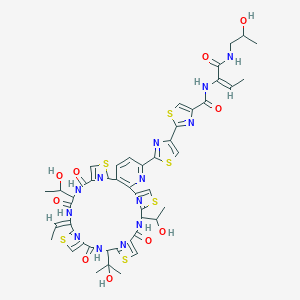
Thiocillin I
Übersicht
Beschreibung
Thiocillin I is an antibiotic originally isolated from Bacillus. It has activity against Gram-positive bacteria but not Gram-negative bacteria . It inhibits the growth of B. subtilis, B. anthracis, S. aureus, S. pyogenes, and S. pneumoniae strains in vitro .
Synthesis Analysis
The total synthesis of Thiocillin I has been described in several studies . This work defines the full structure (constitution and configuration) of the natural product . The synthesis involves a C–H activation strategy to install the trisubstituted pyridine core and thiazole groups .Chemical Reactions Analysis
The chemical reactions involved in the synthesis of Thiocillin I have been described in detail in several studies . These include the construction of the pyridine−thiazole cluster found at the core of the antibiotic .Wissenschaftliche Forschungsanwendungen
Inhibition of Bacterial Cell Growth
Thiopeptides, including Thiocillin I, inhibit bacterial cell growth by blocking ribosomal protein synthesis . The size of the macrocycle in these antibiotics is responsible for distinct modes of action .
Treatment of Drug-Resistant Pathogens
Thiopeptide antibiotics, including Thiocillin I, display antibacterial activities against drug-resistant pathogens . This makes them a potential source of new therapeutics to address the global health threat posed by drug-resistant strains of bacteria .
Wirkmechanismus
Safety and Hazards
Zukünftige Richtungen
The development of a novel Mo(VI)-oxide/picolinic acid catalyst for the cyclodehydration of cysteine peptides to form thiazoline heterocycles has enabled the total syntheses of two representative thiopeptide antibiotics: micrococcin P1 and Thiocillin I . This approach could lead to novel anti-infective agents with improved therapeutic properties .
Eigenschaften
IUPAC Name |
2-[2-[(26E)-26-ethylidene-12,29-bis(1-hydroxyethyl)-19-(2-hydroxypropan-2-yl)-14,21,28,31-tetraoxo-10,17,24,34-tetrathia-6,13,20,27,30,35,36,37,38-nonazahexacyclo[30.2.1.18,11.115,18.122,25.02,7]octatriaconta-1(35),2(7),3,5,8,11(38),15,18(37),22,25(36),32-undecaen-5-yl]-1,3-thiazol-4-yl]-N-[(E)-1-(2-hydroxypropylamino)-1-oxobut-2-en-2-yl]-1,3-thiazole-4-carboxamide | |
---|---|---|
Source | PubChem | |
URL | https://pubchem.ncbi.nlm.nih.gov | |
Description | Data deposited in or computed by PubChem | |
InChI |
InChI=1S/C48H49N13O10S6/c1-8-23(36(65)49-12-19(3)62)51-37(66)27-15-74-45(56-27)31-18-75-44(58-31)25-11-10-22-34(50-25)26-13-76-46(53-26)33(21(5)64)60-39(68)29-17-77-47(57-29)35(48(6,7)71)61-40(69)30-16-73-43(55-30)24(9-2)52-41(70)32(20(4)63)59-38(67)28-14-72-42(22)54-28/h8-11,13-21,32-33,35,62-64,71H,12H2,1-7H3,(H,49,65)(H,51,66)(H,52,70)(H,59,67)(H,60,68)(H,61,69)/b23-8+,24-9+ | |
Source | PubChem | |
URL | https://pubchem.ncbi.nlm.nih.gov | |
Description | Data deposited in or computed by PubChem | |
InChI Key |
FEORQDDAQBRWPT-NBBXXHIDSA-N | |
Source | PubChem | |
URL | https://pubchem.ncbi.nlm.nih.gov | |
Description | Data deposited in or computed by PubChem | |
Canonical SMILES |
CC=C1C2=NC(=CS2)C(=O)NC(C3=NC(=CS3)C(=O)NC(C4=NC(=CS4)C5=C(C=CC(=N5)C6=NC(=CS6)C7=NC(=CS7)C(=O)NC(=CC)C(=O)NCC(C)O)C8=NC(=CS8)C(=O)NC(C(=O)N1)C(C)O)C(C)O)C(C)(C)O | |
Source | PubChem | |
URL | https://pubchem.ncbi.nlm.nih.gov | |
Description | Data deposited in or computed by PubChem | |
Isomeric SMILES |
C/C=C/1\C2=NC(=CS2)C(=O)NC(C3=NC(=CS3)C(=O)NC(C4=NC(=CS4)C5=C(C=CC(=N5)C6=NC(=CS6)C7=NC(=CS7)C(=O)N/C(=C/C)/C(=O)NCC(C)O)C8=NC(=CS8)C(=O)NC(C(=O)N1)C(C)O)C(C)O)C(C)(C)O | |
Source | PubChem | |
URL | https://pubchem.ncbi.nlm.nih.gov | |
Description | Data deposited in or computed by PubChem | |
Molecular Formula |
C48H49N13O10S6 | |
Source | PubChem | |
URL | https://pubchem.ncbi.nlm.nih.gov | |
Description | Data deposited in or computed by PubChem | |
Molecular Weight |
1160.4 g/mol | |
Source | PubChem | |
URL | https://pubchem.ncbi.nlm.nih.gov | |
Description | Data deposited in or computed by PubChem | |
Product Name |
Thiocillin I | |
CAS RN |
59979-01-0 | |
Record name | Thiocillin I | |
Source | ChemIDplus | |
URL | https://pubchem.ncbi.nlm.nih.gov/substance/?source=chemidplus&sourceid=0059979010 | |
Description | ChemIDplus is a free, web search system that provides access to the structure and nomenclature authority files used for the identification of chemical substances cited in National Library of Medicine (NLM) databases, including the TOXNET system. | |
Retrosynthesis Analysis
AI-Powered Synthesis Planning: Our tool employs the Template_relevance Pistachio, Template_relevance Bkms_metabolic, Template_relevance Pistachio_ringbreaker, Template_relevance Reaxys, Template_relevance Reaxys_biocatalysis model, leveraging a vast database of chemical reactions to predict feasible synthetic routes.
