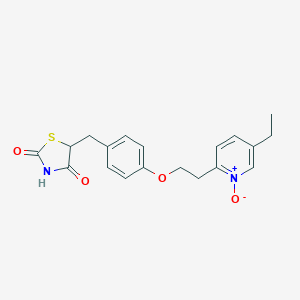
Pioglitazone N-Oxide
Übersicht
Beschreibung
Pioglitazone N-Oxide is a derivative of pioglitazone, a thiazolidinedione class drug primarily used to treat type 2 diabetes mellitus. This compound is an oxidative degradation product of pioglitazone and has been studied for its potential biological activities and chemical properties .
Wirkmechanismus
Target of Action
Pioglitazone N-Oxide primarily targets the Peroxisome Proliferator-Activated Receptor Gamma (PPARγ) . PPARγ is a ligand-activated transcription factor involved in the expression of more than 100 genes affecting numerous metabolic processes, most notably lipid and glucose homeostasis .
Mode of Action
This compound, as a selective agonist at PPARγ, interacts with its targets in target tissues for insulin action such as adipose tissue, skeletal muscle, and liver . The activation of PPARγ increases the transcription of insulin-responsive genes involved in the control of glucose and lipid production, transport, and utilization .
Biochemical Pathways
The activation of PPARγ by this compound affects several biochemical pathways. It promotes insulin sensitivity and improves the uptake of blood glucose . It also has anti-inflammatory effects, possibly by inhibiting the accumulation of neutrophils at the inflamed area, which indirectly regulates the release of nitric oxide (NO) in the affected area . Furthermore, it has been reported to attenuate oxidative stress and NLRP3 inflammasome activation .
Pharmacokinetics
The pharmacokinetics of this compound involves its absorption, distribution, metabolism, and excretion (ADME). It is metabolized primarily by the CYP2C8 enzyme . Genetic variations in the CYP2C8 and PPARG genes contribute to variability in this compound’s pharmacokinetics and pharmacodynamics .
Result of Action
The molecular and cellular effects of this compound’s action include reduced redness, scaling, and skin thickening, surpassing the effectiveness of standard drugs . It significantly inhibits the release of both myeloperoxidase (MPO) and NO from the psoriatic lesions . It also reduces the severity of psoriasis .
Action Environment
The action, efficacy, and stability of this compound can be influenced by various environmental factors. For instance, the individual health state and lifestyle measures like caloric restriction and aerobic exercise can affect the drug’s effectiveness . Further investigations into the mechanisms of actions of this compound, the potential of glitazone drugs to contribute to unmet needs in complex diseases associated with the dynamics of adaptive homeostasis, and also the routes to minimizing adverse effects in every-day patients throughout the world are imperative .
Biochemische Analyse
Biochemical Properties
Pioglitazone N-Oxide, like its parent compound Pioglitazone, is likely to interact with various enzymes, proteins, and other biomolecules. Pioglitazone is known to act as a selective agonist at peroxisome proliferator-activated receptor-gamma (PPARγ) in target tissues for insulin action such as adipose tissue, skeletal muscle, and liver . The nature of these interactions involves the activation of PPARγ, which increases the transcription of insulin-responsive genes involved in the control of glucose and lipid production .
Cellular Effects
The effects of this compound on various types of cells and cellular processes are expected to be similar to those of Pioglitazone. Pioglitazone has been shown to inhibit the proliferation and invasiveness of non-small cell lung cancer (NSCLC) cells . It also influences cell function by affecting cell signaling pathways, gene expression, and cellular metabolism . For instance, Pioglitazone has been reported to down-regulate MAPK, Myc, and Ras genes, thereby affecting the MAPK signaling pathway .
Molecular Mechanism
The molecular mechanism of action of this compound is likely to be similar to that of Pioglitazone. Pioglitazone exerts its effects at the molecular level by binding to PPARγ, which leads to the activation of insulin-responsive genes . This can result in changes in gene expression, enzyme inhibition or activation, and alterations in cellular metabolism .
