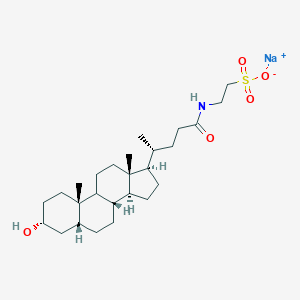
Taurolithocholic acid sodium salt
Übersicht
Beschreibung
Taurolithocholic acid sodium salt (TLCA) is a taurine-conjugated bile acid derived from lithocholic acid (LCA). Structurally, it consists of a steroidal backbone with a single hydroxyl group at the C3 position and a taurine moiety conjugated via an amide bond to the side chain . TLCA is endogenously produced in the liver through the conjugation of lithocholic acid with taurine, mediated by bile acid-CoA:amino acid N-acyltransferase (BAAT) .
TLCA has been implicated in pathological conditions such as chronic kidney disease (CKD), where its accumulation in serum correlates with renal dysfunction and inflammation . Notably, TLCA is a potent endogenous agonist of the G-protein bile acid receptor 1 (GPBAR1), influencing metabolic and inflammatory pathways .
Vorbereitungsmethoden
Chemical Conjugation Methods
EEDQ-Mediated Synthesis
The 2-ethoxy-1-ethoxycarbonyl-1,2-dihydroquinoline (EEDQ) method, adapted from sodium taurocholate synthesis , offers a scalable pathway for TLC-Na production. The process involves two stages:
Stage 1: Sodium Taurate Preparation
Taurine is reacted with sodium hydroxide (NaOH) in aqueous medium at 40–100°C for 30 minutes. Optimal molar ratios (taurine:NaOH = 1:0.9–1.1) yield sodium taurate with >95% purity after vacuum drying .
Stage 2: Condensation with Lithocholic Acid
Sodium taurate (1 eq), lithocholic acid (1–1.15 eq), and EEDQ (1.1–1.15 eq) are combined in polar solvents (e.g., N,N-dimethylformamide) at 80–120°C for 6–8 hours . The reaction proceeds via activation of lithocholic acid’s carboxyl group by EEDQ, forming an intermediate mixed anhydride that couples with sodium taurate’s amine (Fig. 1).
Reaction Conditions
Parameter | Optimal Range |
---|---|
Temperature | 80–120°C |
Solvent | N,N-dimethylformamide |
Molar Ratio (Taurate:Acid:EEDQ) | 1:1.1:1.1 |
Reaction Time | 6–8 hours |
This method achieves 70–85% yield, with residual EEDQ byproducts removed via ethanol precipitation .
DCC/DMAP Coupling Approach
Dicyclohexylcarbodiimide (DCC) and 4-dimethylaminopyridine (DMAP) provide an alternative pathway for amide bond formation. Lithocholic acid (1 eq) is dissolved in anhydrous dichloromethane, followed by DCC (1.2 eq) and DMAP (0.1 eq). After 1 hour activation, sodium taurate (1 eq) is added, and the mixture is stirred at 25°C for 24 hours. The dicyclohexylurea precipitate is filtered, and the product is extracted with sodium bicarbonate solution.
Advantages :
-
Mild conditions suitable for acid-sensitive substrates.
-
Higher selectivity with DMAP catalysis.
Limitations :
-
Lower yields (60–75%) due to competing side reactions.
-
Requires rigorous solvent drying.
Enzymatic Synthesis
Bile acid-CoA:amino acid N-acyltransferase (BAT) catalyzes the conjugation of taurine with lithocholic acid-CoA in hepatic mitochondria. In vitro enzymatic methods utilize recombinant BAT expressed in E. coli, with reaction parameters optimized as follows:
Parameter | Value |
---|---|
pH | 8.0–8.5 |
Temperature | 37°C |
Cofactor | ATP (2 mM) |
Reaction Time | 12–18 hours |
Yields range from 50–65%, with superior stereoselectivity but higher production costs .
Purification and Characterization
Isolation Techniques
Crude TLC-Na is purified via:
-
Ethanol Precipitation : Removes hydrophobic byproducts by dissolving the product in hot ethanol (80°C) and cooling to −20°C .
-
Column Chromatography : Silica gel (ethyl acetate:methanol:water = 7:2:1) resolves residual taurine and lithocholic acid.
Analytical Validation
-
HPLC : C18 column (acetonitrile:0.1% formic acid = 65:35), retention time = 8.2 min .
-
¹H NMR (D₂O): δ 0.65 (s, 3H, C18-CH₃), 3.40 (t, 2H, taurine-CH₂), 4.05 (m, 1H, C3-OH) .
