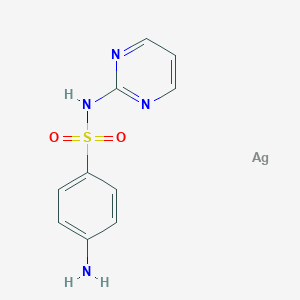
Silver sulfadiazine
Übersicht
Beschreibung
Silver sulfadiazine (SSD, C₁₀H₉AgN₄O₂S) is a topical antimicrobial agent synthesized through the reaction of sulfadiazine with silver nitrate, forming a water-insoluble complex . It is widely used for infected burn wounds due to its dual mechanism: sustained release of silver ions (Ag⁺) and the antibacterial action of sulfadiazine, a sulfonamide . SSD’s slow Ag⁺ release distinguishes it from rapid-release silver salts like silver nitrate (AgNO₃), reducing cytotoxicity while maintaining prolonged antimicrobial activity . Proprietary names include Silvadene®, Flamazine®, and Thermazene® .
Vorbereitungsmethoden
Chemical Synthesis via Catalyzed Precipitation
Reaction Mechanism and Catalytic Enhancement
The conventional chemical synthesis involves the reaction of silver nitrate (AgNO₃) with sulfadiazine sodium (C₁₀H₉N₄NaO₂S) in aqueous medium. A key advancement documented in patent CN101613323B introduces trifluoromethanesulfonic acid (TFMS) or its salts as catalysts to improve reaction efficiency . The catalytic mechanism involves nucleophilic substitution at the sulfonamide nitrogen, facilitating the formation of silver sulfadiazine while minimizing side reactions.
The reaction proceeds as follows:
3 + \text{C}{10}\text{H}9\text{N}4\text{NaO}2\text{S} \xrightarrow{\text{TFMS catalyst}} \text{C}{10}\text{H}9\text{AgN}4\text{O}2\text{S} + \text{NaNO}3
The catalyst concentration (0.1–1.0% mol relative to sulfadiazine) and temperature (45–55°C) critically influence yield and purity .
Temperature Control
Elevating the reaction temperature to 48–52°C enhances ionic mobility and reaction kinetics, achieving 98.5–98.8% purity and >97% yield (Table 1) . Lower temperatures (e.g., 25°C) result in incomplete reactions, reducing yield to 85–90% .
Catalyst Selection
Trifluoromethanesulfonic acid sodium (TFMS-Na) outperforms potassium or lithium salts due to its solubility and stability in alkaline conditions. At 0.5–0.6% mol catalyst loading, the reaction achieves near-stoichiometric conversion, eliminating the need for excess sulfadiazine sodium .
Table 1: Impact of Temperature and Catalyst Loading on Chemical Synthesis
Temperature (°C) | Catalyst Loading (% mol) | Purity (%) | Yield (%) |
---|---|---|---|
45 | 0.1 | 97.2 | 95.1 |
49 | 0.5 | 98.7 | 97.8 |
55 | 0.8 | 98.5 | 97.3 |
25 (Control) | 0 | 93.4 | 86.2 |
Industrial-Scale Process
The patented method involves sequential steps:
-
Silver Nitrate Solution Preparation : AgNO₃ dissolved in purified water at 80°C.
-
Sulfadiazine Sodium Synthesis : Sulfadiazine powder reacted with NaOH in the presence of TFMS-Na.
-
Precipitation and Purification : Combined solutions stirred at 45–55°C, followed by centrifugation and drying (80–90°C for 8 hours) .
Electrochemical Synthesis: A Green Alternative
Single-Step Galvanostatic Approach
A novel electrochemical method reported by RSC Advances eliminates the need for pre-synthesized silver salts . The setup comprises:
-
Anode : Sacrificial silver rod (Ag → Ag⁺ + e⁻).
-
Cathode : Stainless steel plate (2H⁺ + 2e⁻ → H₂).
The cell is divided into two chambers: -
Anolyte : Sulfadiazine and NaNO₃ in water.
-
Catholyte : HNO₃ solution.
The overall reaction is:
{10}\text{H}{10}\text{N}4\text{O}2\text{S} + \text{H}^+ \rightarrow \text{C}{10}\text{H}9\text{AgN}4\text{O}2\text{S} + \text{H}_2
Current Density
A current density of 10 mA cm⁻² balances reaction rate and energy consumption. Higher densities (>15 mA cm⁻²) cause excessive Ag⁺ release, leading to particle aggregation .
