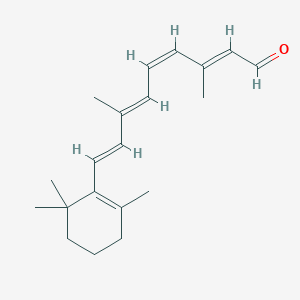
11-cis-Retinal
Übersicht
Beschreibung
Rhodopsin, auch bekannt als Sehpurpur, ist ein lichtempfindliches Rezeptorprotein, das in den Stäbchen der Netzhaut vorkommt. Es ist ein G-Protein-gekoppelter Rezeptor (GPCR), der eine entscheidende Rolle im Phototransduktionsweg spielt und das Sehen bei schlechten Lichtverhältnissen ermöglicht. Rhodopsin besteht aus dem Protein Opsin und einem kovalent gebundenen Retinal-Chromophor. Bei Lichteinwirkung erfährt Rhodopsin eine Konformationsänderung, die eine Kaskade biochemischer Reaktionen auslöst, die letztendlich zur visuellen Wahrnehmung führen .
Herstellungsmethoden
Synthetische Wege und Reaktionsbedingungen
Rhodopsin kann mit verschiedenen Methoden synthetisiert und gereinigt werden. Ein gängiger Ansatz beinhaltet die Expression von Rhodopsin in Säugetierzellen, wie z. B. HEK293-Zellen. Die Zellen werden mit einem Plasmid transfiziert, das das Rhodopsingen kodiert, und das Protein wird anschließend mit Affinitätschromatographie extrahiert und gereinigt .
Industrielle Produktionsmethoden
Die industrielle Produktion von Rhodopsin erfolgt typischerweise in großtechnischen Zellkultursystemen. Die Zellen werden in Bioreaktoren gezüchtet, und das Rhodopsinprotein wird mit ähnlichen Techniken geerntet und gereinigt wie bei der Produktion im Labormaßstab. Fortschritte in der Proteintechnik und in den Reinigungsprotokollen haben die Ausbeute und die Qualität des industriell produzierten Rhodopsins verbessert .
Wissenschaftliche Forschungsanwendungen
Rhodopsin has numerous applications in scientific research, particularly in the fields of chemistry, biology, medicine, and industry. In optogenetics, rhodopsin and its microbial counterparts are used to control cellular processes with light, enabling precise manipulation of neuronal activity . Rhodopsin is also studied extensively to understand the molecular mechanisms of vision and to develop treatments for retinal diseases such as retinitis pigmentosa and congenital stationary night blindness .
Wirkmechanismus
Target of Action
The primary target of 11-cis-retinal is the protein opsin . Opsin is found in the mammalian visual system, where this compound binds to it as an inverse agonist, forming the inactive conformation of rhodopsin . Rhodopsin is a compound responsible for vision in low-light conditions and is the biologically active component of rod photoreceptors .
Mode of Action
Upon absorption of a photon of light, this compound undergoes isomerization to form all-trans-retinal . This isomerization is the initiating event for vision in vertebrates . The isomerization process involves promoting a p electron in the pi bond to a higher-energy orbital, which temporarily converts the double bond into a single bond . This allows the molecule to rotate around this single bond .
Biochemical Pathways
The isomerization of this compound to all-trans-retinal triggers a biochemical cascade culminating in the closure of cGMP-gated channels and hyperpolarization of the cell . This process is part of the visual cycle , a series of enzymatic reactions that regenerate this compound, the chromophore of rod and cone opsins . The visual cycle involves the cleavage and isomerization of all-trans-retinyl fatty acid esters to 11-cis-retinol, which is further oxidized by 11-cis retinol dehydrogenase to this compound for use as a visual chromophore .
Pharmacokinetics
The pharmacokinetics of this compound are closely tied to its role in the visual cycle. Retinol, the common precursor of active retinoids, is transported in plasma in a 1:1 complex with retinol-binding protein . Following uptake by target cells, retinol is converted, through several enzymatic steps, into its active derivatives . The final step in the retinoid visual cycle is catalyzed by 11-cis-retinol dehydrogenases (11-cis-RDHs) that oxidize 11-cis-retinol to this compound .