Comparative Analysis of Synthesis Methods
Method | Yield (%) | Purity (%) | Cost (Relative) | Scalability |
---|---|---|---|---|
EEDQ-Mediated | 70–85 | 95–98 | Low | Industrial |
DCC/DMAP | 60–75 | 90–95 | Moderate | Lab-scale |
Enzymatic | 50–65 | 98–99 | High | Preclinical |
The EEDQ method balances cost and efficiency, making it preferable for bulk synthesis, while enzymatic routes suit high-purity applications .
Applications and Industrial Relevance
TLC-Na’s role in inducing cholestasis (75 µM) and apoptosis underscores the need for reliable synthesis . Pharmaceutical formulations require >98% purity, achievable via EEDQ protocols with gradient crystallization. Recent advancements in continuous-flow reactors may further enhance yield and reduce EEDQ waste .
Analyse Chemischer Reaktionen
Types of Reactions: Taurolithocholic acid sodium salt undergoes various chemical reactions, including:
Oxidation: This reaction can lead to the formation of oxidized derivatives of the compound.
Reduction: Reduction reactions can modify the functional groups present in the compound.
Substitution: Substitution reactions can occur at specific sites on the molecule, leading to the formation of different derivatives.
Common Reagents and Conditions:
Oxidation: Common oxidizing agents include potassium permanganate and hydrogen peroxide.
Reduction: Reducing agents such as sodium borohydride and lithium aluminum hydride are often used.
Substitution: Various halogenating agents and nucleophiles can be used for substitution reactions.
Major Products: The major products formed from these reactions depend on the specific conditions and reagents used. For example, oxidation can lead to the formation of hydroxylated derivatives, while reduction can yield deoxygenated products .
Wissenschaftliche Forschungsanwendungen
Biological Role and Mechanism of Action
Cholagogue and Choleretic Agent
TLCA serves as a cholagogue, promoting bile secretion, and as a choleretic agent, enhancing bile production. These properties are crucial for the emulsification of dietary fats and the absorption of fat-soluble vitamins. The compound is synthesized in the liver through the conjugation of lithocholic acid with taurine, facilitating its solubility and transport in the bile .
Induction of Apoptosis
Research has demonstrated that TLCA can induce apoptosis in hepatocytes at concentrations around 75 µM. This property is particularly useful for studying liver diseases, including hepatitis B, where TLCA has been shown to increase levels of apoptosis-related proteins such as caspase-3 and caspase-7 in Hep3B cells .
Cholestasis Studies
TLCA has been extensively used to model cholestasis, a condition characterized by impaired bile flow. In experimental settings, it has been effective in inducing cholestasis in both ex vivo and in vivo models. For instance, studies have utilized TLCA to investigate hepatocellular secretion disorders, providing insights into the mechanisms underlying bile salt transport and liver function .
Metabolic Investigations
The compound is also employed in metabolic studies to explore bile acid synthesis and metabolism. TLCA's role as a biomarker for various metabolic conditions is under investigation, particularly its serum concentration changes during oral lipid tolerance tests. Elevated levels of TLCA post-ingestion indicate its involvement in lipid metabolism and absorption processes .
Therapeutic Potential
There is emerging interest in TLCA's potential therapeutic applications due to its biological activities. Its ability to activate TGR5 receptors suggests a role in energy expenditure regulation, making it a candidate for obesity and metabolic syndrome research. Additionally, its effects on apoptosis may provide avenues for cancer research .
Case Studies and Experimental Findings
Wirkmechanismus
Taurolithocholic acid sodium salt primarily exerts its effects through its role in lipid digestion and absorption in the gastrointestinal tract. It interacts with bile acid receptors and transporters, influencing lipid metabolism, cholesterol homeostasis, and bile acid signaling pathways . The compound’s cholestatic effects are mediated through mechanisms involving calcium ion signaling and protein kinase C activation .
Vergleich Mit ähnlichen Verbindungen
Comparison with Similar Bile Acid Derivatives
Structural and Functional Differences
The table below summarizes key structural and functional distinctions between TLCA and related taurine-conjugated bile acids:
Key Research Findings
- Receptor Interactions : TLCA activates GPBAR1 with higher potency than TUDCA or TCA, making it a critical tool for studying TGR5/GPBAR1-mediated pathways . In contrast, TCDCA and TDCA are stronger activators of the farnesoid X receptor (FXR), regulating bile acid synthesis .
