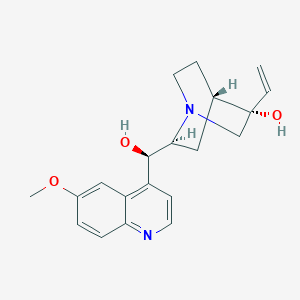
3-Hydroxychinolin
Übersicht
Beschreibung
Cinchonan-3,9-diol, 6’-methoxy-, (8alpha,9R)-: is a chemical compound known for its significant role in asymmetric organic catalysis. This compound is derived from the cinchona alkaloids, which are naturally occurring in the bark of cinchona trees. The compound has a molecular formula of C20H26N2O2 and a molecular weight of 326.4326 .
Wissenschaftliche Forschungsanwendungen
Cinchonan-3,9-diol, 6’-methoxy-, (8alpha,9R)- has extensive applications in scientific research:
Wirkmechanismus
Target of Action
3-Hydroxyquinine, a metabolite of quinine, primarily targets the malaria parasite Plasmodium falciparum . The compound is toxic to the parasite and interferes with its ability to break down and digest hemoglobin .
Mode of Action
The theorized mechanism of action for 3-hydroxyquinine and related anti-malarial drugs is that these drugs are toxic to the malaria parasite . Specifically, these drugs interfere with the parasite’s ability to break down and digest hemoglobin . This disruption in the parasite’s metabolism leads to its eventual death and clearance from the host’s body.
Biochemical Pathways
It is known that the compound is involved in the metabolism of the malaria parasite, particularly in the breakdown and digestion of hemoglobin
Pharmacokinetics
3-Hydroxyquinine is primarily eliminated via hepatic biotransformation . Approximately 20% of quinine, the parent compound of 3-hydroxyquinine, is excreted unchanged in urine . The half-life of quinine is approximately 18 hours . The clearance rates are 0.17 L/h/kg for healthy individuals and 0.09 L/h/kg for patients with uncomplicated malaria .
Result of Action
The primary result of 3-hydroxyquinine’s action is the clearance of the malaria parasite from the host’s body . By disrupting the parasite’s ability to break down and digest hemoglobin, 3-hydroxyquinine causes the death of the parasite . This leads to the resolution of the malaria infection.
Action Environment
The action of 3-hydroxyquinine can be influenced by various environmental factors. For instance, the presence of other drugs can affect the metabolism of 3-hydroxyquinine. In one study, concurrent administration of nevirapine and quinine led to significant reductions in the plasma levels of quinine and elevated plasma levels of 3-hydroxyquinine . This could potentially reduce the efficacy of quinine while increasing toxicity, since 3-hydroxyquinine has higher toxicity and lower anti-malarial activity .
Biochemische Analyse
Biochemical Properties
3-Hydroxyquinine is a product of the metabolism of quinine by the Cytochrome P450 enzymes, specifically CYPs 3A4 and 2D6 . It interacts with these enzymes to undergo hydroxylation, a biochemical reaction that introduces a hydroxyl group into the molecule .
Cellular Effects
It is known that quinine, the parent compound of 3-Hydroxyquinine, can cause oxidative stress in human erythrocytes . This suggests that 3-Hydroxyquinine may have similar effects, potentially influencing cell signaling pathways and cellular metabolism.
Molecular Mechanism
The molecular mechanism of 3-Hydroxyquinine involves interactions with the Cytochrome P450 enzymes. These enzymes catalyze the hydroxylation of quinine to form 3-Hydroxyquinine . This process may involve binding interactions with the enzymes, potentially leading to changes in enzyme activity and gene expression.
Temporal Effects in Laboratory Settings
Studies on quinine suggest that its metabolites, including 3-Hydroxyquinine, may have long-term effects on cellular function .
Dosage Effects in Animal Models
The effects of 3-Hydroxyquinine at different dosages in animal models have not been extensively studied. Given its role as a metabolite of quinine, it is likely that its effects would be similar to those of quinine, which can cause adverse effects at high doses .
Metabolic Pathways
3-Hydroxyquinine is involved in the metabolic pathways of quinine. It is formed through the action of the Cytochrome P450 enzymes, specifically CYPs 3A4 and 2D6 .
