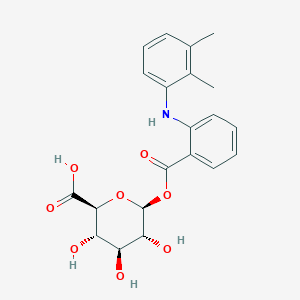
Mefenaminsäureglucuronid
Übersicht
Beschreibung
Mefenamic acid glucuronide is a metabolite of mefenamic acid, a non-steroidal anti-inflammatory drug (NSAID) commonly used for its analgesic, anti-inflammatory, and antipyretic properties . This compound is formed through the process of glucuronidation, where mefenamic acid is conjugated with glucuronic acid, enhancing its solubility and facilitating its excretion from the body .
Wissenschaftliche Forschungsanwendungen
Mefenamic acid glucuronide has several applications in scientific research:
Pharmacokinetics: Studying the metabolism and excretion of mefenamic acid in the body.
Toxicology: Investigating the potential toxic effects of acyl glucuronides, which can form covalent adducts with proteins.
Drug Development: Understanding the role of glucuronidation in drug metabolism and designing drugs with improved pharmacokinetic profiles.
Biomarker Discovery: Using glucuronide metabolites as biomarkers for drug exposure and efficacy.
Wirkmechanismus
Target of Action
Mefenamic acid, the parent compound of mefenamic acid glucuronide, primarily targets the prostaglandin synthetase receptors COX-1 and COX-2 . These receptors play a significant role as major mediators of inflammation and prostanoid signaling in activity-dependent plasticity .
Mode of Action
Mefenamic acid interacts with its targets by binding to the prostaglandin synthetase receptors COX-1 and COX-2, thereby inhibiting the action of prostaglandin synthetase . This inhibition results in a temporary reduction in the symptoms of pain .
Biochemical Pathways
The primary biochemical pathway affected by mefenamic acid involves the synthesis of prostaglandins. Mefenamic acid is a potent inhibitor of prostaglandin synthesis . Prostaglandins are mediators of inflammation, and their synthesis is inhibited by mefenamic acid, which may contribute to its analgesic, anti-inflammatory, and antipyretic properties .
Pharmacokinetics
Mefenamic acid is rapidly absorbed after oral administration. Following a single 1-gram oral dose, mean peak plasma levels ranging from 10-20 mcg/mL have been reported . Peak plasma levels are attained in 2 to 4 hours and the elimination half-life approximates 2 hours . Approximately fifty-two percent of a mefenamic acid dose is excreted into the urine primarily as glucuronides of mefenamic acid .
Result of Action
The molecular and cellular effects of mefenamic acid’s action primarily involve the reduction of inflammation and pain. By inhibiting prostaglandin synthesis, mefenamic acid may decrease prostaglandins in peripheral tissues, reducing inflammation and pain .
Action Environment
The action of mefenamic acid glucuronide can be influenced by environmental factors such as pH. For instance, the degradation of mefenamic acid glucuronide was found to be accelerated under alkaline conditions . Furthermore, mefenamic acid glucuronide, although extremely stable in buffer at physiological pH, was found to bind irreversibly to human serum albumin in vitro .
Biochemische Analyse
Biochemical Properties
Mefenamic Acid Glucuronide interacts with various enzymes and proteins in the body. It is primarily excreted into the urine as glucuronides of Mefenamic Acid . The interactions of this compound with other biomolecules are complex and involve multiple biochemical reactions.
Cellular Effects
Mefenamic Acid Glucuronide influences cell function by modulating the inflammatory response. It impacts cell signaling pathways, gene expression, and cellular metabolism . Its effects are particularly notable in cells involved in the immune response.
Molecular Mechanism
The mechanism of action of Mefenamic Acid Glucuronide involves binding interactions with biomolecules, enzyme inhibition or activation, and changes in gene expression . These interactions allow it to exert its effects at the molecular level.
