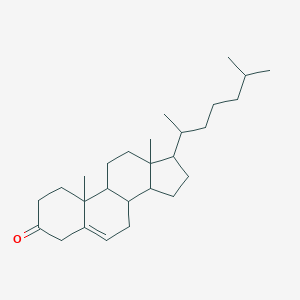
Cholest-5-EN-3-on
Übersicht
Beschreibung
CHOLEST-5-EN-3-ON, auch bekannt als 5-Cholesten-3-on, ist eine steroidale Verbindung, die aus Cholesterin gewonnen wird. Es zeichnet sich durch das Vorhandensein einer Ketogruppe am dritten Kohlenstoffatom und einer Doppelbindung zwischen dem fünften und sechsten Kohlenstoffatom in der Steroidringstruktur aus.
Wissenschaftliche Forschungsanwendungen
CHOLEST-5-EN-3-ON wurde ausgiebig auf seine Anwendungen in verschiedenen Bereichen untersucht:
Chemie: Wird als Zwischenprodukt bei der Synthese von steroidalen Medikamenten verwendet.
Biologie: Untersucht auf seine Rolle in Stoffwechselwegen und als Metabolit im Cholesterinstoffwechsel.
Medizin: Untersucht auf seine potenziellen Anti-Fettleibigkeit und entzündungshemmenden Wirkungen.
Wirkmechanismus
This compound übt seine Wirkungen durch verschiedene molekulare Mechanismen aus. Es ist an der Oxidation und Isomerisierung von Cholesterin beteiligt, die von Enzymen wie Cholesterinoxidase katalysiert wird. Die Verbindung kann die Aktivierung des nukleären Faktor Kappa B (NFκB) hemmen, wodurch die Produktion von entzündungsfördernden Zytokinen reduziert wird . Dieser Mechanismus ist insbesondere für seine entzündungshemmenden und anti-adipösen Wirkungen relevant.
Wirkmechanismus
Target of Action
Cholest-5-EN-3-one, also known as cholestenone, primarily targets the enzyme cholesterol oxidase . This enzyme is a bacterial-specific flavoenzyme that catalyzes the oxidation and isomerization of steroids containing a 3β hydroxyl group and a double bond at the Δ5–6 of the steroid ring system .
Mode of Action
Cholest-5-EN-3-one interacts with cholesterol oxidase, which catalyzes the oxidation and isomerization of cholesterol to cholest-4-en-3-one via cholest-5-en-3-one . It has been proposed that His447 acts as the general base catalyst for oxidation, and that the resulting imidazolium ion formed acts as an electrophile for isomerization .
Biochemical Pathways
The enzyme cholesterol oxidase catalyzes three chemical conversions . In the first step, the 3β-hydroxyl group of the steroid ring system is oxidized to the corresponding ketone. Key to this conversion is the FAD cofactor, which becomes reduced in the process. In the second step, the enzyme catalyzes isomerization of the double bond in the oxidized steroid ring system from the Δ5–6 position to Δ4–5 position, to give the final steroid product, cholest-4-en-3-one . In the final step of the enzyme, called the oxidative half-reaction, the reduced cofactor reacts with dioxygen and is thus reoxidized while O2 is reduced to H2O2 .
Pharmacokinetics
It’s known that every cell in vertebrates is able both to synthesize cholesterol and to metabolize it .
Result of Action
The result of the action of Cholest-5-EN-3-one is the production of cholest-4-en-3-one . This compound is an important synthetic intermediate in many steroid transformations . Previous studies have shown that it is effective against obesity, liver disease, and keratinization .
Biochemische Analyse
Biochemical Properties
Cholest-5-EN-3-one is involved in several biochemical reactions, primarily as an intermediate in the metabolism of cholesterol. One of the key enzymes that interact with cholest-5-EN-3-one is cholesterol oxidase, which catalyzes the oxidation of cholesterol to cholest-5-EN-3-one and subsequently to cholest-4-EN-3-one . This enzyme is crucial for the catabolism of cholesterol in microorganisms and has applications in clinical diagnostics and biotechnological processes.
