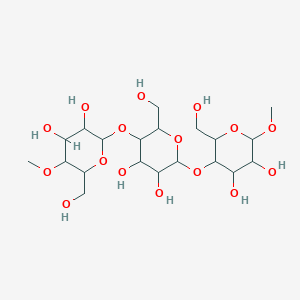
Galactan
- Klicken Sie auf QUICK INQUIRY, um ein Angebot von unserem Expertenteam zu erhalten.
- Mit qualitativ hochwertigen Produkten zu einem WETTBEWERBSFÄHIGEN Preis können Sie sich mehr auf Ihre Forschung konzentrieren.
Übersicht
Beschreibung
Galactan, also known as galactosan, is a polysaccharide composed of polymerized galactose units. It is found in various natural sources, including terrestrial plants and marine macroalgae. Galactans typically have a core structure of galactose units connected by α(1→3) or α(1→6) linkages, with other monosaccharides as side chains .
Wissenschaftliche Forschungsanwendungen
Galactans have a wide range of scientific research applications due to their unique properties. They are used in:
Wirkmechanismus
Target of Action
Galactan, a polysaccharide primarily composed of galactose units, is widely distributed in terrestrial plants and macroalgae . It interacts with various biological targets, including macrophages , Toll-like receptor 2 , and potentially other cell types involved in immune response . These targets play crucial roles in immune response, cell signaling, and maintaining homeostasis .
Mode of Action
This compound interacts with its targets to induce various biological effects. For instance, a specific type of this compound, 3-O-methylated-galactan, has been shown to polarize macrophages, leading to enhanced secretion of the cytokine TNF-α . This interaction results in cytotoxic effects on certain cancer cells . The mode of action involves targeting Toll-like receptor 2 and activation of MAPKs and NF-κB signaling pathways .
Biochemical Pathways
This compound’s interaction with its targets affects several biochemical pathways. In the case of macrophages, this compound influences the polarization process, which involves various signaling pathways, including MAPKs and NF-κB . These pathways play a crucial role in immune response and inflammation, leading to downstream effects such as enhanced cytokine secretion and cytotoxic effects on cancer cells .
Pharmacokinetics
It is known that non-absorbed this compound is vigorously fermented by gastrointestinal microflora . This fermentation process can lead to the production of short-chain fatty acids and decrease the generation and absorption of ammonia .
Result of Action
The interaction of this compound with its targets and the subsequent changes in biochemical pathways result in various molecular and cellular effects. For instance, the polarization of macrophages by this compound leads to enhanced secretion of cytokine TNF-α, which has cytotoxic effects on certain cancer cells . Additionally, this compound has been reported to have antitumor effects .
Action Environment
The action of this compound can be influenced by various environmental factors. For instance, the structural properties of this compound, such as its sulfation patterns, can greatly influence its functional properties . Moreover, the presence of 3,6-anhydrogalactopyranose residues in galactans can increase the flexibility of the this compound chains and allow larger contraction of the random coil structure . These factors can affect the rheological properties of this compound and its interaction with other compounds .
Biochemische Analyse
Biochemical Properties
Galactan participates in numerous biochemical reactions. It interacts with a variety of enzymes, proteins, and other biomolecules. For instance, the this compound Synthase 1 (GalS1) enzyme is responsible for the synthesis of β-1,4-galactan chains . The interaction between this compound and these biomolecules is often facilitated by specific binding sites, which have been identified through deep evolutionary analysis and molecular simulations .
Cellular Effects
This compound has significant effects on various types of cells and cellular processes. It influences cell function by impacting cell signaling pathways, gene expression, and cellular metabolism. For example, in plants, this compound is involved in seedling development .
Molecular Mechanism
The molecular mechanism of this compound involves its binding interactions with biomolecules, enzyme inhibition or activation, and changes in gene expression. For instance, this compound interacts with the GalS1 enzyme to facilitate the synthesis of β-1,4-galactan chains .
Temporal Effects in Laboratory Settings
In laboratory settings, the effects of this compound can change over time. This includes information on this compound’s stability, degradation, and any long-term effects on cellular function observed in in vitro or in vivo studies .
Metabolic Pathways
This compound is involved in several metabolic pathways. It interacts with various enzymes and cofactors, and can influence metabolic flux or metabolite levels. For example, this compound is synthesized by the GalS1 enzyme in a specific metabolic pathway .