Vorbereitungsmethoden
Synthetic Routes and Reaction Conditions: The synthesis of Cinchonan-3,9-diol, 6’-methoxy-, (8alpha,9R)- typically involves the modification of naturally occurring cinchona alkaloids. The process includes several steps such as methylation and reduction. Specific reaction conditions, such as temperature and pH, are carefully controlled to ensure the desired stereochemistry is achieved .
Industrial Production Methods: Industrial production of this compound often involves large-scale extraction from cinchona bark followed by chemical modification. The process is optimized for yield and purity, employing advanced techniques such as chromatography for purification.
Analyse Chemischer Reaktionen
Types of Reactions: Cinchonan-3,9-diol, 6’-methoxy-, (8alpha,9R)- undergoes various types of chemical reactions, including:
Oxidation: This reaction can be facilitated by reagents such as potassium permanganate or chromium trioxide.
Reduction: Common reducing agents include lithium aluminum hydride and sodium borohydride.
Substitution: This compound can participate in nucleophilic substitution reactions, often using reagents like alkyl halides.
Common Reagents and Conditions:
Oxidation: Potassium permanganate in acidic conditions.
Reduction: Lithium aluminum hydride in anhydrous ether.
Substitution: Alkyl halides in the presence of a base.
Major Products Formed:
Oxidation: Formation of ketones or carboxylic acids.
Reduction: Formation of alcohols.
Substitution: Formation of substituted cinchona derivatives.
Vergleich Mit ähnlichen Verbindungen
- Cinchonan-9-ol, 10,11-dihydro-6’-methoxy-, (8alpha,9R)-
- Cinchonan-3,9-diol, 6’-methoxy-, (3alpha,8alpha,9R)-(9CI)
Comparison: Cinchonan-3,9-diol, 6’-methoxy-, (8alpha,9R)- is unique due to its specific stereochemistry, which imparts distinct catalytic properties. Compared to similar compounds, it offers higher selectivity and efficiency in asymmetric synthesis .
Biologische Aktivität
3-Hydroxyquinine (3-OHQ) is a significant metabolite of quinine, an alkaloid with well-documented antimalarial properties. The biological activity of 3-hydroxyquinine has garnered attention due to its implications in pharmacokinetics, therapeutic efficacy, and potential side effects. This article explores the biological activity of 3-hydroxyquinine through detailed research findings, case studies, and data tables.
Overview of 3-Hydroxyquinine
3-Hydroxyquinine is primarily formed through the metabolism of quinine by cytochrome P450 enzymes, particularly CYP3A4. This metabolite retains a portion of the antimalarial activity associated with quinine itself. Studies have indicated that 3-OHQ contributes approximately 12% to the overall antimalarial efficacy of quinine in patients suffering from falciparum malaria .
Pharmacokinetics
The pharmacokinetic profile of 3-hydroxyquinine reveals important insights into its absorption, distribution, metabolism, and excretion:
- Absorption : Following the administration of quinine, 3-hydroxyquinine is rapidly detectable in plasma.
- Distribution : The distribution volume for both quinine and its metabolite is significant, indicating extensive tissue binding.
- Metabolism : The formation of 3-hydroxyquinine is mediated by CYP3A4; thus, factors affecting this enzyme can influence the levels of 3-OHQ in the body.
- Excretion : Renal clearance plays a crucial role in the elimination of both quinine and 3-hydroxyquinine.
Table 1: Pharmacokinetic Parameters of Quinine and 3-Hydroxyquinine
Parameter | Quinine | 3-Hydroxyquinine |
---|---|---|
Half-life (hours) | 11-12 | 10-12 |
Volume of Distribution | ~2 L/kg | ~1.5 L/kg |
Clearance (L/h) | ~10 | ~8 |
Bioavailability (%) | ~70 | - |
Antimalarial Efficacy
Research indicates that while quinine is a potent antimalarial agent, its metabolite, 3-hydroxyquinine, also exhibits antimalarial properties. A study demonstrated that patients treated with quinine showed measurable plasma concentrations of 3-OHQ that correlated with therapeutic outcomes .
Drug Interactions
The metabolism of quinine to 3-hydroxyquinine can be influenced by various factors including drug interactions. For instance, co-administration with CYP3A4 inhibitors such as troleandomycin significantly reduces the formation clearance of 3-OHQ by approximately 81% . This highlights the importance of considering potential drug interactions when prescribing quinine.
Case Studies
Case Study 1 : A clinical trial involving healthy volunteers assessed the impact of troleandomycin on the pharmacokinetics of quinine and its metabolite. Results indicated that inhibition of CYP3A4 led to increased plasma levels of quinine while significantly decreasing those of 3-hydroxyquinine, suggesting that monitoring these metabolites could be essential in clinical settings .