In addition to cholesterol oxidase, cholest-5-EN-3-one interacts with various other enzymes and proteins involved in steroidogenesis. For instance, it serves as a substrate for 3β-hydroxysteroid dehydrogenase, which converts cholest-5-EN-3-one to pregnenolone, a precursor for steroid hormones . These interactions highlight the importance of cholest-5-EN-3-one in the synthesis of biologically active steroids.
Cellular Effects
Cholest-5-EN-3-one exerts significant effects on various cell types and cellular processes. In hepatocytes, it influences cholesterol homeostasis by modulating the expression of genes involved in cholesterol synthesis and uptake. Cholest-5-EN-3-one has been shown to regulate the activity of sterol regulatory element-binding proteins (SREBPs), which are key transcription factors controlling lipid metabolism . This regulation impacts cellular cholesterol levels and overall lipid homeostasis.
Furthermore, cholest-5-EN-3-one affects cell signaling pathways, particularly those related to inflammation and oxidative stress. It has been observed to modulate the activity of nuclear factor-kappa B (NF-κB), a transcription factor involved in the inflammatory response . By influencing NF-κB signaling, cholest-5-EN-3-one can impact the expression of pro-inflammatory cytokines and other mediators of inflammation.
Molecular Mechanism
The molecular mechanism of cholest-5-EN-3-one involves its interactions with specific biomolecules and enzymes. Cholest-5-EN-3-one binds to cholesterol oxidase, facilitating the oxidation of cholesterol to cholest-5-EN-3-one . This binding interaction is essential for the enzymatic conversion and subsequent metabolic processes.
Additionally, cholest-5-EN-3-one acts as a substrate for 3β-hydroxysteroid dehydrogenase, which catalyzes its conversion to pregnenolone . This enzymatic reaction is a critical step in the biosynthesis of steroid hormones, highlighting the role of cholest-5-EN-3-one in endocrine function.
Temporal Effects in Laboratory Settings
In laboratory settings, the effects of cholest-5-EN-3-one can vary over time. The stability and degradation of cholest-5-EN-3-one are influenced by factors such as temperature, pH, and the presence of oxidative agents. Studies have shown that cholest-5-EN-3-one is relatively stable under physiological conditions but can undergo degradation in the presence of strong oxidizing agents .
Long-term effects of cholest-5-EN-3-one on cellular function have been observed in both in vitro and in vivo studies. Prolonged exposure to cholest-5-EN-3-one can lead to alterations in gene expression and cellular metabolism, impacting processes such as lipid synthesis and inflammatory responses .
Dosage Effects in Animal Models
The effects of cholest-5-EN-3-one in animal models are dose-dependent. At low doses, cholest-5-EN-3-one has been shown to modulate cholesterol metabolism and improve lipid profiles . At high doses, it can induce toxic effects, including hepatotoxicity and oxidative stress . These adverse effects highlight the importance of careful dosage regulation in experimental and therapeutic applications.
Metabolic Pathways
Cholest-5-EN-3-one is involved in several metabolic pathways, primarily related to cholesterol metabolism. It is an intermediate in the biosynthesis of steroid hormones and bile acids. The conversion of cholest-5-EN-3-one to pregnenolone by 3β-hydroxysteroid dehydrogenase is a key step in steroidogenesis . Additionally, cholest-5-EN-3-one can be further metabolized to cholest-4-EN-3-one by cholesterol oxidase .
Transport and Distribution
Within cells and tissues, cholest-5-EN-3-one is transported and distributed through interactions with specific transporters and binding proteins. Lipoproteins, such as low-density lipoprotein (LDL) and high-density lipoprotein (HDL), play a crucial role in the transport of cholest-5-EN-3-one in the bloodstream . These lipoproteins facilitate the delivery of cholest-5-EN-3-one to target tissues, where it can exert its biochemical effects.
Subcellular Localization
Cholest-5-EN-3-one exhibits specific subcellular localization, which influences its activity and function. It is primarily localized in the endoplasmic reticulum and mitochondria, where it participates in the biosynthesis of steroid hormones and bile acids . The targeting of cholest-5-EN-3-one to these organelles is mediated by specific targeting signals and post-translational modifications.