- Disease Associations: TLCA levels are elevated in CKD and correlate with uremic toxins like indoline-2-carboxylic acid, suggesting a role in renal pathology . TCA is widely used to induce acute pancreatitis in animal models, while TLCA 3-sulfate requires supraphysiological concentrations for similar effects, limiting its translational relevance .
Metabolic and Analytical Considerations
- Solubility : Sulfated derivatives like TLCA 3-sulfate exhibit improved water solubility, reducing their toxicity and altering receptor binding profiles .
- Analytical Detection : TLCA is quantified alongside TCA, TDCA, and TCDCA in LC-MS/MS assays, often using deuterated internal standards (e.g., [²H₄]-TLCA) to enhance accuracy .
Biologische Aktivität
Introduction
Taurolithocholic acid sodium salt (TLCA) is a conjugated bile acid derived from lithocholic acid, known for its significant role in various biological processes, including lipid metabolism, inflammation modulation, and cellular signaling. This article explores the biological activity of TLCA, focusing on its mechanisms of action, therapeutic potential, and relevant case studies.
TLCA exerts its biological effects primarily through two receptor-mediated pathways: the Takeda G-protein coupled receptor 5 (TGR5) and the farnesoid X receptor (FXR) . These receptors are involved in regulating inflammation, lipid metabolism, and cellular homeostasis.
TGR5 Pathway
- Phagocytosis Enhancement : TLCA has been shown to enhance the phagocytic activity of bone marrow-derived macrophages (BMDM) under inflammatory conditions. In a study, TLCA counteracted the inhibitory effects of lipopolysaccharides (LPS) on myelin clearance by increasing the expression of phagocytosis receptors such as CD36 and TREM2 .
- Anti-inflammatory Effects : Activation of TGR5 leads to the inhibition of NFκB, a key transcription factor in inflammatory responses. This signaling pathway promotes cytoprotection and reduces inflammation in various models .
FXR Pathway
- Lipid Metabolism Regulation : TLCA activates FXR, which plays a crucial role in maintaining bile acid homeostasis and regulating lipid metabolism. This activation can lead to improved metabolic profiles in conditions such as obesity and diabetes .
- Cholestasis Induction : TLCA has been used to induce cholestasis in animal models, allowing researchers to study liver function and drug-induced liver injury (DILI). Elevated serum levels of TLCA have been associated with cholestatic liver diseases .
In Vitro Studies
- Phagocytosis Assays : TLCA significantly improved myelin phagocytosis in BMDM exposed to inflammatory stimuli. The study demonstrated that TLCA's effects were mediated through TGR5 signaling pathways, highlighting its potential therapeutic role in neuroinflammatory conditions .
- UGT Enzyme Inhibition : TLCA was found to inhibit UDP-glucuronosyltransferase (UGT) enzymes, which are critical for drug metabolism. This inhibition suggests that TLCA may influence pharmacokinetics and drug interactions in clinical settings .
In Vivo Studies
- Cholestasis Models : In animal studies, TLCA has been utilized to model cholestasis, providing insights into the mechanisms underlying liver injury and recovery processes. The findings indicate that TLCA administration can exacerbate liver injury under certain pathological conditions .
Case Study 1: Neuroinflammation
A study investigated the effects of TLCA on neuroinflammation following spinal cord injury (SCI). The results indicated that TLCA treatment enhanced myelin clearance by modulating macrophage activity, suggesting its potential as a therapeutic agent for neurodegenerative diseases .
Case Study 2: Drug-Induced Liver Injury
In an analysis of drug-induced cholestasis using TLCA, researchers noted significant alterations in liver enzyme levels and histological changes indicative of cholestatic injury. These findings underscore the importance of assessing bile acid profiles in evaluating liver toxicity during drug development .
Summary Table of Biological Activities
Q & A
Basic Research Questions
Q. Q1. How can researchers confirm the identity and purity of taurolithocholic acid sodium salt in experimental preparations?
Methodological Answer:
- Synthesis Verification: Use thin-layer chromatography (TLC) with phase systems like Butanol 1 (butanol:acetic acid:water = 50:5:5) or Butanol 3 (butanol:Tris buffer:propionic acid = 50:9.25:0.75) to compare migration patterns against known standards .
- Purity Assessment: Employ nuclear magnetic resonance (NMR) spectroscopy to analyze structural integrity, focusing on characteristic peaks for the taurine-conjugated side chain and sulfate groups (if applicable) .
- Quantitative Analysis: High-performance liquid chromatography (HPLC) with UV detection at 210 nm can quantify impurities, ensuring >95% purity as per quality control standards .
