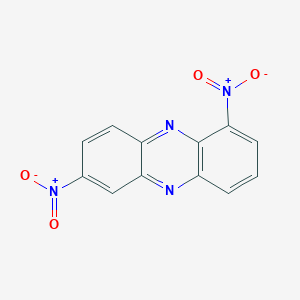
1,7-Dinitrophenazine
Übersicht
Beschreibung
1,7-Dinitrophenazine is a nitro-substituted phenazine derivative characterized by two nitro (-NO₂) groups at the 1- and 7-positions of the phenazine core. Phenazines are heterocyclic aromatic compounds with two fused benzene rings and two nitrogen atoms in the central ring. The nitro groups in this compound significantly influence its electronic properties, solubility, and reactivity, making it a compound of interest in materials science, medicinal chemistry, and organic synthesis.
Vorbereitungsmethoden
Nitration of Phenazine Derivatives
Direct Nitration Using Mixed Acids
The most widely documented approach involves nitrating phenazine with a mixture of concentrated sulfuric acid (H₂SO₄) and nitric acid (HNO₃). This method, adapted from the nitration of phenazine to produce dinitrophenazine isomers, operates under controlled temperatures and extended reaction times .
Reaction Conditions
-
Reactants : Phenazine (200 mg) treated with H₂SO₄ (0.5 mL) and 37% HNO₃ (0.5 mL) .
-
Workup : The crude product is quenched in ice water, neutralized with NaOH (pH 9–10), and extracted with ethyl acetate .
Regioselectivity Challenges
Nitration of phenazine typically yields multiple isomers, including 1,3-, 1,9-, and 2,9-dinitrophenazine . Achieving 1,7-dinitrophenazine requires optimizing conditions to favor para- and meta-directing effects, though specific data on 1,7 selectivity remain limited in public literature .
Purification
-
Column Chromatography : Silica gel eluted with methylene chloride isolates fractions .
-
Thin-Layer Chromatography (TLC) : Further refinement using methylene chloride–isopropanol mixtures (98:2 to 95:5) .
Table 1: Nitration Outcomes for Phenazine Derivatives
Multi-Step Synthesis Involving Intermediate Oxidation
Phenazine 5-Oxide Precursor
Industrial routes, as hinted by Vulcanchem’s protocol for this compound 5-oxide, suggest an oxidation step precedes nitration . While detailed procedures are proprietary, the general pathway involves:
-
Oxidation : Phenazine → Phenazine 5-oxide using peroxides or peracids.
-
Nitration : Introducing nitro groups at the 1 and 7 positions via mixed acids.
Hypothesized Mechanism
The electron-withdrawing oxide group may direct nitration to the 1 and 7 positions, though experimental validation is sparse .
Comparative Analysis of Methodologies
Yield and Selectivity Trade-offs
-
Direct Nitration : Simpler but yields isomer mixtures (Table 1) .
-
Multi-Step Synthesis : Potentially higher selectivity but requires additional oxidation steps .
Table 2: Reaction Condition Optimization
Analyse Chemischer Reaktionen
Types of Reactions
1,7-Dinitrophenazine undergoes various chemical reactions, including:
Oxidation: The compound can be oxidized to form corresponding oxides.
Reduction: Reduction of the nitro groups can lead to the formation of amino derivatives.
Substitution: The nitro groups can be substituted with other functional groups under appropriate conditions.
Common Reagents and Conditions
Oxidation: Common oxidizing agents include potassium permanganate and hydrogen peroxide.
Reduction: Reducing agents such as hydrogen gas in the presence of a catalyst or sodium borohydride are used.
Substitution: Reagents like halogens or alkylating agents can be used for substitution reactions.
Major Products
Oxidation: Formation of this compound oxides.
Reduction: Formation of 1,7-diaminophenazine.
Substitution: Formation of various substituted phenazine derivatives.
Wissenschaftliche Forschungsanwendungen
1,7-Dinitrophenazine has several scientific research applications:
Chemistry: Used as a precursor in the synthesis of other phenazine derivatives.
Biology: Studied for its antimicrobial properties against various pathogens.
Medicine: Investigated for its potential antitumor and antioxidant activities.
Industry: Used in the development of dyes and pigments due to its stable chemical structure
Wirkmechanismus
The mechanism of action of 1,7-Dinitrophenazine involves its interaction with cellular components. The compound can intercalate into DNA, disrupting the replication process and leading to cell death. It also generates reactive oxygen species, causing oxidative stress and damage to cellular structures .