Electrolyte Composition
-
NaNO₃ Concentration : 0.1 M optimizes ionic conductivity without side reactions.
-
HNO₃ Concentration : 0.05 M maintains cathodic proton supply while minimizing corrosion.
Table 2: Electrochemical Synthesis Performance Under Optimized Conditions
Parameter | Optimal Value | Yield (%) | Energy Consumption (kWh/kg) |
---|---|---|---|
Current Density | 10 mA cm⁻² | 94.5 | 2.8 |
NaNO₃ Concentration | 0.1 M | 95.2 | 2.6 |
HNO₃ Concentration | 0.05 M | 94.8 | 2.7 |
Morphological Characteristics
Field-emission scanning electron microscopy (FE-SEM) reveals that electrochemically synthesized this compound forms microcrystals (1–5 µm) with uniform morphology, advantageous for topical formulations .
Comparative Analysis of Synthesis Methods
Table 3: Chemical vs. Electrochemical Synthesis
Analyse Chemischer Reaktionen
Reaktionstypen: Silbersulfadiazin durchläuft verschiedene chemische Reaktionen, darunter:
Oxidation: Silbersulfadiazin kann unter bestimmten Bedingungen oxidiert werden, was zur Bildung von Silberoxid und anderen Nebenprodukten führt.
Reduktion: Die Verbindung kann unter bestimmten reduzierenden Bedingungen zu ihren Bestandteilen, Silber und Sulfadiazin, reduziert werden.
Substitution: Silbersulfadiazin kann an Substitutionsreaktionen teilnehmen, bei denen das Silberion durch andere Metallionen ersetzt wird.
Häufige Reagenzien und Bedingungen:
Oxidation: Häufige Oxidationsmittel sind Wasserstoffperoxid und Kaliumpermanganat.
Reduktion: Reduktionsmittel wie Natriumborhydrid und Hydrazin werden häufig verwendet.
Substitution: Metallsalze wie Natriumchlorid und Kaliumbromid können in Substitutionsreaktionen verwendet werden.
Hauptprodukte, die gebildet werden:
Oxidation: Silberoxid und Sulfadiazin-Derivate.
Reduktion: Elementares Silber und Sulfadiazin.
Substitution: Verschiedene Metallsulfadiazin-Komplexe.
Wissenschaftliche Forschungsanwendungen
Wound Healing Applications
Effectiveness in Burn Treatment
Silver sulfadiazine is primarily used in the management of second- and third-degree burns. Its application helps to prevent infection, promote healing, and reduce pain. A systematic review indicated that SSD significantly enhances healing time compared to other treatments, showing a statistically significant difference in healing rates (p<0.0001) when compared to non-silver dressings .
Mechanism of Action
The mechanism by which this compound exerts its effects involves the disruption of bacterial cell membranes and walls, leading to cell death. Silver ions released from SSD bind to various cellular components, causing protein denaturation and enzyme inhibition . This action is complemented by sulfadiazine's ability to inhibit folic acid synthesis in bacteria, enhancing its antimicrobial efficacy.
Antimicrobial Properties
Broad-Spectrum Antibacterial Activity
this compound exhibits potent activity against a range of microorganisms, including both Gram-positive and Gram-negative bacteria. Studies have demonstrated its effectiveness in preventing infections in burn wounds, where the risk of bacterial colonization is high .
Comparative Studies
Research comparing SSD with other topical agents has shown that while SSD is effective, newer formulations incorporating silver nanoparticles may offer enhanced antimicrobial properties due to their increased surface area and reactivity .
Innovative Formulations
Hydrogel Incorporation
Recent studies have explored the incorporation of this compound into hydrogels for improved delivery and sustained release. For instance, SSD-loaded polyvinyl alcohol (PVA) hydrogels have been developed, demonstrating low cytotoxicity and effective antimicrobial activity over extended periods . These formulations can be particularly beneficial for chronic wounds requiring prolonged treatment.
Nanoparticle Applications
The development of silver nanoparticles (AgNPs) using green synthesis methods has opened new avenues for SSD applications. AgNPs possess unique properties that enhance their antimicrobial effects and reduce toxicity compared to traditional silver compounds. These nanoparticles can be utilized in various medical applications beyond wound care, including drug delivery systems and cancer therapy .