Q. Q2. What experimental models are suitable for studying the solubility and stability of this compound in biological systems?
Methodological Answer:
- Solubility Profiling: Use in vitro assays with simulated intestinal or biliary fluids (e.g., pH 6.8 phosphate buffer with bile salts) to measure critical micellar concentration (CMC) .
- Stability Testing: Incubate the compound at physiological temperatures (37°C) and monitor degradation via mass spectrometry over 24–72 hours .
- Animal Models: Administer radiolabeled this compound in rodents to track biliary excretion and metabolic stability .
Advanced Research Questions
Q. Q3. How does this compound induce pancreatitis in mouse models, and how can researchers control for confounding factors?
Methodological Answer:
- Mechanistic Studies: Use bile duct cannulation to infuse this compound (e.g., 10 mM concentration) into the pancreatic duct of mice, monitoring inflammatory markers (IL-6, TNF-α) and histopathological changes .
- Control Strategies:
- Data Analysis: Apply multivariate regression to correlate dose, exposure time, and severity of pancreatitis .
Q. Q4. Why does this compound fail to induce ornithine decarboxylase (ODC) activity in gastric mucosa, unlike other bile acids?
Methodological Answer:
- Comparative Studies: Test structurally similar bile acids (e.g., taurodeoxycholic acid sodium salt) in parallel to identify functional group dependencies .
- Receptor Profiling: Use radioligand binding assays (e.g., with muscarinic M1 receptors) to assess whether this compound antagonizes signaling pathways linked to ODC induction .
- Gene Expression Analysis: Perform RNA sequencing on gastric mucosal cells to identify differential regulation of ODC-related genes (e.g., MYC, ODC1) .
Q. Q5. How can researchers resolve contradictions in data on this compound’s role in bile acid transport?
Methodological Answer:
- Model-Specific Validation: Compare transport kinetics in polarized cell lines (e.g., Caco-2 monolayers) versus primary hepatocytes to identify tissue-specific transporters (e.g., ASBT vs. NTCP) .
- Inhibitor Studies: Co-administer transporter inhibitors (e.g., cyclosporine A for P-glycoprotein) to isolate contributions of specific pathways .
- Metabolite Tracking: Use stable isotope-labeled this compound (e.g., deuterated analogs) to distinguish parent compound from sulfated or glucuronidated metabolites in LC-MS/MS workflows .
Q. Q6. What methodologies are recommended for studying this compound’s calcium channel agonism?
Methodological Answer:
- Electrophysiology: Perform patch-clamp experiments on isolated neurons or transfected HEK293 cells expressing calcium channels (e.g., T-type) to measure current modulation .
- Calcium Imaging: Use Fluo-4 AM dye in live-cell imaging to quantify intracellular Ca²⁺ flux upon compound exposure .
- Dose-Response Analysis: Construct concentration-response curves (1 nM–100 µM) to calculate EC₅₀ values and compare potency with known agonists (e.g., Bay K8644) .
Q. Q7. How should researchers design experiments to investigate this compound’s sulfation and its metabolic implications?
Methodological Answer:
- Sulfation Pathway Analysis: Incubate the compound with human liver microsomes or recombinant sulfotransferases (SULT2A1) to identify enzymatic activity .
- Metabolite Identification: Use solvolysis (acidified acetone, pH ≤1) followed by TLC or LC-MS to detect desulfated derivatives .
- In Vivo Relevance: Administer sulfated vs. non-sulfated forms in bile duct-ligated rodents to assess detoxification effects on hepatotoxicity .