Case Study 2 : In patients with liver disease, altered metabolism was observed for both quinine and its metabolite. The disposition studies indicated that liver impairment could lead to increased levels of 3-hydroxyquinine, which may contribute to enhanced pharmacological effects or toxicity .
Eigenschaften
IUPAC Name |
(3S,4S,6S)-3-ethenyl-6-[(R)-hydroxy-(6-methoxyquinolin-4-yl)methyl]-1-azabicyclo[2.2.2]octan-3-ol | |
---|---|---|
Source | PubChem | |
URL | https://pubchem.ncbi.nlm.nih.gov | |
Description | Data deposited in or computed by PubChem | |
InChI |
InChI=1S/C20H24N2O3/c1-3-20(24)12-22-9-7-13(20)10-18(22)19(23)15-6-8-21-17-5-4-14(25-2)11-16(15)17/h3-6,8,11,13,18-19,23-24H,1,7,9-10,12H2,2H3/t13-,18-,19+,20+/m0/s1 | |
Source | PubChem | |
URL | https://pubchem.ncbi.nlm.nih.gov | |
Description | Data deposited in or computed by PubChem | |
InChI Key |
BSRUJCFCZKMFMB-YGHPHNMRSA-N | |
Source | PubChem | |
URL | https://pubchem.ncbi.nlm.nih.gov | |
Description | Data deposited in or computed by PubChem | |
Canonical SMILES |
COC1=CC2=C(C=CN=C2C=C1)C(C3CC4CCN3CC4(C=C)O)O | |
Source | PubChem | |
URL | https://pubchem.ncbi.nlm.nih.gov | |
Description | Data deposited in or computed by PubChem | |
Isomeric SMILES |
COC1=CC2=C(C=CN=C2C=C1)[C@H]([C@@H]3C[C@@H]4CCN3C[C@@]4(C=C)O)O | |
Source | PubChem | |
URL | https://pubchem.ncbi.nlm.nih.gov | |
Description | Data deposited in or computed by PubChem | |
Molecular Formula |
C20H24N2O3 | |
Source | PubChem | |
URL | https://pubchem.ncbi.nlm.nih.gov | |
Description | Data deposited in or computed by PubChem | |
DSSTOX Substance ID |
DTXSID901316798 | |
Record name | 3-Hydroxyquinine | |
Source | EPA DSSTox | |
URL | https://comptox.epa.gov/dashboard/DTXSID901316798 | |
Description | DSSTox provides a high quality public chemistry resource for supporting improved predictive toxicology. | |
Molecular Weight |
340.4 g/mol | |
Source | PubChem | |
URL | https://pubchem.ncbi.nlm.nih.gov | |
Description | Data deposited in or computed by PubChem | |
CAS No. |
78549-61-8 | |
Record name | 3-Hydroxyquinine | |
Source | CAS Common Chemistry | |
URL | https://commonchemistry.cas.org/detail?cas_rn=78549-61-8 | |
Description | CAS Common Chemistry is an open community resource for accessing chemical information. Nearly 500,000 chemical substances from CAS REGISTRY cover areas of community interest, including common and frequently regulated chemicals, and those relevant to high school and undergraduate chemistry classes. This chemical information, curated by our expert scientists, is provided in alignment with our mission as a division of the American Chemical Society. | |
Explanation | The data from CAS Common Chemistry is provided under a CC-BY-NC 4.0 license, unless otherwise stated. | |
Record name | 3-Hydroxyquinine | |
Source | ChemIDplus | |
URL | https://pubchem.ncbi.nlm.nih.gov/substance/?source=chemidplus&sourceid=0078549618 | |
Description | ChemIDplus is a free, web search system that provides access to the structure and nomenclature authority files used for the identification of chemical substances cited in National Library of Medicine (NLM) databases, including the TOXNET system. | |
Record name | 3-Hydroxyquinine | |
Source | EPA DSSTox | |
URL | https://comptox.epa.gov/dashboard/DTXSID901316798 | |
Description | DSSTox provides a high quality public chemistry resource for supporting improved predictive toxicology. | |
Record name | 3-HYDROXYQUININE | |
Source | FDA Global Substance Registration System (GSRS) | |
URL | https://gsrs.ncats.nih.gov/ginas/app/beta/substances/4D7695509M | |
Description | The FDA Global Substance Registration System (GSRS) enables the efficient and accurate exchange of information on what substances are in regulated products. Instead of relying on names, which vary across regulatory domains, countries, and regions, the GSRS knowledge base makes it possible for substances to be defined by standardized, scientific descriptions. | |
Explanation | Unless otherwise noted, the contents of the FDA website (www.fda.gov), both text and graphics, are not copyrighted. They are in the public domain and may be republished, reprinted and otherwise used freely by anyone without the need to obtain permission from FDA. Credit to the U.S. Food and Drug Administration as the source is appreciated but not required. | |
Retrosynthesis Analysis
AI-Powered Synthesis Planning: Our tool employs the Template_relevance Pistachio, Template_relevance Bkms_metabolic, Template_relevance Pistachio_ringbreaker, Template_relevance Reaxys, Template_relevance Reaxys_biocatalysis model, leveraging a vast database of chemical reactions to predict feasible synthetic routes.