Temporal Effects in Laboratory Settings
In laboratory settings, the effects of Mefenamic Acid Glucuronide change over time. It has been observed that this compound has a certain degree of stability, but can undergo degradation under specific conditions . Long-term effects on cellular function have been observed in both in vitro and in vivo studies.
Dosage Effects in Animal Models
The effects of Mefenamic Acid Glucuronide vary with different dosages in animal models. At certain thresholds, it exhibits therapeutic effects, while at high doses, it may cause toxic or adverse effects .
Metabolic Pathways
Mefenamic Acid Glucuronide is involved in several metabolic pathways. It interacts with various enzymes and cofactors, and can affect metabolic flux or metabolite levels .
Transport and Distribution
Mefenamic Acid Glucuronide is transported and distributed within cells and tissues. It interacts with various transporters and binding proteins, which can affect its localization or accumulation .
Subcellular Localization
The subcellular localization of Mefenamic Acid Glucuronide can affect its activity or function. It may be directed to specific compartments or organelles based on targeting signals or post-translational modifications .
Vorbereitungsmethoden
Synthetic Routes and Reaction Conditions: Mefenamic acid glucuronide is synthesized through the enzymatic process of glucuronidation. This involves the enzyme UDP-glucuronosyltransferase (UGT), which catalyzes the transfer of glucuronic acid from uridine diphosphate glucuronic acid to mefenamic acid . The reaction typically occurs in the liver, where UGT enzymes are abundant.
Industrial Production Methods: Industrial production of mefenamic acid glucuronide involves the use of recombinant UGT enzymes in bioreactors. The process is optimized for high yield and purity, often involving steps such as enzyme immobilization, controlled reaction conditions (pH, temperature), and downstream purification techniques like chromatography .
Analyse Chemischer Reaktionen
Types of Reactions: Mefenamic acid glucuronide primarily undergoes hydrolysis, where the glucuronic acid moiety is cleaved off, reverting it back to mefenamic acid . It can also participate in transacylation reactions, where the acyl group is transferred to other nucleophiles .
Common Reagents and Conditions:
Hydrolysis: Acidic or basic conditions can catalyze the hydrolysis of mefenamic acid glucuronide.
Transacylation: This reaction can occur in the presence of nucleophiles like amino acids or proteins under physiological conditions.
Major Products:
Hydrolysis: Mefenamic acid and glucuronic acid.
Transacylation: Various acylated products depending on the nucleophile involved.
Vergleich Mit ähnlichen Verbindungen
Ibuprofen Glucuronide: Another NSAID glucuronide with similar pharmacokinetic properties.
Naproxen Glucuronide: Exhibits similar metabolic pathways and therapeutic effects.
Uniqueness: Mefenamic acid glucuronide is unique due to its specific parent compound, mefenamic acid, which belongs to the fenamate class of NSAIDs. This class is known for its distinct chemical structure and specific therapeutic applications .
Biologische Aktivität
Mefenamic acid glucuronide (MFA-G) is a metabolite of mefenamic acid, a nonsteroidal anti-inflammatory drug (NSAID) widely used for its analgesic and anti-inflammatory properties. Understanding the biological activity of MFA-G is crucial due to its implications in pharmacology, toxicology, and drug metabolism. This article synthesizes findings from various studies to provide a comprehensive overview of the biological activity of MFA-G, including its stability, reactivity, and potential clinical implications.
Overview of Mefenamic Acid and Its Metabolism
Mefenamic acid is primarily metabolized in the liver through phase I and phase II metabolic pathways. The phase II metabolism involves conjugation with glucuronic acid via the enzyme UDP-glucuronosyltransferase (UGT), resulting in the formation of several glucuronide metabolites, including MFA-G. The pharmacokinetics of mefenamic acid indicate that over 90% is bound to plasma proteins, and it has a volume of distribution of approximately 1.06 L/kg in healthy adults .
Stability of Mefenamic Acid Glucuronide
Studies have demonstrated that MFA-G exhibits significant stability under physiological conditions. The half-life of MFA-G at 37°C and pH 7.4 is approximately 16.5 hours, which is notably longer than many other acyl glucuronides . However, its stability decreases under alkaline conditions, where the half-life drops to about 5 hours at pH 8.0. This stability profile suggests that MFA-G may persist longer in biological systems compared to other metabolites.