Vorbereitungsmethoden
Synthetic Routes and Reaction Conditions
Galactans can be extracted from natural sources such as red seaweeds (e.g., Gracilaria species) using eco-friendly methods. The extraction process involves grinding the dried seaweed and using a mixed solvent of acetone, chloroform, and methanol in a Soxhlet apparatus for 48 hours . The extracted galactans are then characterized using techniques like FT-IR, 13C NMR, GC-MS, and rheological measurements .
Industrial Production Methods
In industrial settings, galactans are primarily obtained from agarophytes, which are red seaweeds used for agar production. The extraction process includes pretreatment, extraction, and purification steps. The specific methods applied for galactan isolation and purification vary depending on the source and desired properties .
Analyse Chemischer Reaktionen
Types of Reactions
Galactans undergo various chemical reactions, including oxidation, reduction, and substitution. For example, sulfated galactans can be prepared by partial hydrolysis and subsequent chemical sulfonation .
Common Reagents and Conditions
Common reagents used in galactan reactions include acids for hydrolysis and sulfating agents for sulfonation. The conditions for these reactions typically involve controlled temperatures and pH levels to ensure the desired modifications .
Major Products Formed
The major products formed from this compound reactions include sulfated galactans, which have enhanced biological activities such as anticoagulant and antithrombotic properties .
Vergleich Mit ähnlichen Verbindungen
Galactans are compared with other polysaccharides such as carrageenans and agars. While all these compounds are derived from marine macroalgae, galactans are unique due to their specific structural features and biological activities . Similar compounds include:
Carrageenans: Sulfated polysaccharides with gelling properties.
Agars: Polysaccharides used as gelling agents in microbiological media.
Eigenschaften
CAS-Nummer |
39300-87-3 |
---|---|
Molekularformel |
C14H26O11 |
Molekulargewicht |
370.35 g/mol |
IUPAC-Name |
(2S,3R,4R,5R,6R)-2-[(2R,3R,4R,5R,6R)-4,5-dihydroxy-2-(hydroxymethyl)-6-methoxyoxan-3-yl]oxy-6-(hydroxymethyl)-5-methoxyoxane-3,4-diol |
InChI |
InChI=1S/C14H26O11/c1-21-11-5(3-15)24-14(10(20)7(11)17)25-12-6(4-16)23-13(22-2)9(19)8(12)18/h5-20H,3-4H2,1-2H3/t5-,6-,7-,8-,9-,10-,11+,12+,13-,14+/m1/s1 |
InChI-Schlüssel |
PTHCMJGKKRQCBF-ICIGWGHZSA-N |
SMILES |
COC1C(OC(C(C1O)O)OC2C(OC(C(C2O)O)OC3C(OC(C(C3O)O)OC)CO)CO)CO |
Isomerische SMILES |
CO[C@H]1[C@H](O[C@H]([C@@H]([C@H]1O)O)O[C@H]2[C@H](O[C@H]([C@@H]([C@H]2O)O)OC)CO)CO |
Kanonische SMILES |
COC1C(OC(C(C1O)O)OC2C(OC(C(C2O)O)OC)CO)CO |
Physikalische Beschreibung |
Solid |
Synonyme |
GALACTAN EX GUM ARABIC; GALACTANE; GALACTAN; POLYGALACTAN; GALACTAN, AUS GUMMI ARABICUM; GALACTAL; GalactanPolygalactan |
Herkunft des Produkts |
United States |
Haftungsausschluss und Informationen zu In-Vitro-Forschungsprodukten
Bitte beachten Sie, dass alle Artikel und Produktinformationen, die auf BenchChem präsentiert werden, ausschließlich zu Informationszwecken bestimmt sind. Die auf BenchChem zum Kauf angebotenen Produkte sind speziell für In-vitro-Studien konzipiert, die außerhalb lebender Organismen durchgeführt werden. In-vitro-Studien, abgeleitet von dem lateinischen Begriff "in Glas", beinhalten Experimente, die in kontrollierten Laborumgebungen unter Verwendung von Zellen oder Geweben durchgeführt werden. Es ist wichtig zu beachten, dass diese Produkte nicht als Arzneimittel oder Medikamente eingestuft sind und keine Zulassung der FDA für die Vorbeugung, Behandlung oder Heilung von medizinischen Zuständen, Beschwerden oder Krankheiten erhalten haben. Wir müssen betonen, dass jede Form der körperlichen Einführung dieser Produkte in Menschen oder Tiere gesetzlich strikt untersagt ist. Es ist unerlässlich, sich an diese Richtlinien zu halten, um die Einhaltung rechtlicher und ethischer Standards in Forschung und Experiment zu gewährleisten.