Result of Action
The result of this compound’s action is the initiation of the phototransduction cycle , which is the basis for mammalian vision . The isomerization of this compound to all-trans-retinal triggers a series of events that ultimately lead to the conversion of light into electrical signals . This process is essential for vision, growth, and development .
Action Environment
The action of this compound is influenced by environmental factors such as light. The isomerization of this compound to all-trans-retinal is triggered by the absorption of a photon of light . Furthermore, the regeneration of this compound, necessary for sustained visual function, is an endergonic process normally conducted by specialized enzyme systems . This compound can also be formed through reverse photoisomerization from all-trans-retinal upon light exposure .
Safety and Hazards
Under moderate light exposure conditions, all-trans-retinal is continuously recycled to 11-cis-retinal by RPE cells and is not hazardous for cells . When light exposure is longer or more intense, all-trans-retinal accumulates and its activation by blue light can cause oxidative stress that damages the cellular components of the outer segment of the photoreceptors .
Zukünftige Richtungen
There are ongoing research and clinical trials exploring the therapeutic potential of targeting IRBP in retinal diseases . Manipulating the expression of IRBP in the retina could potentially rescue or prevent photoreceptor degeneration in many retinal diseases . Another promising direction is the use of synthetic 9-cis-retinyl acetate as a replacement for the missing 11-cis-retinal .
Biochemische Analyse
Biochemical Properties
11-cis-Retinal interacts with various enzymes and proteins in the visual system. It is involved in the regeneration of visual pigments in vertebrates . The pigments that employ c-opsins rely on a dark, enzymatically catalyzed isomerization process . The toxicity of retinal and its potential for oxidation require that chaperones bind and sequester the retinoid and that dehydrogenases are available to reduce it to the less toxic retinol .
Cellular Effects
This compound has significant effects on various types of cells and cellular processes. Exposure to high light intensities or continuous illumination can result in oxidative stress within these cells, leading to a loss of their functionality .
Molecular Mechanism
The molecular mechanism of this compound involves the absorption of a photon by the chromophore of these systems, resulting in an isomerization in which the cis-double bond at position 11 is converted to a trans-double bond . This 11-cis to all-trans-retinal photoisomerization is a key event because the change in shape of the chromophore is transmitted to the opsin, causing it to change its conformation and to interact with a G protein downstream in a signal transduction pathway .
Temporal Effects in Laboratory Settings
The effects of this compound over time in laboratory settings have been observed. For instance, single doses of 9-cis-Retinal, an analogue of this compound, provided significant dose-dependent improvement in electroretinographic responses . This suggests that this compound may have similar temporal effects in laboratory settings.
Dosage Effects in Animal Models
The effects of this compound vary with different dosages in animal models. For instance, treatment with 9-cis-Retinal, an analogue of this compound, improves visual function and preserves retinal morphology in Rpe65 −/− mice . This suggests that this compound may have similar dosage effects in animal models.
Metabolic Pathways
This compound is involved in several metabolic pathways. It is a critical component of the visual cycle, a complex process involving photoreceptors and adjacent retinal pigment epithelial cells (RPE) . The regeneration of this compound is critical for sustaining vision in vertebrates .
Transport and Distribution
This compound is transported and distributed within cells and tissues. It is coupled to opsins in both rod and cone photoreceptor cells and is photo-isomerized to all-trans-retinal by light . This suggests that this compound is transported and distributed within cells and tissues in a light-dependent manner.
Subcellular Localization
The subcellular localization of this compound suggests that it is present in the outer segments of cone photoreceptors . This indicates that the enzymatic machinery required for the oxidation of recycled cis retinol as part of the retina visual cycle is present in the outer segments of cones .
Vorbereitungsmethoden
Synthetic Routes and Reaction Conditions
Rhodopsin can be synthesized and purified using various methods. One common approach involves the expression of rhodopsin in mammalian cells, such as HEK293 cells. The cells are transfected with a plasmid encoding the rhodopsin gene, and the protein is subsequently extracted and purified using affinity chromatography .
Industrial Production Methods
Industrial production of rhodopsin typically involves large-scale cell culture systems. The cells are grown in bioreactors, and the rhodopsin protein is harvested and purified using similar techniques as those used in laboratory-scale production. Advances in protein engineering and purification protocols have improved the yield and quality of rhodopsin produced industrially .
