChI=1S/C23H48NO5PS/c1-5-6-7-8-9-10-11-12-13-14-15-16-17-18-23(25)31-22-21-29-30(26,27)28-20-19-24(2,3)4/h5-22H2,1-4H3 | |
Source | PubChem | |
URL | https://pubchem.ncbi.nlm.nih.gov | |
Description | Data deposited in or computed by PubChem | |
InChI Key |
BDPQVGIMLZYZQA-UHFFFAOYSA-N | |
Source | PubChem | |
URL | https://pubchem.ncbi.nlm.nih.gov | |
Description | Data deposited in or computed by PubChem | |
Canonical SMILES |
CCCCCCCCCCCCCCCC(=O)SCCOP(=O)([O-])OCC[N+](C)(C)C | |
Source | PubChem | |
URL | https://pubchem.ncbi.nlm.nih.gov | |
Description | Data deposited in or computed by PubChem | |
Molecular Formula |
C23H48NO5PS | |
Source | PubChem | |
URL | https://pubchem.ncbi.nlm.nih.gov | |
Description | Data deposited in or computed by PubChem | |
DSSTOX Substance ID |
DTXSID30209625 | |
Record name | Thioglycollecithin | |
Source | EPA DSSTox | |
URL | https://comptox.epa.gov/dashboard/DTXSID30209625 | |
Description | DSSTox provides a high quality public chemistry resource for supporting improved predictive toxicology. | |
Molecular Weight |
481.7 g/mol | |
Source | PubChem | |
URL | https://pubchem.ncbi.nlm.nih.gov | |
Description | Data deposited in or computed by PubChem | |
CAS No. |
60793-01-3 | |
Record name | Thioglycollecithin | |
Source | ChemIDplus | |
URL | https://pubchem.ncbi.nlm.nih.gov/substance/?source=chemidplus&sourceid=0060793013 | |
Description | ChemIDplus is a free, web search system that provides access to the structure and nomenclature authority files used for the identification of chemical substances cited in National Library of Medicine (NLM) databases, including the TOXNET system. | |
Record name | Thioglycollecithin | |
Source | EPA DSSTox | |
URL | https://comptox.epa.gov/dashboard/DTXSID30209625 | |
Description | DSSTox provides a high quality public chemistry resource for supporting improved predictive toxicology. | |
Retrosynthesis Analysis
AI-Powered Synthesis Planning: Our tool employs the Template_relevance Pistachio, Template_relevance Bkms_metabolic, Template_relevance Pistachio_ringbreaker, Template_relevance Reaxys, Template_relevance Reaxys_biocatalysis model, leveraging a vast database of chemical reactions to predict feasible synthetic routes.
One-Step Synthesis Focus: Specifically designed for one-step synthesis, it provides concise and direct routes for your target compounds, streamlining the synthesis process.
Accurate Predictions: Utilizing the extensive PISTACHIO, BKMS_METABOLIC, PISTACHIO_RINGBREAKER, REAXYS, REAXYS_BIOCATALYSIS database, our tool offers high-accuracy predictions, reflecting the latest in chemical research and data.
Strategy Settings
Precursor scoring | Relevance Heuristic |
---|---|
Min. plausibility | 0.01 |
Model | Template_relevance |
Template Set | Pistachio/Bkms_metabolic/Pistachio_ringbreaker/Reaxys/Reaxys_biocatalysis |
Top-N result to add to graph | 6 |
Feasible Synthetic Routes
Q1: What is the main application of 2-Hexadecanoylthio-1-ethylphosphorylcholine described in the provided research paper?
A: The research article focuses on the synthesis of this compound and its application as a substrate for determining phospholipase A2 activity []. While the paper doesn't delve into the compound's therapeutic potential, its role in studying enzymatic activity is highlighted.
Haftungsausschluss und Informationen zu In-Vitro-Forschungsprodukten
Bitte beachten Sie, dass alle Artikel und Produktinformationen, die auf BenchChem präsentiert werden, ausschließlich zu Informationszwecken bestimmt sind. Die auf BenchChem zum Kauf angebotenen Produkte sind speziell für In-vitro-Studien konzipiert, die außerhalb lebender Organismen durchgeführt werden. In-vitro-Studien, abgeleitet von dem lateinischen Begriff "in Glas", beinhalten Experimente, die in kontrollierten Laborumgebungen unter Verwendung von Zellen oder Geweben durchgeführt werden. Es ist wichtig zu beachten, dass diese Produkte nicht als Arzneimittel oder Medikamente eingestuft sind und keine Zulassung der FDA für die Vorbeugung, Behandlung oder Heilung von medizinischen Zuständen, Beschwerden oder Krankheiten erhalten haben. Wir müssen betonen, dass jede Form der körperlichen Einführung dieser Produkte in Menschen oder Tiere gesetzlich strikt untersagt ist. Es ist unerlässlich, sich an diese Richtlinien zu halten, um die Einhaltung rechtlicher und ethischer Standards in Forschung und Experiment zu gewährleisten.