Vorbereitungsmethoden
Synthesewege und Reaktionsbedingungen
CHOLEST-5-EN-3-ON kann durch verschiedene Verfahren synthetisiert werden. Ein gängiger Ansatz beinhaltet die Oxidation von Cholesterin. Der Prozess umfasst typischerweise die Verwendung von Oxidationsmitteln wie Chromtrioxid oder Pyridiniumchlorochromat in Gegenwart von Lösungsmitteln wie Dichlormethan . Eine andere Methode beinhaltet die Debromierung von Dibromcholestanons mit Zinkstaub in siedendem Ethanol oder Methanol .
Industrielle Produktionsverfahren
Die industrielle Produktion von this compound setzt häufig enzymatische Reaktionen ein. So kann beispielsweise Cholesterinoxidase aus Rhodococcus-Spezies verwendet werden, um Cholesterin in einem wässrig-organischen Zweiphasensystem in this compound umzuwandeln. Diese Methode ist aufgrund ihrer hohen Ausbeute und Reinheit vorteilhaft, wodurch sie sich für die großtechnische Produktion eignet .
Analyse Chemischer Reaktionen
Arten von Reaktionen
CHOLEST-5-EN-3-ON unterliegt verschiedenen chemischen Reaktionen, darunter:
Oxidation: Es kann weiter oxidiert werden, um Cholest-4-en-3-on zu bilden.
Reduktion: Die Reduktion von this compound kann Cholest-5-en-3-ol liefern.
Substitution: Es kann an Substitutionsreaktionen teilnehmen, wie z. B. Halogenierung, bei der Chlor- oder Bromatome Wasserstoffatome ersetzen
Häufige Reagenzien und Bedingungen
Oxidation: Chromtrioxid, Pyridiniumchlorochromat.
Reduktion: Natriumborhydrid, Lithiumaluminiumhydrid.
Substitution: Chlor, Brom in Lösungsmitteln wie Chloroform oder Chlorbenzol.
Hauptprodukte, die gebildet werden
Oxidation: Cholest-4-en-3-on.
Reduktion: Cholest-5-en-3-ol.
Substitution: 5α,5β-Dichlorid, 5β,6α-Dichlorid.
Vergleich Mit ähnlichen Verbindungen
Ähnliche Verbindungen
Cholest-4-en-3-on: Eine weitere oxidierte Form von Cholesterin mit einer Doppelbindung zwischen dem vierten und fünften Kohlenstoffatom.
Cholest-5-en-3-ol: Eine reduzierte Form von CHOLEST-5-EN-3-ON mit einer Hydroxylgruppe am dritten Kohlenstoffatom.
Cholest-7-en-3-on: Eine ähnliche Verbindung mit einer Doppelbindung zwischen dem siebten und achten Kohlenstoffatom
Einzigartigkeit
This compound ist aufgrund seiner spezifischen Struktur einzigartig, die es ihm ermöglicht, an unterschiedlichen biochemischen Reaktionen und Wegen teilzunehmen. Seine Fähigkeit, die NFκB-Aktivierung zu hemmen und Entzündungen zu reduzieren, unterscheidet es von anderen Cholesterinderivaten .