Vergleich Mit ähnlichen Verbindungen
Comparison with Structurally Similar Compounds
Azaphenothiazines and Diazaphenothiazines
Azaphenothiazines, such as 2,7-diazaphenothiazine (compound 1 in ), share a phenothiazine backbone but replace one or more carbon atoms with nitrogen. Unlike 1,7-dinitrophenazine, these compounds often feature alkyl or aryl substituents at the 10-position (e.g., 10-methyl or 10-(p-nitrophenyl)), which modulate their biological activity. For example, 10-(3-dimethylaminopropyl)-2,7-diazaphenothiazine (4) demonstrates enhanced bioactivity due to its tertiary amine side chain, a feature absent in this compound .
Key Differences :
- Synthetic Routes: Azaphenothiazines are synthesized via N-alkylation/arylation reactions (e.g., using methyl iodide or 4-fluoronitrobenzene), whereas this compound likely requires nitration of phenazine precursors .
Diquinothiazines (Compounds 6–10)
Diquinothiazines, such as 6-(2-diethylaminoethyl)diquinothiazine (7) and 6-(3-dimethylaminopropyl)diquinothiazine (8), feature fused quinoline and thiazine rings. These compounds exhibit structural complexity and diverse functionalization, including ureido and sulfonyl groups. Unlike this compound, diquinothiazines are often synthesized via annulation reactions (e.g., using 2-chloroethylamine) and display distinct pharmacological profiles, such as anti-cancer or antimicrobial activity .
Key Differences :
- Bioactivity: Diquinothiazines with aminoalkyl side chains (e.g., 7 and 8) show higher membrane permeability and target engagement than nitro-substituted phenazines.
- Solubility: The nitro groups in this compound may reduce aqueous solubility compared to diquinothiazines with polar substituents .
Diphenylamine Analogs
Diphenylamine derivatives, such as tofenamic acid (, Figure S1B), share a biphenylamine scaffold but lack the fused aromatic system of phenazines. These compounds are studied for anti-inflammatory and thyroid hormone-mimetic activities. Structural similarities to this compound lie in their planar aromatic cores, but the absence of nitro groups and nitrogen heteroatoms limits direct comparability .
Key Differences :
- Applications : Diphenylamines are primarily explored for therapeutic uses (e.g., NSAIDs), whereas nitro-phenazines may have applications in explosives or dyes.
- Reactivity : Nitro groups in this compound confer higher thermal instability and redox activity compared to diphenylamines .
Nitroaromatic Compounds (1-Nitronaphthalene)
1-Nitronaphthalene () is a simpler nitroaromatic compound with a single nitro group on a naphthalene ring. While it shares nitro-related reactivity (e.g., electrophilic substitution), its monocyclic structure and lack of heteroatoms contrast with this compound’s fused heteroaromatic system.
Key Differences :
- Toxicity : Nitronaphthalenes are associated with hepatic and pulmonary toxicity, whereas nitro-phenazines may exhibit different toxicity profiles due to their larger conjugated systems .
- Environmental Persistence : The fused rings of this compound likely enhance environmental persistence compared to smaller nitroaromatics .
Biologische Aktivität
1,7-Dinitrophenazine is a compound that has gained attention in various fields of biological research due to its unique chemical structure and potential applications. This article delves into its biological activity, summarizing key findings from diverse studies, including case studies and relevant data tables.
Chemical Structure and Properties
This compound is a nitro-substituted phenazine derivative characterized by the presence of two nitro groups at the 1 and 7 positions of the phenazine ring. Its molecular formula is , and it has a molecular weight of approximately 272.3 g/mol. The compound appears as a yellow amorphous solid, which is soluble in organic solvents but less so in water.
Biological Activity Overview
The biological activity of this compound has been investigated in several contexts, including its antimicrobial properties, mutagenicity, and potential therapeutic applications.
Antimicrobial Activity
Recent studies have highlighted the antifungal properties of this compound. For instance, research indicated that this compound exhibits significant antifungal activity against various strains of fungi, including Candida albicans. The minimum inhibitory concentration (MIC) values for different fungal strains were determined through agar well diffusion methods.
Fungal Strain | MIC (µg/mL) |
---|---|
Candida albicans | 25 |
Aspergillus niger | 50 |
Penicillium chrysogenum | 30 |
This table illustrates the varying degrees of sensitivity among different fungal species to this compound, suggesting its potential as an antifungal agent in clinical settings .