One-Step Synthesis Focus: Specifically designed for one-step synthesis, it provides concise and direct routes for your target compounds, streamlining the synthesis process.
Accurate Predictions: Utilizing the extensive PISTACHIO, BKMS_METABOLIC, PISTACHIO_RINGBREAKER, REAXYS, REAXYS_BIOCATALYSIS database, our tool offers high-accuracy predictions, reflecting the latest in chemical research and data.
Strategy Settings
Precursor scoring | Relevance Heuristic |
---|---|
Min. plausibility | 0.01 |
Model | Template_relevance |
Template Set | Pistachio/Bkms_metabolic/Pistachio_ringbreaker/Reaxys/Reaxys_biocatalysis |
Top-N result to add to graph | 6 |
Feasible Synthetic Routes
Q1: What is the primary metabolic pathway of quinine in humans?
A1: The primary metabolic pathway of quinine in humans is 3-hydroxylation, resulting in the formation of 3-hydroxyquinine. [, , ] This metabolic process is predominantly mediated by the cytochrome P450 enzyme, specifically CYP3A4. [, , ]
Q2: Which cytochrome P450 (CYP) enzyme is primarily responsible for the formation of 3-hydroxyquinine?
A2: CYP3A4 is the major enzyme responsible for the 3-hydroxylation of quinine, leading to the formation of 3-hydroxyquinine. [, , , ] While CYP2C19 also plays a minor role, other CYP isoforms seem to have negligible involvement in this metabolic pathway. []
Q3: What is the significance of 3-hydroxyquinine in the context of quinine's antimalarial activity?
A3: 3-Hydroxyquinine, the major metabolite of quinine, also contributes to its antimalarial activity. [, ] This highlights the importance of understanding the factors influencing 3-hydroxyquinine formation and its levels in the body.
Q4: How do liver diseases impact the metabolism of quinine and 3-hydroxyquinine?
A4: Studies show that patients with liver diseases exhibit compromised metabolism of quinine, leading to increased levels of quinine and decreased levels of 3-hydroxyquinine compared to healthy individuals. [] This suggests a need to adjust quinine dosage in patients with compromised liver function, especially those with malaria, to avoid potential toxicity.
Q5: How do antimalarial drugs like doxycycline, primaquine, and tetracycline affect quinine metabolism?
A5: These antimalarial drugs have been shown to inhibit quinine 3-hydroxylation to varying degrees. Doxycycline exhibits the most potent inhibition, followed by primaquine and tetracycline. [] This interaction could potentially lead to altered quinine levels when co-administered with these drugs.
Q6: Can rifampin be co-administered with quinine for malaria treatment?
A6: Despite initially shorter parasite clearance times observed in patients receiving both rifampin and quinine, the combination led to significantly higher recrudescence rates compared to quinine alone. [] This negative impact on cure rates is attributed to rifampin’s ability to enhance quinine’s metabolic clearance, leading to significantly lower quinine plasma concentrations. [] Therefore, rifampin is not recommended for co-administration with quinine.
Q7: How does co-administration of quinine with CYP3A4 inhibitors like ketoconazole, troleandomycin, and ritonavir impact quinine levels?
A7: Co-administration with potent CYP3A4 inhibitors like ketoconazole, troleandomycin, and ritonavir has been shown to significantly increase plasma concentrations of quinine. [, , , , ] This interaction necessitates careful consideration and potential dosage adjustments when these drugs are used concurrently with quinine.