Eigenschaften
IUPAC Name |
10,13-dimethyl-17-(6-methylheptan-2-yl)-1,2,4,7,8,9,11,12,14,15,16,17-dodecahydrocyclopenta[a]phenanthren-3-one | |
---|---|---|
Details | Computed by Lexichem TK 2.7.0 (PubChem release 2021.05.07) | |
Source | PubChem | |
URL | https://pubchem.ncbi.nlm.nih.gov | |
Description | Data deposited in or computed by PubChem | |
InChI |
InChI=1S/C27H44O/c1-18(2)7-6-8-19(3)23-11-12-24-22-10-9-20-17-21(28)13-15-26(20,4)25(22)14-16-27(23,24)5/h9,18-19,22-25H,6-8,10-17H2,1-5H3 | |
Details | Computed by InChI 1.0.6 (PubChem release 2021.05.07) | |
Source | PubChem | |
URL | https://pubchem.ncbi.nlm.nih.gov | |
Description | Data deposited in or computed by PubChem | |
InChI Key |
GGCLNOIGPMGLDB-UHFFFAOYSA-N | |
Details | Computed by InChI 1.0.6 (PubChem release 2021.05.07) | |
Source | PubChem | |
URL | https://pubchem.ncbi.nlm.nih.gov | |
Description | Data deposited in or computed by PubChem | |
Canonical SMILES |
CC(C)CCCC(C)C1CCC2C1(CCC3C2CC=C4C3(CCC(=O)C4)C)C | |
Details | Computed by OEChem 2.3.0 (PubChem release 2021.05.07) | |
Source | PubChem | |
URL | https://pubchem.ncbi.nlm.nih.gov | |
Description | Data deposited in or computed by PubChem | |
Molecular Formula |
C27H44O | |
Details | Computed by PubChem 2.1 (PubChem release 2021.05.07) | |
Source | PubChem | |
URL | https://pubchem.ncbi.nlm.nih.gov | |
Description | Data deposited in or computed by PubChem | |
Molecular Weight |
384.6 g/mol | |
Details | Computed by PubChem 2.1 (PubChem release 2021.05.07) | |
Source | PubChem | |
URL | https://pubchem.ncbi.nlm.nih.gov | |
Description | Data deposited in or computed by PubChem | |
CAS No. |
601-54-7 | |
Record name | Cholesterone | |
Source | DTP/NCI | |
URL | https://dtp.cancer.gov/dtpstandard/servlet/dwindex?searchtype=NSC&outputformat=html&searchlist=118979 | |
Description | The NCI Development Therapeutics Program (DTP) provides services and resources to the academic and private-sector research communities worldwide to facilitate the discovery and development of new cancer therapeutic agents. | |
Explanation | Unless otherwise indicated, all text within NCI products is free of copyright and may be reused without our permission. Credit the National Cancer Institute as the source. | |
Retrosynthesis Analysis
AI-Powered Synthesis Planning: Our tool employs the Template_relevance Pistachio, Template_relevance Bkms_metabolic, Template_relevance Pistachio_ringbreaker, Template_relevance Reaxys, Template_relevance Reaxys_biocatalysis model, leveraging a vast database of chemical reactions to predict feasible synthetic routes.
One-Step Synthesis Focus: Specifically designed for one-step synthesis, it provides concise and direct routes for your target compounds, streamlining the synthesis process.
Accurate Predictions: Utilizing the extensive PISTACHIO, BKMS_METABOLIC, PISTACHIO_RINGBREAKER, REAXYS, REAXYS_BIOCATALYSIS database, our tool offers high-accuracy predictions, reflecting the latest in chemical research and data.
Strategy Settings
Precursor scoring | Relevance Heuristic |
---|---|
Min. plausibility | 0.01 |
Model | Template_relevance |
Template Set | Pistachio/Bkms_metabolic/Pistachio_ringbreaker/Reaxys/Reaxys_biocatalysis |
Top-N result to add to graph | 6 |
Feasible Synthetic Routes
Q1: What is the molecular formula and weight of cholest-5-en-3-one?
A1: The molecular formula of cholest-5-en-3-one is C27H44O, and its molecular weight is 384.64 g/mol. []
Q2: What spectroscopic data is available for cholest-5-en-3-one?
A2: Researchers frequently use IR, 1H NMR, and 13C NMR spectroscopy to characterize cholest-5-en-3-one. These techniques provide information about the functional groups and the carbon skeleton of the molecule. [, , , , ]
Q3: What is the role of cholest-5-en-3-one in the enzymatic conversion of cholesterol to cholest-4-en-3-one?
A3: Cholest-5-en-3-one acts as an intermediate in the reaction catalyzed by cholesterol oxidase. The enzyme first oxidizes cholesterol to cholest-5-en-3-one, which is subsequently isomerized to cholest-4-en-3-one. [, , , ]
Q4: How does the H121A mutation in cholesterol oxidase affect the isomerization of cholest-5-en-3-one?
A4: Interestingly, the H121A mutation, while impacting the flavin reduction step, does not significantly affect the isomerization of cholest-5-en-3-one to cholest-4-en-3-one. This suggests that the isomerization step is relatively independent of this particular residue. []
Q5: Can other enzymes besides cholesterol oxidase utilize cholest-5-en-3-one as a substrate?