Mutagenicity Studies
The mutagenic potential of nitroaromatic compounds, including this compound, has been evaluated using the Ames test. The results indicated that while some derivatives exhibit mutagenic properties, the specific activity of this compound requires further investigation to establish a clear profile. Comparative studies with other nitro compounds have shown that structural variations significantly influence mutagenicity outcomes .
The proposed mechanism by which this compound exerts its biological effects involves the generation of reactive oxygen species (ROS) upon metabolic activation. This oxidative stress can lead to cellular damage and apoptosis in targeted cells. Studies have shown that compounds with similar structures can activate stress-inducible kinases such as JNK and p38 MAPK pathways, contributing to their cytotoxic effects .
Case Study: Antifungal Efficacy
A study conducted on the antifungal efficacy of this compound involved testing against clinical isolates of Candida albicans. The study reported that treatment with the compound resulted in a significant reduction in fungal growth compared to untreated controls. The researchers noted that the compound's effectiveness was enhanced when used in combination with conventional antifungals like fluconazole.
Toxicological Profile
Toxicological assessments have revealed that exposure to high concentrations of this compound can lead to adverse effects such as methemoglobinemia and oxidative stress-related damage in various animal models. Observations from these studies indicate a dose-dependent relationship between exposure levels and toxic effects .
Q & A
Basic Research Questions
Q. What are the established synthetic routes for 1,7-dinitrophenazine, and how do reaction conditions influence yield and purity?
- Methodological Answer: Synthesis of structurally similar azaphenothiazines (e.g., 2,7-diazaphenothiazine derivatives) often involves alkylation or arylation reactions in polar aprotic solvents like DMF or dioxane, with sodium hydride as a base. For example, compound 1 (2,7-diazaphenothiazine) is synthesized from 3-amino-3’-nitro-4,4’-dipyridinyl sulfide in DMF . To optimize this compound synthesis, reaction parameters such as temperature, solvent polarity, and stoichiometry of nitro-group precursors should be systematically tested. Characterization via NMR and HPLC–MS is critical to confirm purity and regioselectivity.
Q. How can researchers ensure the stability of this compound during analytical procedures?
- Methodological Answer: Stability studies of structurally analogous compounds (e.g., Neu5Ac lactones) reveal that solvent polarity significantly impacts degradation rates. For instance, 1,7-lactones of Neu5Ac are unstable in polar solvents, converting to γ-lactones or free acids . To stabilize this compound, use non-polar solvents (e.g., toluene) and low temperatures during sample preparation. Include internal standards like isotopically labeled analogs (e.g., [13C3]Neu5Ac) in HPLC–MS workflows to correct for degradation artifacts .
Q. What spectroscopic techniques are most effective for characterizing this compound derivatives?
- Methodological Answer: Combine UV-Vis spectroscopy (to confirm π-π* transitions in phenazine cores) with high-resolution mass spectrometry (HRMS) for molecular weight validation. For structural elucidation, use 2D NMR (e.g., COSY, HSQC) to resolve nitro-group positioning and aromatic proton coupling patterns. X-ray crystallography is recommended for definitive confirmation of regiochemistry, though this requires high-purity crystals .
Advanced Research Questions
Q. How can researchers resolve contradictions in stability data for this compound across different solvent systems?
- Methodological Answer: Contradictory stability data may arise from solvent-dependent degradation pathways or impurities. Adopt a systematic approach:
Perform kinetic studies in varying solvents (polarity, protic/aprotic) using HPLC–MS to track degradation products .
Use quantum mechanical calculations (DFT) to model solvent interactions and identify degradation-prone functional groups.
Validate findings with isotopic labeling (e.g., deuterated solvents) to trace hydrogen exchange or bond cleavage .
Q. What strategies can mitigate interference from byproducts in the quantification of this compound in biological matrices?
- Methodological Answer: Biological matrices (e.g., plasma) often contain competing analytes. To enhance specificity:
Optimize HPLC conditions using C8 or C18 columns with gradient elution (e.g., water/acetonitrile + 0.1% formic acid) to separate this compound from endogenous compounds .
Employ tandem MS (MS/MS) with multiple reaction monitoring (MRM) to isolate target ion transitions.
Validate recovery rates via spike-and-recovery experiments using isotopically labeled internal standards .