Case Studies and Clinical Trials
Clinical Efficacy in Burns
A randomized controlled trial compared the effectiveness of SSD with aloe vera cream in treating second-degree burns. Results indicated that SSD significantly outperformed aloe vera in terms of healing time and infection prevention .
Veterinary Applications
In veterinary medicine, this compound has been applied to treat skin injuries in animals. A study involving horses with induced skin injuries found that while SSD improved neovascularization and epithelialization rates compared to control treatments, results varied based on the specific conditions of the wounds .
Wirkmechanismus
Silver sulfadiazine exerts its effects by acting on the bacterial cell membrane and cell wall, leading to cell death . The silver ions bind to nucleophilic amino acids, sulfhydryl, amino, imidazole, phosphate, and carboxyl groups in proteins, causing protein denaturation and enzyme inhibition . This disrupts the bacterial cell membrane, leading to proton leaks and ultimately cell death .
Vergleich Mit ähnlichen Verbindungen
Comparison with Other Silver-Based Compounds
Silver Nitrate (AgNO₃)
- Mechanism: Rapid Ag⁺ release provides immediate bactericidal effects but increases cytotoxicity and genotoxicity via oxidative stress .
- Efficacy: While effective against Gram-positive and Gram-negative bacteria, AgNO₃ requires frequent reapplication due to rapid ion depletion .
- Safety : Higher risk of local toxicity compared to SSD .
Silver Sulfathiazole (Argosulfan®)
- Mechanism: Combines silver with sulfathiazole, another sulfonamide.
- Efficacy: Similar antimicrobial spectrum to SSD but less studied in burns. Limited evidence supports its superiority .
Colloidal Silver (Protargol®, Collargol®)
- Mechanism: Silver nanoparticles or protein-bound silver with variable Ag⁺ release.
- Efficacy : Less potent than SSD in burn infections; primarily used for ocular and nasal antiseptics .
Comparison with Non-Silver Topical Agents
Povidone-Iodine
- Mechanism : Broad-spectrum iodine-based antiseptic.
- Efficacy : In pressure ulcers, SSD showed superior bacterial reduction (70% vs. 50%) and faster healing compared to povidone-iodine .
- Safety : Iodine can cause thyroid dysfunction, whereas SSD risks systemic silver absorption .
Mafenide Acetate
- Mechanism : Sulfonamide derivative with eschar-penetrating ability.
- Efficacy : Effective in deep burns but inferior to SSD in infection prevention (34% vs. 28% infection rates) .
- Safety : Causes metabolic acidosis, unlike SSD .
Combination Therapies
SSD + Chlorhexidine (Silvazine®)
- Efficacy : Silvazine reduced infection rates by 15% compared to SSD alone in burn prophylaxis .
- Safety: No significant difference in adverse effects .
SSD + Hyaluronic Acid
- Efficacy : Accelerated healing by 3–5 days in partial-thickness burns and reduced edema .
- Mechanism : Hyaluronic acid enhances re-epithelialization, counteracting SSD’s delayed healing .
Efficacy and Clinical Outcomes
A 2019 meta-analysis compared SSD with 12 alternatives (e.g., honey, nanocrystalline silver) in 42 studies :
Outcome | SSD vs. Alternatives | Odds Ratio (95% CI) |
---|---|---|
Infection Prevention | Non-significant difference | 0.92 (0.78–1.09) |
Healing Time | Faster with SSD combinations | 1.34 (1.12–1.61) |
SSD remains the "gold standard" for burns, though combination therapies show promise .
Market and Usage Trends
Biologische Aktivität
Silver sulfadiazine (SSD) is a topical antimicrobial agent widely used in the treatment of burn wounds and other skin infections. Its effectiveness stems from its dual action as both a sulfonamide antibiotic and a silver-based compound, which provides broad-spectrum antibacterial activity. This article delves into the biological activity of this compound, exploring its mechanisms of action, efficacy against various pathogens, and clinical applications supported by research findings and case studies.
This compound exerts its antimicrobial effects through several mechanisms:
- Bacterial Cell Wall Interaction : SSD disrupts the integrity of bacterial cell membranes, leading to increased permeability and eventual cell lysis. This action is primarily attributed to silver ions, which bind to nucleophilic sites on proteins and nucleic acids, causing denaturation and enzyme inhibition .
- Inhibition of Folate Synthesis : The sulfadiazine component acts as a competitive inhibitor of para-aminobenzoic acid (PABA), essential for bacterial folate synthesis. This inhibition is crucial for the growth and replication of bacteria .