One-Step Synthesis Focus: Specifically designed for one-step synthesis, it provides concise and direct routes for your target compounds, streamlining the synthesis process.
Accurate Predictions: Utilizing the extensive PISTACHIO, BKMS_METABOLIC, PISTACHIO_RINGBREAKER, REAXYS, REAXYS_BIOCATALYSIS database, our tool offers high-accuracy predictions, reflecting the latest in chemical research and data.
Strategy Settings
Precursor scoring | Relevance Heuristic |
---|---|
Min. plausibility | 0.01 |
Model | Template_relevance |
Template Set | Pistachio/Bkms_metabolic/Pistachio_ringbreaker/Reaxys/Reaxys_biocatalysis |
Top-N result to add to graph | 6 |
Feasible Synthetic Routes
Q & A
Q1: What is thiocillin I and how does it differ from other related antibiotics?
A1: Thiocillin I is a thiopeptide antibiotic, first isolated from Bacillus cereus G-15 and Bacillus badius AR-91. [, ] These sulfur-rich, ribosomally synthesized and post-translationally modified peptides are known for their potent activity against Gram-positive bacteria. [, ] Thiocillin I shares structural similarities with micrococcin P1, another thiopeptide antibiotic, but they differ in specific structural units. [, ] These differences are evident in their chromatographic behavior and potentially contribute to variations in their biological activity. [, ]
Q2: What is the mechanism of action of thiocillin I?
A2: While the exact mechanism is still being elucidated, thiopeptides, like thiocillin I, are known to inhibit bacterial ribosomes. [] This suggests that they interfere with bacterial protein synthesis, ultimately leading to bacterial cell death.
Q3: Have there been any successful total syntheses of thiocillin I?
A4: Yes, the total synthesis of thiocillin I has been achieved. [, , ] Notably, a novel molybdenum catalyst played a crucial role in enabling the synthesis. [] This breakthrough facilitates further research into the structure-activity relationship, mode of action, and potential therapeutic applications of thiocillin I.
Q4: How does thiocillin I influence interspecies interactions within a biofilm?
A5: Research using a four-species model biofilm demonstrated that thiocillin I, produced by Bacillus cereus, plays a significant role in shaping the biofilm's composition. [] It exerts a negative interaction on other species, influencing their distribution and abundance within the biofilm. This highlights the ecological importance of thiocillin I in complex microbial communities.
Q5: What are the future directions for research on thiocillin I?
A5: Future research could focus on several aspects of thiocillin I, including:
Haftungsausschluss und Informationen zu In-Vitro-Forschungsprodukten
Bitte beachten Sie, dass alle Artikel und Produktinformationen, die auf BenchChem präsentiert werden, ausschließlich zu Informationszwecken bestimmt sind. Die auf BenchChem zum Kauf angebotenen Produkte sind speziell für In-vitro-Studien konzipiert, die außerhalb lebender Organismen durchgeführt werden. In-vitro-Studien, abgeleitet von dem lateinischen Begriff "in Glas", beinhalten Experimente, die in kontrollierten Laborumgebungen unter Verwendung von Zellen oder Geweben durchgeführt werden. Es ist wichtig zu beachten, dass diese Produkte nicht als Arzneimittel oder Medikamente eingestuft sind und keine Zulassung der FDA für die Vorbeugung, Behandlung oder Heilung von medizinischen Zuständen, Beschwerden oder Krankheiten erhalten haben. Wir müssen betonen, dass jede Form der körperlichen Einführung dieser Produkte in Menschen oder Tiere gesetzlich strikt untersagt ist. Es ist unerlässlich, sich an diese Richtlinien zu halten, um die Einhaltung rechtlicher und ethischer Standards in Forschung und Experiment zu gewährleisten.