Analyse Chemischer Reaktionen
Reaktionstypen
Rhodopsin durchläuft verschiedene Arten chemischer Reaktionen, die hauptsächlich seinen Retinal-Chromophor betreffen. Die bemerkenswerteste Reaktion ist die Photoisomerisierung von 11-cis-Retinal zu all-trans-Retinal nach Absorption eines Photons. Diese Isomerisierung löst eine Reihe von Konformationsänderungen im Opsin-Protein aus, die zur Aktivierung der Phototransduktionskaskade führen .
Häufige Reagenzien und Bedingungen
Die Photoisomerisierungsreaktion von Rhodopsin erfordert Licht als Hauptreagenz. Im Labor kann diese Reaktion mit Hilfe der Hochleistungsflüssigkeitschromatographie (HPLC) untersucht werden, um die Isomerisierungsprodukte zu analysieren .
Wichtige gebildete Produkte
Das Hauptprodukt der Photoisomerisierungsreaktion ist all-trans-Retinal. Dieses Molekül dissoziiert anschließend vom Opsin-Protein und führt zur Bildung von Metarhodopsin II, einem aktivierten Zustand von Rhodopsin, der mit dem G-Protein Transducin interagiert, um das visuelle Signal zu verstärken .
Wissenschaftliche Forschungsanwendungen
Rhodopsin hat zahlreiche Anwendungen in der wissenschaftlichen Forschung, insbesondere in den Bereichen Chemie, Biologie, Medizin und Industrie. In der Optogenetik werden Rhodopsin und seine mikrobiellen Gegenstücke verwendet, um zelluläre Prozesse mit Licht zu steuern, was eine präzise Manipulation der neuronalen Aktivität ermöglicht . Rhodopsin wird auch ausgiebig untersucht, um die molekularen Mechanismen des Sehens zu verstehen und Behandlungen für Netzhauterkrankungen wie Retinitis pigmentosa und angeborene stationäre Nachtblindheit zu entwickeln .
Wirkmechanismus
Rhodopsin übt seine Wirkung über einen genau definierten Mechanismus aus, der die Aktivierung der Phototransduktionskaskade beinhaltet. Nach Absorption von Licht isomerisiert this compound zu all-trans-Retinal, wodurch sich das Opsin-Protein konformationsmäßig verändert. Diese Veränderung aktiviert das G-Protein Transducin, das wiederum die Phosphodiesterase aktiviert, was zu einer Abnahme des zyklischen Guanosinmonophosphats (cGMP) führt. Die Abnahme von cGMP führt zur Schließung von cGMP-gesteuerten Ionenkanälen, was zu einer Hyperpolarisation der Stäbchenzelle und der Übertragung des visuellen Signals zum Gehirn führt .
Vergleich Mit ähnlichen Verbindungen
Während tierisches Rhodopsin wie Seh-Rhodopsin am Sehen beteiligt ist, haben mikrobielles Rhodopsin verschiedene Funktionen, darunter Ionentransport und lichtgetriebene Energieproduktion . Der wichtigste Unterschied zwischen diesen Typen liegt in ihren Funktionsrollen und den spezifischen Isomerisierungsreaktionen, die sie durchlaufen. So fungiert Bacteriorhodopsin als lichtgetriebene Protonenpumpe, während Halorhodopsin als Chloridionenpumpe wirkt .
Ähnliche Verbindungen
Bacteriorhodopsin: Ein mikrobielles Rhodopsin, das als lichtgetriebene Protonenpumpe fungiert.
Halorhodopsin: Ein mikrobielles Rhodopsin, das als Chloridionenpumpe wirkt.
Channelrhodopsin: Ein mikrobielles Rhodopsin, das in der Optogenetik verwendet wird, um die neuronale Aktivität mit Licht zu steuern
Die einzigartige Rolle von Rhodopsin im Sehen und seine weitreichenden Anwendungen in der wissenschaftlichen Forschung machen es zu einer Verbindung von großem Interesse in verschiedenen Disziplinen.