- Reactive Oxygen Species (ROS) Generation : Silver ions can induce oxidative stress in bacterial cells by generating reactive oxygen species, contributing to their bactericidal effects .
Mechanism | Description |
---|---|
Cell Membrane Disruption | Silver ions increase permeability, leading to cell lysis |
Folate Synthesis Inhibition | Sulfadiazine inhibits PABA, disrupting folate synthesis |
ROS Generation | Induction of oxidative stress through silver ion activity |
Antimicrobial Spectrum
This compound demonstrates broad-spectrum antimicrobial activity against various gram-positive and gram-negative bacteria, as well as some fungi. Key pathogens affected include:
- Gram-Positive Bacteria : Staphylococcus aureus, Streptococcus pyogenes
- Gram-Negative Bacteria : Escherichia coli, Pseudomonas aeruginosa
- Fungi : Certain strains of Candida spp.
Table 2: Efficacy Against Common Pathogens
Pathogen | Type | Minimum Inhibitory Concentration (MIC) |
---|---|---|
Staphylococcus aureus | Gram-Positive | 0.5 - 1 µg/mL |
Streptococcus pyogenes | Gram-Positive | 1 µg/mL |
Escherichia coli | Gram-Negative | 2 µg/mL |
Pseudomonas aeruginosa | Gram-Negative | 4 µg/mL |
Clinical Applications
This compound is primarily indicated for the prevention and treatment of burn wound infections. Its application has been extensively studied in various clinical settings:
Case Study 1: Burn Wound Management
A study involving 100 patients with second-degree burns demonstrated that those treated with SSD showed a significant reduction in infection rates compared to those receiving standard care without SSD. The healing time was also notably shorter in the SSD group .
Case Study 2: Efficacy Against Streptococcus pyogenes
In a comparative study, SSD was found to have an inhibitory effect on S. pyogenes comparable to that of mupirocin and fusidic acid creams. This suggests that SSD can be an effective alternative for managing infections caused by this pathogen in burn wounds .
Table 3: Clinical Outcomes in Burn Treatment
Treatment Group | Infection Rate (%) | Average Healing Time (Days) |
---|---|---|
This compound | 10 | 14 |
Standard Care | 30 | 21 |
Adverse Effects
While this compound is generally well-tolerated, some adverse effects have been reported:
- Local Reactions : Burning sensation, itching, or rash at the application site.
- Systemic Effects : Rarely, prolonged use can lead to argyria (skin discoloration due to silver accumulation) or methemoglobinemia.
Monitoring for these effects is essential, especially in patients with extensive burns receiving large areas of topical treatment .
Q & A
Basic Research Questions
Q. What are the primary mechanisms of silver sulfadiazine’s antimicrobial action in burn wound models, and how do researchers standardize experimental conditions to isolate these mechanisms?
- Methodological Answer: Researchers typically employ in vitro assays (e.g., broth microdilution or agar diffusion) to quantify minimum inhibitory concentrations (MICs) against common pathogens like Pseudomonas aeruginosa and Staphylococcus aureus. For in vivo models, partial-thickness burn wounds in rodents are standardized using controlled thermal injury devices. Variables such as wound size, bacterial inoculum concentration, and application frequency of this compound (SSD) are tightly regulated to isolate its ionic silver release and sulfadiazine-mediated folate pathway disruption .
Q. How do researchers address confounding variables when evaluating this compound’s efficacy in clinical versus preclinical studies?
- Methodological Answer: Preclinical studies often control variables like host immunity (e.g., using immunosuppressed rodents) and wound hydration. In clinical trials, stratification by burn severity (e.g., % total body surface area) and randomization are critical. Blinded assessments of wound healing rates (e.g., via planimetry) and standardized infection criteria (e.g., CDC definitions) reduce bias. Meta-analyses further adjust for heterogeneity across studies using statistical models (e.g., random-effects models) .
Q. What key parameters are measured to assess this compound’s impact on re-epithelialization in burn models?
- Methodological Answer: Histological metrics include epidermal thickness, collagen deposition (via Masson’s trichrome staining), and keratinocyte migration rates. Non-invasive tools like optical coherence tomography (OCT) and transepidermal water loss (TEWL) measurements are used to monitor barrier function restoration. Researchers also track pro-inflammatory cytokines (e.g., IL-6, TNF-α) to differentiate SSD’s antimicrobial effects from potential delays in healing .