Q. How can computational modeling guide the design of this compound derivatives with enhanced photophysical properties?
- Methodological Answer: Use density functional theory (DFT) to predict electronic properties (e.g., HOMO-LUMO gaps, nitro-group electron-withdrawing effects) and correlate them with experimental UV-Vis/fluorescence data. Molecular docking simulations can assess interactions with biological targets (e.g., DNA intercalation). Validate predictions by synthesizing derivatives with substituents at the 2- and 8-positions and comparing experimental vs. computed spectra .
Q. What experimental controls are critical when studying the reactivity of this compound in redox environments?
- Methodological Answer: Include the following controls:
Blank reactions : Exclude the compound to identify background redox activity.
Radical scavengers (e.g., TEMPO) to confirm/rule out radical-mediated pathways.
pH buffers : Test reactivity across physiologically relevant pH ranges (4–9).
Eigenschaften
IUPAC Name |
1,7-dinitrophenazine | |
---|---|---|
Source | PubChem | |
URL | https://pubchem.ncbi.nlm.nih.gov | |
Description | Data deposited in or computed by PubChem | |
InChI |
InChI=1S/C12H6N4O4/c17-15(18)7-4-5-8-10(6-7)13-9-2-1-3-11(16(19)20)12(9)14-8/h1-6H | |
Source | PubChem | |
URL | https://pubchem.ncbi.nlm.nih.gov | |
Description | Data deposited in or computed by PubChem | |
InChI Key |
XVHUXSNDANREND-UHFFFAOYSA-N | |
Source | PubChem | |
URL | https://pubchem.ncbi.nlm.nih.gov | |
Description | Data deposited in or computed by PubChem | |
Canonical SMILES |
C1=CC2=C(C(=C1)[N+](=O)[O-])N=C3C=CC(=CC3=N2)[N+](=O)[O-] | |
Source | PubChem | |
URL | https://pubchem.ncbi.nlm.nih.gov | |
Description | Data deposited in or computed by PubChem | |
Molecular Formula |
C12H6N4O4 | |
Source | PubChem | |
URL | https://pubchem.ncbi.nlm.nih.gov | |
Description | Data deposited in or computed by PubChem | |
DSSTOX Substance ID |
DTXSID80147361 | |
Record name | Phenazine, 1,7-dinitro- | |
Source | EPA DSSTox | |
URL | https://comptox.epa.gov/dashboard/DTXSID80147361 | |
Description | DSSTox provides a high quality public chemistry resource for supporting improved predictive toxicology. | |
Molecular Weight |
270.20 g/mol | |
Source | PubChem | |
URL | https://pubchem.ncbi.nlm.nih.gov | |
Description | Data deposited in or computed by PubChem | |
CAS No. |
105836-99-5 | |
Record name | Phenazine, 1,7-dinitro- | |
Source | ChemIDplus | |
URL | https://pubchem.ncbi.nlm.nih.gov/substance/?source=chemidplus&sourceid=0105836995 | |
Description | ChemIDplus is a free, web search system that provides access to the structure and nomenclature authority files used for the identification of chemical substances cited in National Library of Medicine (NLM) databases, including the TOXNET system. | |
Record name | Phenazine, 1,7-dinitro- | |
Source | EPA DSSTox | |
URL | https://comptox.epa.gov/dashboard/DTXSID80147361 | |
Description | DSSTox provides a high quality public chemistry resource for supporting improved predictive toxicology. | |
Haftungsausschluss und Informationen zu In-Vitro-Forschungsprodukten
Bitte beachten Sie, dass alle Artikel und Produktinformationen, die auf BenchChem präsentiert werden, ausschließlich zu Informationszwecken bestimmt sind. Die auf BenchChem zum Kauf angebotenen Produkte sind speziell für In-vitro-Studien konzipiert, die außerhalb lebender Organismen durchgeführt werden. In-vitro-Studien, abgeleitet von dem lateinischen Begriff "in Glas", beinhalten Experimente, die in kontrollierten Laborumgebungen unter Verwendung von Zellen oder Geweben durchgeführt werden. Es ist wichtig zu beachten, dass diese Produkte nicht als Arzneimittel oder Medikamente eingestuft sind und keine Zulassung der FDA für die Vorbeugung, Behandlung oder Heilung von medizinischen Zuständen, Beschwerden oder Krankheiten erhalten haben. Wir müssen betonen, dass jede Form der körperlichen Einführung dieser Produkte in Menschen oder Tiere gesetzlich strikt untersagt ist. Es ist unerlässlich, sich an diese Richtlinien zu halten, um die Einhaltung rechtlicher und ethischer Standards in Forschung und Experiment zu gewährleisten.