Eigenschaften
IUPAC Name |
(2E,4Z,6E,8E)-3,7-dimethyl-9-(2,6,6-trimethylcyclohexen-1-yl)nona-2,4,6,8-tetraenal | |
---|---|---|
Source | PubChem | |
URL | https://pubchem.ncbi.nlm.nih.gov | |
Description | Data deposited in or computed by PubChem | |
InChI |
InChI=1S/C20H28O/c1-16(8-6-9-17(2)13-15-21)11-12-19-18(3)10-7-14-20(19,4)5/h6,8-9,11-13,15H,7,10,14H2,1-5H3/b9-6-,12-11+,16-8+,17-13+ | |
Source | PubChem | |
URL | https://pubchem.ncbi.nlm.nih.gov | |
Description | Data deposited in or computed by PubChem | |
InChI Key |
NCYCYZXNIZJOKI-IOUUIBBYSA-N | |
Source | PubChem | |
URL | https://pubchem.ncbi.nlm.nih.gov | |
Description | Data deposited in or computed by PubChem | |
Canonical SMILES |
CC1=C(C(CCC1)(C)C)C=CC(=CC=CC(=CC=O)C)C | |
Source | PubChem | |
URL | https://pubchem.ncbi.nlm.nih.gov | |
Description | Data deposited in or computed by PubChem | |
Isomeric SMILES |
CC1=C(C(CCC1)(C)C)/C=C/C(=C/C=C\C(=C\C=O)\C)/C | |
Source | PubChem | |
URL | https://pubchem.ncbi.nlm.nih.gov | |
Description | Data deposited in or computed by PubChem | |
Molecular Formula |
C20H28O | |
Source | PubChem | |
URL | https://pubchem.ncbi.nlm.nih.gov | |
Description | Data deposited in or computed by PubChem | |
DSSTOX Substance ID |
DTXSID80415057 | |
Record name | 11-cis-Retinal | |
Source | EPA DSSTox | |
URL | https://comptox.epa.gov/dashboard/DTXSID80415057 | |
Description | DSSTox provides a high quality public chemistry resource for supporting improved predictive toxicology. | |
Molecular Weight |
284.4 g/mol | |
Source | PubChem | |
URL | https://pubchem.ncbi.nlm.nih.gov | |
Description | Data deposited in or computed by PubChem | |
Physical Description |
Solid | |
Record name | 11-cis-Retinaldehyde | |
Source | Human Metabolome Database (HMDB) | |
URL | http://www.hmdb.ca/metabolites/HMDB0002152 | |
Description | The Human Metabolome Database (HMDB) is a freely available electronic database containing detailed information about small molecule metabolites found in the human body. | |
Explanation | HMDB is offered to the public as a freely available resource. Use and re-distribution of the data, in whole or in part, for commercial purposes requires explicit permission of the authors and explicit acknowledgment of the source material (HMDB) and the original publication (see the HMDB citing page). We ask that users who download significant portions of the database cite the HMDB paper in any resulting publications. | |
CAS No. |
564-87-4 | |
Record name | 11-cis-Retinal | |
Source | CAS Common Chemistry | |
URL | https://commonchemistry.cas.org/detail?cas_rn=564-87-4 | |
Description | CAS Common Chemistry is an open community resource for accessing chemical information. Nearly 500,000 chemical substances from CAS REGISTRY cover areas of community interest, including common and frequently regulated chemicals, and those relevant to high school and undergraduate chemistry classes. This chemical information, curated by our expert scientists, is provided in alignment with our mission as a division of the American Chemical Society. | |
Explanation | The data from CAS Common Chemistry is provided under a CC-BY-NC 4.0 license, unless otherwise stated. | |
Record name | Retinal, (11Z)- | |
Source | ChemIDplus | |
URL | https://pubchem.ncbi.nlm.nih.gov/substance/?source=chemidplus&sourceid=0000564874 | |
Description | ChemIDplus is a free, web search system that provides access to the structure and nomenclature authority files used for the identification of chemical substances cited in National Library of Medicine (NLM) databases, including the TOXNET system. | |
Record name | 11-cis-Retinal | |
Source | EPA DSSTox | |
URL | https://comptox.epa.gov/dashboard/DTXSID80415057 | |
Description | DSSTox provides a high quality public chemistry resource for supporting improved predictive toxicology. | |
Record name | RETINAL, (11Z)- | |
Source | FDA Global Substance Registration System (GSRS) | |
URL | https://gsrs.ncats.nih.gov/ginas/app/beta/substances/91058V6HCH | |