Advanced Research Questions
Q. How can researchers resolve contradictions in the literature regarding this compound’s efficacy compared to newer antimicrobial agents (e.g., nanocrystalline silver)?
- Methodological Answer: Comparative studies must standardize endpoints (e.g., time to 50% wound closure, bacterial load reduction) and control for formulation differences (e.g., SSD’s cream base vs. nanocrystalline silver’s moisture-retaining dressings). Bayesian network meta-analyses can rank treatments by efficacy while accounting for indirect comparisons. In vitro models simulating wound biofilm complexity (e.g., flow-cell systems) may clarify mechanistic advantages .
Q. What experimental designs are optimal for evaluating the concentration-dependent cytotoxicity of this compound on human keratinocytes?
- Methodological Answer: Dose-response assays using immortalized keratinocyte lines (e.g., HaCaT) are combined with live/dead staining (e.g., calcein-AM/propidium iodide) and mitochondrial viability assays (e.g., MTT). To mimic in vivo conditions, 3D keratinocyte-fibroblast co-cultures or ex vivo human skin explants are exposed to SSD at clinically relevant concentrations (typically 1% w/w). Confocal microscopy tracks silver nanoparticle penetration and cellular apoptosis markers (e.g., caspase-3) .
Q. How should researchers address discrepancies between in vitro antimicrobial activity and in vivo therapeutic outcomes for this compound?
- Methodological Answer: Bridging this gap requires pharmacokinetic/pharmacodynamic (PK/PD) modeling. In vitro assays should incorporate simulated wound fluid (e.g., albumin-rich media) to mimic silver ion binding. In vivo studies must measure silver deposition in eschar via inductively coupled plasma mass spectrometry (ICP-MS) and correlate it with bacterial clearance rates. Machine learning approaches can integrate multi-omics data (e.g., microbiome shifts, host transcriptomics) to identify predictive biomarkers .
Q. What methodologies are recommended for systematic reviews assessing this compound’s long-term resistance development in chronic wound populations?
- Methodological Answer: Follow PRISMA guidelines with explicit inclusion criteria (e.g., studies reporting ≥6-month follow-up). Extract data on resistance mechanisms (e.g., sil operon upregulation, efflux pump activity) and use GRADE criteria to assess evidence quality. Subgroup analyses by wound type (e.g., diabetic ulcers vs. burns) and geographic antimicrobial resistance patterns are critical. Prospectively registered protocols (e.g., PROSPERO) enhance reproducibility .
Q. How can researchers optimize this compound formulations to enhance penetration into biofilms without increasing cytotoxicity?
- Methodological Answer: Combinatorial approaches include encapsulating SSD in lipid nanoparticles or conjugating it with biofilm-disrupting agents (e.g., DNase I). Microfluidic systems model biofilm architecture to test penetration efficiency. Synchrotron radiation X-ray fluorescence (SR-XRF) maps silver distribution within biofilms. Toxicity is assessed via 3D human skin equivalents and genomic toxicity screens (e.g., Comet assay) .
Q. Data Analysis and Reporting Standards
Q. What statistical approaches are robust for handling heterogeneous outcomes in this compound meta-analyses?
- Methodological Answer: Multivariate meta-regression adjusts for covariates like burn depth and comorbidities. Sensitivity analyses exclude high-risk-of-bias studies (e.g., those lacking blinding). For rare adverse events (e.g., leukopenia), Bayesian hierarchical models pool sparse data. Forest plots should visualize heterogeneity (I² statistics), and trial sequential analysis (TSA) evaluates whether cumulative evidence is conclusive .
Q. How should researchers document raw data and experimental protocols to ensure reproducibility in this compound studies?
- Methodological Answer: Adhere to FAIR principles (Findable, Accessible, Interoperable, Reusable). Publish raw microscopy images, flow cytometry files, and bacterial viability counts in repositories like Figshare or Zenodo. Detailed protocols for SSD preparation (e.g., USP standards) and animal models should follow ARRIVE 2.0 guidelines. Use electronic lab notebooks with version control for transparency .