Temporal Effects in Laboratory Settings
Pioglitazone has been shown to have significant effects on nitric oxide bioavailability in angiotensin II-infused rabbits .
Dosage Effects in Animal Models
Studies on Pioglitazone have shown that it significantly reduces redness, scaling, and skin thickening in a rat model of psoriasis . It also inhibits the release of myeloperoxidase and nitric oxide from psoriatic lesions .
Metabolic Pathways
Pioglitazone is known to be metabolized via two novel pathways: N-glucuronidation of the thiazolidinedione ring and methylation of the mercapto group of the thiazolidinedione ring-opened mercapto carboxylic acid .
Transport and Distribution
Pioglitazone is known to decrease mitochondrial membrane potential in isolated mitochondria and intact thymocytes .
Subcellular Localization
Pioglitazone has been shown to suppress PPARγ loss and enhance the transcriptional activity of PPARγ, inhibiting the nucleus-export of PPARγ in microglia induced by lipopolysaccharide .
Vorbereitungsmethoden
Synthetic Routes and Reaction Conditions
Pioglitazone N-Oxide can be synthesized through the oxidation of pioglitazone. One common method involves the use of hydrogen peroxide as an oxidizing agent under controlled conditions. The reaction typically takes place in an organic solvent such as methanol or acetonitrile, and the temperature is maintained at room temperature to slightly elevated temperatures .
Industrial Production Methods
While specific industrial production methods for this compound are not extensively documented, the process generally involves large-scale oxidation reactions using similar reagents and conditions as those used in laboratory synthesis. The scalability of the reaction is achieved by optimizing parameters such as reagent concentrations, reaction time, and temperature .
Analyse Chemischer Reaktionen
Types of Reactions
Pioglitazone N-Oxide undergoes various chemical reactions, including:
Oxidation: Further oxidation can lead to the formation of other oxidative degradation products.
Reduction: Reduction reactions can revert this compound back to pioglitazone.
Substitution: Nucleophilic substitution reactions can occur at the nitrogen or oxygen atoms.
Common Reagents and Conditions
Oxidation: Hydrogen peroxide, organic peroxides.
Reduction: Sodium borohydride, lithium aluminum hydride.
Substitution: Nucleophiles such as amines, thiols.
Major Products Formed
Oxidation: Further oxidized derivatives of pioglitazone.
Reduction: Pioglitazone.
Substitution: Various substituted derivatives depending on the nucleophile used.
Wissenschaftliche Forschungsanwendungen
Diabetes Management
Pioglitazone N-Oxide plays a role in enhancing insulin sensitivity and is primarily studied for its effects in type 2 diabetes mellitus. Research indicates that it may help in reducing insulin resistance and improving glycemic control through the activation of peroxisome proliferator-activated receptor gamma (PPAR-γ) pathways .
Neuroprotection
Recent studies have highlighted the neuroprotective effects of this compound. For instance, it has been shown to protect dopaminergic neurons from lipopolysaccharide (LPS)-induced damage by inhibiting inducible nitric oxide synthase (iNOS) expression and consequently reducing nitric oxide generation . This suggests potential applications in neurodegenerative diseases such as Parkinson's disease.
Biodegradation Studies
This compound has also been investigated for its biodegradation potential. A study demonstrated that microorganisms isolated from soil could oxygenate this compound, leading to the formation of various metabolites . This finding is significant for understanding the environmental impact of pharmaceutical compounds and their degradation pathways.
High-Performance Liquid Chromatography (HPLC)
The determination of this compound concentrations in biological samples has been extensively studied using HPLC techniques. These methods are crucial for pharmacokinetic studies and therapeutic monitoring, ensuring accurate dosing and efficacy assessments in clinical settings .
Case Studies
Vergleich Mit ähnlichen Verbindungen
Similar Compounds
Pioglitazone: The parent compound, primarily used for treating type 2 diabetes.
Rosiglitazone: Another thiazolidinedione with similar glucose-lowering effects.