Description | The FDA Global Substance Registration System (GSRS) enables the efficient and accurate exchange of information on what substances are in regulated products. Instead of relying on names, which vary across regulatory domains, countries, and regions, the GSRS knowledge base makes it possible for substances to be defined by standardized, scientific descriptions. | |
Explanation | Unless otherwise noted, the contents of the FDA website (www.fda.gov), both text and graphics, are not copyrighted. They are in the public domain and may be republished, reprinted and otherwise used freely by anyone without the need to obtain permission from FDA. Credit to the U.S. Food and Drug Administration as the source is appreciated but not required. | |
Record name | 11-cis-Retinaldehyde | |
Source | Human Metabolome Database (HMDB) | |
URL | http://www.hmdb.ca/metabolites/HMDB0002152 | |
Description | The Human Metabolome Database (HMDB) is a freely available electronic database containing detailed information about small molecule metabolites found in the human body. | |
Explanation | HMDB is offered to the public as a freely available resource. Use and re-distribution of the data, in whole or in part, for commercial purposes requires explicit permission of the authors and explicit acknowledgment of the source material (HMDB) and the original publication (see the HMDB citing page). We ask that users who download significant portions of the database cite the HMDB paper in any resulting publications. | |
Melting Point |
63.5 - 64.4 °C | |
Record name | 11-cis-Retinaldehyde | |
Source | Human Metabolome Database (HMDB) | |
URL | http://www.hmdb.ca/metabolites/HMDB0002152 | |
Description | The Human Metabolome Database (HMDB) is a freely available electronic database containing detailed information about small molecule metabolites found in the human body. | |
Explanation | HMDB is offered to the public as a freely available resource. Use and re-distribution of the data, in whole or in part, for commercial purposes requires explicit permission of the authors and explicit acknowledgment of the source material (HMDB) and the original publication (see the HMDB citing page). We ask that users who download significant portions of the database cite the HMDB paper in any resulting publications. | |
Retrosynthesis Analysis
AI-Powered Synthesis Planning: Our tool employs the Template_relevance Pistachio, Template_relevance Bkms_metabolic, Template_relevance Pistachio_ringbreaker, Template_relevance Reaxys, Template_relevance Reaxys_biocatalysis model, leveraging a vast database of chemical reactions to predict feasible synthetic routes.
One-Step Synthesis Focus: Specifically designed for one-step synthesis, it provides concise and direct routes for your target compounds, streamlining the synthesis process.
Accurate Predictions: Utilizing the extensive PISTACHIO, BKMS_METABOLIC, PISTACHIO_RINGBREAKER, REAXYS, REAXYS_BIOCATALYSIS database, our tool offers high-accuracy predictions, reflecting the latest in chemical research and data.
Strategy Settings
Precursor scoring | Relevance Heuristic |
---|---|
Min. plausibility | 0.01 |
Model | Template_relevance |
Template Set | Pistachio/Bkms_metabolic/Pistachio_ringbreaker/Reaxys/Reaxys_biocatalysis |
Top-N result to add to graph | 6 |
Feasible Synthetic Routes
Q1: What is 11-cis-retinal and why is it important?
A1: this compound is a light-sensitive molecule crucial for vision in vertebrates. It acts as the chromophore, the light-absorbing part, of visual pigments found in rod and cone photoreceptor cells of the retina [, , , ].
Q2: How does this compound interact with opsins to enable vision?
A2: this compound binds covalently to opsin proteins via a Schiff base linkage to form visual pigments like rhodopsin in rods and cone opsins in cones [, , , , ]. This binding occurs at a specific lysine residue (Lys-296 in rhodopsin) []. Upon absorbing light, this compound isomerizes to all-trans-retinal, triggering a conformational change in the opsin, which initiates the phototransduction cascade and ultimately leads to vision [, , , , ].
Q3: What happens to the all-trans-retinal after it absorbs light?