Eigenschaften
Key on ui mechanism of action |
Studies utilizing radioactive micronized silver sulfadiazine, electron microscopy, and biochemical techniques have revealed that the mechanism of action of silver sulfadiazine on bacteria differs from silver nitrate and sodium sulfadiazine. Silver sulfadiazine acts only on the cell membrane and cell wall to produce its bactericidal effect. A specific mechanism of action has not been determined, but silver sulfadiazine's effectiveness may possibly be from a synergistic interaction, or the action of each component. Silver is a biocide, which binds to a broad range of targets. Silver ions bind to nucleophilic amino acids, as well as sulfhydryl, amino, imidazole, phosphate, and carboxyl groups in proteins, causing protein denaturation and enzyme inhibition. Silver binds to surface membranes and proteins, causing proton leaks in the membrane, leading to cell death. Sulfadiazine is a competitive inhibitor of bacterial para-aminobenzoic acid (PABA), a substrate of the enzyme dihydropteroate synthetase. The inhibited reaction is necessary in these organisms for the synthesis of folic acid. |
---|---|
CAS-Nummer |
22199-08-2 |
Molekularformel |
C10H9AgN4O2S |
Molekulargewicht |
357.14 g/mol |
IUPAC-Name |
silver;(4-aminophenyl)sulfonyl-pyrimidin-2-ylazanide |
InChI |
InChI=1S/C10H9N4O2S.Ag/c11-8-2-4-9(5-3-8)17(15,16)14-10-12-6-1-7-13-10;/h1-7H,11H2;/q-1;+1 |
InChI-Schlüssel |
UEJSSZHHYBHCEL-UHFFFAOYSA-N |
SMILES |
C1=CN=C(N=C1)[N-]S(=O)(=O)C2=CC=C(C=C2)N.[Ag+] |
Kanonische SMILES |
C1=CN=C(N=C1)[N-]S(=O)(=O)C2=CC=C(C=C2)N.[Ag+] |
melting_point |
285 °C |
Key on ui other cas no. |
22199-08-2 |
Piktogramme |
Irritant |
Synonyme |
Brandiazin Dermazin Flamazine Flammazine Sicazine Silvadene Silvederma Silver Sulfadiazine Silver Sulfafdiazine SSD SSD AF Sulfadiazine, Silver Sulfafdiazine, Silver Sulfargen Thermazene |
Herkunft des Produkts |
United States |
Retrosynthesis Analysis
AI-Powered Synthesis Planning: Our tool employs the Template_relevance Pistachio, Template_relevance Bkms_metabolic, Template_relevance Pistachio_ringbreaker, Template_relevance Reaxys, Template_relevance Reaxys_biocatalysis model, leveraging a vast database of chemical reactions to predict feasible synthetic routes.
One-Step Synthesis Focus: Specifically designed for one-step synthesis, it provides concise and direct routes for your target compounds, streamlining the synthesis process.
Accurate Predictions: Utilizing the extensive PISTACHIO, BKMS_METABOLIC, PISTACHIO_RINGBREAKER, REAXYS, REAXYS_BIOCATALYSIS database, our tool offers high-accuracy predictions, reflecting the latest in chemical research and data.
Strategy Settings
Precursor scoring | Relevance Heuristic |
---|---|
Min. plausibility | 0.01 |
Model | Template_relevance |
Template Set | Pistachio/Bkms_metabolic/Pistachio_ringbreaker/Reaxys/Reaxys_biocatalysis |
Top-N result to add to graph | 6 |
Feasible Synthetic Routes
Haftungsausschluss und Informationen zu In-Vitro-Forschungsprodukten
Bitte beachten Sie, dass alle Artikel und Produktinformationen, die auf BenchChem präsentiert werden, ausschließlich zu Informationszwecken bestimmt sind. Die auf BenchChem zum Kauf angebotenen Produkte sind speziell für In-vitro-Studien konzipiert, die außerhalb lebender Organismen durchgeführt werden. In-vitro-Studien, abgeleitet von dem lateinischen Begriff "in Glas", beinhalten Experimente, die in kontrollierten Laborumgebungen unter Verwendung von Zellen oder Geweben durchgeführt werden. Es ist wichtig zu beachten, dass diese Produkte nicht als Arzneimittel oder Medikamente eingestuft sind und keine Zulassung der FDA für die Vorbeugung, Behandlung oder Heilung von medizinischen Zuständen, Beschwerden oder Krankheiten erhalten haben. Wir müssen betonen, dass jede Form der körperlichen Einführung dieser Produkte in Menschen oder Tiere gesetzlich strikt untersagt ist. Es ist unerlässlich, sich an diese Richtlinien zu halten, um die Einhaltung rechtlicher und ethischer Standards in Forschung und Experiment zu gewährleisten.