A5: Yes, cholest-4-en-3-one-Δ1-dehydrogenase, an enzyme involved in the anoxic metabolism of cholesterol, can oxidize cholest-5-en-3-one, albeit with lower catalytic efficiency compared to its preferred substrate, cholest-4-en-3-one. []
Q6: How does the stereochemistry of cholest-5-en-3-one influence its reactions?
A6: The stereochemistry of the molecule, particularly around the double bond, plays a crucial role in determining the stereochemical outcome of reactions such as epoxidation, halogenation, and singlet oxygenation. [, , , ]
Q7: Can cholest-5-en-3-one undergo rearrangement reactions?
A7: Yes, in the presence of acids like acetic acid or trichloroacetic acid, cholest-5-en-3-one rearranges to cholest-4-en-3-one. This isomerization involves protonation and deprotonation steps, and the stereochemistry of the product is influenced by the reaction conditions. [, ]
Q8: What is a significant application of cholest-5-en-3-one in organic synthesis?
A8: Cholest-5-en-3-one serves as a versatile starting material for synthesizing a variety of steroidal compounds. Researchers have used it to create steroidal thiazolidinones, spiro-oxadiazolines, and pyridines. These syntheses highlight the utility of cholest-5-en-3-one in constructing diverse heterocyclic systems. [, , ]
Q9: Does cholest-5-en-3-one exhibit any biological activity?
A9: Research suggests that cholest-5-en-3-one might have potential anti-diabetic effects. Studies in db/db mice showed that dietary cholest-5-en-3-one could alleviate hyperglycemia and hyperinsulinemia, possibly by reducing inflammatory cytokine production via suppression of the NFκB signaling pathway. []
Q10: How does cholest-5-en-3-one interact with cell membranes?
A10: Studies in Acholeplasma laidlawii have shown that the effects of cholest-5-en-3-one on cell membranes are dependent on the acyl chain composition of the membrane lipids. While it may stimulate growth in membranes with saturated acyl chains, it can cause lysis in those with unsaturated chains. []
Q11: Can cholest-5-en-3-one be utilized by microorganisms?
A11: Yes, certain microorganisms, such as Mycoplasma mycoides, can utilize cholest-5-en-3-one for growth, but it might also inhibit the growth-promoting effects of cholesterol in other species. []
Q12: How is cholest-5-en-3-one quantified in biological samples?
A12: Gas-liquid chromatography (GLC) is commonly employed for the detection, resolution, and quantification of cholest-5-en-3-one in biological samples, especially when analyzing complex mixtures of sterols. []
Q13: Are there any biosensor applications for cholest-5-en-3-one?
A13: Researchers are exploring the use of cholest-5-en-3-one in amperometric biosensors for determining free cholesterol levels in serum samples. These biosensors leverage the electron transfer occurring during the enzymatic conversion of cholesterol to cholest-4-en-3-one via cholest-5-en-3-one. []
Haftungsausschluss und Informationen zu In-Vitro-Forschungsprodukten
Bitte beachten Sie, dass alle Artikel und Produktinformationen, die auf BenchChem präsentiert werden, ausschließlich zu Informationszwecken bestimmt sind. Die auf BenchChem zum Kauf angebotenen Produkte sind speziell für In-vitro-Studien konzipiert, die außerhalb lebender Organismen durchgeführt werden. In-vitro-Studien, abgeleitet von dem lateinischen Begriff "in Glas", beinhalten Experimente, die in kontrollierten Laborumgebungen unter Verwendung von Zellen oder Geweben durchgeführt werden. Es ist wichtig zu beachten, dass diese Produkte nicht als Arzneimittel oder Medikamente eingestuft sind und keine Zulassung der FDA für die Vorbeugung, Behandlung oder Heilung von medizinischen Zuständen, Beschwerden oder Krankheiten erhalten haben. Wir müssen betonen, dass jede Form der körperlichen Einführung dieser Produkte in Menschen oder Tiere gesetzlich strikt untersagt ist. Es ist unerlässlich, sich an diese Richtlinien zu halten, um die Einhaltung rechtlicher und ethischer Standards in Forschung und Experiment zu gewährleisten.