Troglitazone: A thiazolidinedione that was withdrawn from the market due to safety concerns.
Uniqueness
Pioglitazone N-Oxide is unique due to its oxidative nature and potential additional biological activities compared to its parent compound, pioglitazone. Its oxidative stability and degradation pathways provide valuable insights into the long-term stability and efficacy of pioglitazone formulations .
Biologische Aktivität
Pioglitazone N-oxide is a metabolite of pioglitazone, a thiazolidinedione compound primarily used in the management of type 2 diabetes mellitus. This article delves into the biological activity of this compound, exploring its pharmacological effects, mechanisms of action, and potential therapeutic applications based on current research findings.
1. Overview of Pioglitazone and Its Metabolite
Chemical Structure and Properties
Pioglitazone (chemical formula: C19H20N2O3S) acts as a selective agonist for peroxisome proliferator-activated receptor gamma (PPAR-γ), which plays a critical role in glucose and lipid metabolism. This compound, formed through metabolic processes, exhibits distinct biological activities that warrant investigation.
This compound retains some pharmacological properties akin to its parent compound, primarily through the modulation of PPAR-γ. The following mechanisms have been identified:
- Insulin Sensitization : Like pioglitazone, this compound enhances insulin sensitivity in peripheral tissues, which is crucial for glucose homeostasis.
- Anti-inflammatory Effects : Studies suggest that this compound may exert anti-inflammatory effects by downregulating pro-inflammatory cytokines and modulating macrophage activation.
3.1 Pharmacological Effects
Recent studies have highlighted various biological activities of this compound:
3.2 Case Studies
Several case studies have documented the clinical implications of this compound:
- Diabetes Management : A patient cohort receiving pioglitazone exhibited improved glycemic control and reduced insulin resistance, with metabolites including this compound contributing to these effects.
4. Clinical Implications
The biological activity of this compound suggests several potential clinical applications:
- Type 2 Diabetes Therapy : As an insulin sensitizer, it may complement existing diabetes treatments.
- Anti-inflammatory Treatments : Given its anti-inflammatory properties, it could be explored for conditions like metabolic syndrome or cardiovascular diseases.
5. Future Research Directions
Further research is necessary to fully elucidate the biological activity of this compound:
- Longitudinal Studies : To assess the long-term effects and safety profile in diverse populations.
- Mechanistic Studies : To clarify the pathways through which this compound exerts its effects.
Eigenschaften
IUPAC Name |
5-[[4-[2-(5-ethyl-1-oxidopyridin-1-ium-2-yl)ethoxy]phenyl]methyl]-1,3-thiazolidine-2,4-dione | |
---|---|---|
Source | PubChem | |
URL | https://pubchem.ncbi.nlm.nih.gov | |
Description | Data deposited in or computed by PubChem | |
InChI |
InChI=1S/C19H20N2O4S/c1-2-13-3-6-15(21(24)12-13)9-10-25-16-7-4-14(5-8-16)11-17-18(22)20-19(23)26-17/h3-8,12,17H,2,9-11H2,1H3,(H,20,22,23) | |
Source | PubChem | |
URL | https://pubchem.ncbi.nlm.nih.gov | |
Description | Data deposited in or computed by PubChem | |
InChI Key |
YTKQAGFCVMHNHM-UHFFFAOYSA-N | |
Source | PubChem | |
URL | https://pubchem.ncbi.nlm.nih.gov | |
Description | Data deposited in or computed by PubChem | |
Canonical SMILES |
CCC1=C[N+](=C(C=C1)CCOC2=CC=C(C=C2)CC3C(=O)NC(=O)S3)[O-] | |
Source | PubChem | |
URL | https://pubchem.ncbi.nlm.nih.gov | |
Description | Data deposited in or computed by PubChem | |
Molecular Formula |
C19H20N2O4S | |
Source | PubChem | |
URL | https://pubchem.ncbi.nlm.nih.gov | |
Description | Data deposited in or computed by PubChem | |
DSSTOX Substance ID |
DTXSID30443587 | |
Record name | Pioglitazone N-Oxide | |
Source | EPA DSSTox | |
URL | https://comptox.epa.gov/dashboard/DTXSID30443587 | |
Description | DSSTox provides a high quality public chemistry resource for supporting improved predictive toxicology. | |
Molecular Weight |
372.4 g/mol | |
Source | PubChem | |
URL | https://pubchem.ncbi.nlm.nih.gov | |
Description | Data deposited in or computed by PubChem | |
CAS No. |
145350-09-0 | |
Record name | Pioglitazone N-Oxide | |
Source | EPA DSSTox | |
URL | https://comptox.epa.gov/dashboard/DTXSID30443587 | |
Description | DSSTox provides a high quality public chemistry resource for supporting improved predictive toxicology. | |
Synthesis routes and methods
Procedure details
Retrosynthesis Analysis
AI-Powered Synthesis Planning: Our tool employs the Template_relevance Pistachio, Template_relevance Bkms_metabolic, Template_relevance Pistachio_ringbreaker, Template_relevance Reaxys, Template_relevance Reaxys_biocatalysis model, leveraging a vast database of chemical reactions to predict feasible synthetic routes.