A3: After photoisomerization, all-trans-retinal is released from the opsin. It undergoes a series of enzymatic reactions known as the visual cycle to be converted back to this compound. This cycle involves both photoreceptor cells and the retinal pigment epithelium (RPE) [, , , , , ].
Q4: Can you describe the structural characteristics of this compound?
A4: this compound is an aldehyde and a polyene chromophore. While its exact molecular weight can vary slightly depending on the source of vitamin A it is derived from, its molecular formula is C20H28O []. Spectroscopically, this compound, when bound to opsin, exhibits an absorption maximum (λmax) that dictates the wavelength of light to which the visual pigment is most sensitive. This λmax varies depending on the specific opsin it is bound to [, , , ].
Q5: How does the structure of this compound influence its function?
A5: The specific cis conformation at the 11th carbon in this compound is crucial for its interaction with opsins. Other cis isomers, while capable of forming pigments with opsins, have different binding affinities and may not support efficient phototransduction [, , ]. Even subtle changes in the chromophore binding pocket of opsins, as seen in some mutants, can significantly impact this compound binding and the phototransduction process [, , ].
Q6: What is the role of the retinal pigment epithelium (RPE) in the visual cycle?
A6: The RPE plays a critical role in the visual cycle by providing a continuous supply of this compound to photoreceptor cells [, , , , ]. This involves enzymatic isomerization of all-trans-retinal back to this compound within the RPE, followed by transport to photoreceptor outer segments for pigment regeneration [, , , , , ].
Q7: Are there alternative pathways for this compound regeneration besides the RPE?
A7: Yes, recent studies suggest the existence of an alternative visual cycle involving Müller cells, particularly in supporting cone function [, , , , ]. This pathway becomes crucial in conditions of prolonged illumination or when the classical visual cycle is compromised [, , , ].
Q8: What are the consequences of disrupting the visual cycle?
A8: Disruptions in the visual cycle, often caused by genetic mutations or nutritional deficiencies, can lead to a shortage of this compound and accumulation of toxic retinoid byproducts [, , , , , , ]. This can lead to retinal diseases like Leber congenital amaurosis (LCA) and retinitis pigmentosa, ultimately causing vision loss [, , , , ].
Q9: Are there any therapeutic strategies targeting the visual cycle?
A9: Research is ongoing to develop therapies that can restore visual function by targeting different stages of the visual cycle [, , , ]. One approach involves gene therapy to replace mutated genes involved in this compound synthesis [, ]. Another approach explores the use of retinal analogs, such as 11-cis-6-membered ring (11-cis-6mr)-retinal, to modulate the activity of visual pigments and potentially slow down retinal degeneration [].
Q10: What are the challenges in developing visual cycle-based therapies?
A10: Developing effective therapies targeting the visual cycle faces challenges such as the complex interplay of different cell types and pathways involved in this compound synthesis and transport [, , ]. The blood-retinal barrier poses another challenge in delivering therapeutics to the retina efficiently []. Further research is needed to overcome these obstacles and develop safe and effective treatments for retinal diseases.
Haftungsausschluss und Informationen zu In-Vitro-Forschungsprodukten
Bitte beachten Sie, dass alle Artikel und Produktinformationen, die auf BenchChem präsentiert werden, ausschließlich zu Informationszwecken bestimmt sind. Die auf BenchChem zum Kauf angebotenen Produkte sind speziell für In-vitro-Studien konzipiert, die außerhalb lebender Organismen durchgeführt werden. In-vitro-Studien, abgeleitet von dem lateinischen Begriff "in Glas", beinhalten Experimente, die in kontrollierten Laborumgebungen unter Verwendung von Zellen oder Geweben durchgeführt werden. Es ist wichtig zu beachten, dass diese Produkte nicht als Arzneimittel oder Medikamente eingestuft sind und keine Zulassung der FDA für die Vorbeugung, Behandlung oder Heilung von medizinischen Zuständen, Beschwerden oder Krankheiten erhalten haben. Wir müssen betonen, dass jede Form der körperlichen Einführung dieser Produkte in Menschen oder Tiere gesetzlich strikt untersagt ist. Es ist unerlässlich, sich an diese Richtlinien zu halten, um die Einhaltung rechtlicher und ethischer Standards in Forschung und Experiment zu gewährleisten.