Q8: Do herbal supplements like grapefruit juice, black cohosh, grape seed extract, green tea extract, and ginseng impact quinine metabolism?
A8: While black cohosh and ginseng showed minimal impact, grapefruit juice and green tea extract demonstrated significant inhibition of CYP3A4 activity, potentially affecting the metabolism of drugs like quinine. [, ] Grape seed extract exhibited variable inhibition, suggesting potential product-specific effects. []
Q9: Does the CYP3A5 genotype influence quinine metabolism?
A9: Yes, studies show a significant association between the CYP3A5 genotype and quinine 3-hydroxylation. [] Individuals with high CYP3A5 expression tend to metabolize quinine faster, highlighting the role of pharmacogenetics in quinine disposition. [, , ]
Q10: How does sex affect the pharmacokinetics of quinine?
A10: Women generally display higher quinine bioavailability than men, despite being faster metabolizers. [] This emphasizes the influence of sex on quinine disposition and highlights the complex interplay of factors affecting drug response.
Q11: What are some analytical techniques employed for quantifying quinine and 3-hydroxyquinine in biological samples?
A11: High-performance liquid chromatography (HPLC) is a widely utilized technique for measuring quinine and 3-hydroxyquinine concentrations in various biological matrices. [, , , , , , , ] Methods employing gas chromatography-mass spectrometry have also been used to identify urinary metabolites of quinine. []
Q12: How effective is the quinine/3-hydroxyquinine metabolic ratio as a marker for CYP3A induction compared to other markers?
A12: Studies indicate that the quinine/3-hydroxyquinine metabolic ratio is comparable in efficacy to midazolam clearance and the 4β-hydroxycholesterol/cholesterol ratio as a marker for CYP3A induction. [, ] Its advantage lies in requiring only a single blood sample for analysis. [, ]
Q13: What is the role of metabonomics in understanding variability in CYP3A4 induction?
A13: Metabonomics, utilizing techniques like 1H NMR spectroscopy, has shown potential in identifying metabolic signatures associated with CYP3A4 activity. [] Studies using metabonomic approaches have successfully predicted CYP3A4-mediated drug response, highlighting its future potential in personalized medicine. []
Q14: What is known about the structure of 3-hydroxyquinine?
A14: The structure of 3-hydroxyquinine has been confirmed through the synthesis and X-ray crystal structure analysis of its 9-aceto analogue. [] This confirmation sheds light on the stereochemistry of this important metabolite.
Q15: Have any new urinary metabolites of quinine been identified in humans?
A15: Research using methane chemical ionization gas chromatography-mass spectrometry has led to the identification of six new urinary metabolites of quinine in humans. [] These findings contribute to a more complete understanding of quinine metabolism in the body.
Q16: What is the role of aldehyde oxidase in the metabolism of cinchona alkaloids like quinine?
A16: Aldehyde oxidase, particularly in rabbit liver, plays a significant role in the oxidative metabolism of cinchona alkaloids, including quinine. [] This metabolic pathway involves the oxidation of these alkaloids at carbon 2 of the quinoline ring, forming the corresponding lactams. []
Haftungsausschluss und Informationen zu In-Vitro-Forschungsprodukten
Bitte beachten Sie, dass alle Artikel und Produktinformationen, die auf BenchChem präsentiert werden, ausschließlich zu Informationszwecken bestimmt sind. Die auf BenchChem zum Kauf angebotenen Produkte sind speziell für In-vitro-Studien konzipiert, die außerhalb lebender Organismen durchgeführt werden. In-vitro-Studien, abgeleitet von dem lateinischen Begriff "in Glas", beinhalten Experimente, die in kontrollierten Laborumgebungen unter Verwendung von Zellen oder Geweben durchgeführt werden. Es ist wichtig zu beachten, dass diese Produkte nicht als Arzneimittel oder Medikamente eingestuft sind und keine Zulassung der FDA für die Vorbeugung, Behandlung oder Heilung von medizinischen Zuständen, Beschwerden oder Krankheiten erhalten haben. Wir müssen betonen, dass jede Form der körperlichen Einführung dieser Produkte in Menschen oder Tiere gesetzlich strikt untersagt ist. Es ist unerlässlich, sich an diese Richtlinien zu halten, um die Einhaltung rechtlicher und ethischer Standards in Forschung und Experiment zu gewährleisten.