One-Step Synthesis Focus: Specifically designed for one-step synthesis, it provides concise and direct routes for your target compounds, streamlining the synthesis process.
Accurate Predictions: Utilizing the extensive PISTACHIO, BKMS_METABOLIC, PISTACHIO_RINGBREAKER, REAXYS, REAXYS_BIOCATALYSIS database, our tool offers high-accuracy predictions, reflecting the latest in chemical research and data.
Strategy Settings
Precursor scoring | Relevance Heuristic |
---|---|
Min. plausibility | 0.01 |
Model | Template_relevance |
Template Set | Pistachio/Bkms_metabolic/Pistachio_ringbreaker/Reaxys/Reaxys_biocatalysis |
Top-N result to add to graph | 6 |
Feasible Synthetic Routes
Q1: How is Pioglitazone N-oxide formed, and what is its significance in pharmaceutical analysis?
A: this compound is a major oxidative degradation product of Pioglitazone hydrochloride, a drug used to treat type 2 diabetes. [] This degradation product was observed during stress degradation studies designed to assess the stability of the drug substance. [] Its identification and characterization are crucial for several reasons:
Q2: Can microorganisms modify this compound?
A: Yes, research has shown that a specific strain of bacteria, Streptomyces hygroscopicus strain 02179, can both oxygenate and deoxygenate this compound. [] This microorganism can perform both N-deoxygenation, converting this compound back to Pioglitazone, and C-oxygenation, introducing an oxygen atom to different positions within the molecule. [] This finding highlights the potential for microbial metabolism to impact the fate and transformation of pharmaceutical compounds in the environment.
Haftungsausschluss und Informationen zu In-Vitro-Forschungsprodukten
Bitte beachten Sie, dass alle Artikel und Produktinformationen, die auf BenchChem präsentiert werden, ausschließlich zu Informationszwecken bestimmt sind. Die auf BenchChem zum Kauf angebotenen Produkte sind speziell für In-vitro-Studien konzipiert, die außerhalb lebender Organismen durchgeführt werden. In-vitro-Studien, abgeleitet von dem lateinischen Begriff "in Glas", beinhalten Experimente, die in kontrollierten Laborumgebungen unter Verwendung von Zellen oder Geweben durchgeführt werden. Es ist wichtig zu beachten, dass diese Produkte nicht als Arzneimittel oder Medikamente eingestuft sind und keine Zulassung der FDA für die Vorbeugung, Behandlung oder Heilung von medizinischen Zuständen, Beschwerden oder Krankheiten erhalten haben. Wir müssen betonen, dass jede Form der körperlichen Einführung dieser Produkte in Menschen oder Tiere gesetzlich strikt untersagt ist. Es ist unerlässlich, sich an diese Richtlinien zu halten, um die Einhaltung rechtlicher und ethischer Standards in Forschung und Experiment zu gewährleisten.