Eigenschaften
IUPAC Name |
sodium;2-[[(4R)-4-[(3R,5R,8R,9S,10S,13R,14S,17R)-3-hydroxy-10,13-dimethyl-2,3,4,5,6,7,8,9,11,12,14,15,16,17-tetradecahydro-1H-cyclopenta[a]phenanthren-17-yl]pentanoyl]amino]ethanesulfonate | |
---|---|---|
Source | PubChem | |
URL | https://pubchem.ncbi.nlm.nih.gov | |
Description | Data deposited in or computed by PubChem | |
InChI |
InChI=1S/C26H45NO5S.Na/c1-17(4-9-24(29)27-14-15-33(30,31)32)21-7-8-22-20-6-5-18-16-19(28)10-12-25(18,2)23(20)11-13-26(21,22)3;/h17-23,28H,4-16H2,1-3H3,(H,27,29)(H,30,31,32);/q;+1/p-1/t17-,18-,19-,20+,21-,22+,23+,25+,26-;/m1./s1 | |
Source | PubChem | |
URL | https://pubchem.ncbi.nlm.nih.gov | |
Description | Data deposited in or computed by PubChem | |
InChI Key |
YAERYJYXPRIDTO-HRHHVWJRSA-M | |
Source | PubChem | |
URL | https://pubchem.ncbi.nlm.nih.gov | |
Description | Data deposited in or computed by PubChem | |
Canonical SMILES |
CC(CCC(=O)NCCS(=O)(=O)[O-])C1CCC2C1(CCC3C2CCC4C3(CCC(C4)O)C)C.[Na+] | |
Source | PubChem | |
URL | https://pubchem.ncbi.nlm.nih.gov | |
Description | Data deposited in or computed by PubChem | |
Isomeric SMILES |
C[C@H](CCC(=O)NCCS(=O)(=O)[O-])[C@H]1CC[C@@H]2[C@@]1(CC[C@H]3[C@H]2CC[C@H]4[C@@]3(CC[C@H](C4)O)C)C.[Na+] | |
Source | PubChem | |
URL | https://pubchem.ncbi.nlm.nih.gov | |
Description | Data deposited in or computed by PubChem | |
Molecular Formula |
C26H44NNaO5S | |
Source | PubChem | |
URL | https://pubchem.ncbi.nlm.nih.gov | |
Description | Data deposited in or computed by PubChem | |
DSSTOX Substance ID |
DTXSID701036211 | |
Record name | Sodium taurolithocholate | |
Source | EPA DSSTox | |
URL | https://comptox.epa.gov/dashboard/DTXSID701036211 | |
Description | DSSTox provides a high quality public chemistry resource for supporting improved predictive toxicology. | |
Molecular Weight |
505.7 g/mol | |
Source | PubChem | |
URL | https://pubchem.ncbi.nlm.nih.gov | |
Description | Data deposited in or computed by PubChem | |
CAS No. |
6042-32-6 | |
Record name | Sodium taurolithocholate | |
Source | EPA DSSTox | |
URL | https://comptox.epa.gov/dashboard/DTXSID701036211 | |
Description | DSSTox provides a high quality public chemistry resource for supporting improved predictive toxicology. | |
Retrosynthesis Analysis
AI-Powered Synthesis Planning: Our tool employs the Template_relevance Pistachio, Template_relevance Bkms_metabolic, Template_relevance Pistachio_ringbreaker, Template_relevance Reaxys, Template_relevance Reaxys_biocatalysis model, leveraging a vast database of chemical reactions to predict feasible synthetic routes.
One-Step Synthesis Focus: Specifically designed for one-step synthesis, it provides concise and direct routes for your target compounds, streamlining the synthesis process.
Accurate Predictions: Utilizing the extensive PISTACHIO, BKMS_METABOLIC, PISTACHIO_RINGBREAKER, REAXYS, REAXYS_BIOCATALYSIS database, our tool offers high-accuracy predictions, reflecting the latest in chemical research and data.
Strategy Settings
Precursor scoring | Relevance Heuristic |
---|---|
Min. plausibility | 0.01 |
Model | Template_relevance |
Template Set | Pistachio/Bkms_metabolic/Pistachio_ringbreaker/Reaxys/Reaxys_biocatalysis |
Top-N result to add to graph | 6 |
Feasible Synthetic Routes
Haftungsausschluss und Informationen zu In-Vitro-Forschungsprodukten
Bitte beachten Sie, dass alle Artikel und Produktinformationen, die auf BenchChem präsentiert werden, ausschließlich zu Informationszwecken bestimmt sind. Die auf BenchChem zum Kauf angebotenen Produkte sind speziell für In-vitro-Studien konzipiert, die außerhalb lebender Organismen durchgeführt werden. In-vitro-Studien, abgeleitet von dem lateinischen Begriff "in Glas", beinhalten Experimente, die in kontrollierten Laborumgebungen unter Verwendung von Zellen oder Geweben durchgeführt werden. Es ist wichtig zu beachten, dass diese Produkte nicht als Arzneimittel oder Medikamente eingestuft sind und keine Zulassung der FDA für die Vorbeugung, Behandlung oder Heilung von medizinischen Zuständen, Beschwerden oder Krankheiten erhalten haben. Wir müssen betonen, dass jede Form der körperlichen Einführung dieser Produkte in Menschen oder Tiere gesetzlich strikt untersagt ist. Es ist unerlässlich, sich an diese Richtlinien zu halten, um die Einhaltung rechtlicher und ethischer Standards in Forschung und Experiment zu gewährleisten.