Reactivity with Proteins
MFA-G is characterized by its ability to bind irreversibly to proteins, which raises concerns regarding potential toxicity. In vitro studies have shown that MFA-G can covalently modify human serum albumin and other cellular proteins . This irreversible binding occurs through a transacylation reaction where nucleophilic sites on proteins react with the carbonyl group of the acyl glucuronide, leading to the formation of drug-protein adducts. Such adducts may contribute to idiosyncratic drug reactions and nephrotoxicity associated with mefenamic acid use .
Biological Activity and Toxicological Implications
The biological activity of MFA-G extends beyond mere binding to proteins; it also involves interactions with various biomolecules. For instance, research indicates that MFA-G can transacylate amino acids and thiol-containing compounds like glutathione (GSH) and N-acetylcysteine (NAC) . These interactions highlight a potential mechanism for toxicity, as they may lead to oxidative stress or other adverse effects.
Case Studies
- Nephrotoxicity : Several case reports have linked mefenamic acid use to acute renal failure and tubulointerstitial nephritis. One proposed mechanism involves the formation of immune responses against drug-protein conjugates formed by MFA-G binding .
- Toxicity Profile : A study examining patients treated with mefenamic acid revealed elevated levels of reactive metabolites in serum samples, correlating with adverse reactions such as gastrointestinal disturbances and renal impairment .
Summary of Key Findings
Parameter | Value |
---|---|
Half-Life at pH 7.4 | 16.5 hours |
Half-Life at pH 8.0 | 5 hours |
Protein Binding | Irreversible binding observed |
Toxicological Concerns | Nephrotoxicity and potential for idiosyncratic reactions |
Eigenschaften
IUPAC Name |
(2S,3S,4S,5R,6S)-6-[2-(2,3-dimethylanilino)benzoyl]oxy-3,4,5-trihydroxyoxane-2-carboxylic acid | |
---|---|---|
Source | PubChem | |
URL | https://pubchem.ncbi.nlm.nih.gov | |
Description | Data deposited in or computed by PubChem | |
InChI |
InChI=1S/C21H23NO8/c1-10-6-5-9-13(11(10)2)22-14-8-4-3-7-12(14)20(28)30-21-17(25)15(23)16(24)18(29-21)19(26)27/h3-9,15-18,21-25H,1-2H3,(H,26,27)/t15-,16-,17+,18-,21-/m0/s1 | |
Source | PubChem | |
URL | https://pubchem.ncbi.nlm.nih.gov | |
Description | Data deposited in or computed by PubChem | |
InChI Key |
DAHIGOGKMFBIOR-CURYNPBISA-N | |
Source | PubChem | |
URL | https://pubchem.ncbi.nlm.nih.gov | |
Description | Data deposited in or computed by PubChem | |
Canonical SMILES |
CC1=C(C(=CC=C1)NC2=CC=CC=C2C(=O)OC3C(C(C(C(O3)C(=O)O)O)O)O)C | |
Source | PubChem | |
URL | https://pubchem.ncbi.nlm.nih.gov | |
Description | Data deposited in or computed by PubChem | |
Isomeric SMILES |
CC1=C(C(=CC=C1)NC2=CC=CC=C2C(=O)O[C@H]3[C@@H]([C@H]([C@@H]([C@H](O3)C(=O)O)O)O)O)C | |
Source | PubChem | |
URL | https://pubchem.ncbi.nlm.nih.gov | |
Description | Data deposited in or computed by PubChem | |
Molecular Formula |
C21H23NO8 | |
Source | PubChem | |
URL | https://pubchem.ncbi.nlm.nih.gov | |
Description | Data deposited in or computed by PubChem | |
DSSTOX Substance ID |
DTXSID90907958 | |
Record name | 1-O-[2-(2,3-Dimethylanilino)benzoyl]hexopyranuronic acid | |
Source | EPA DSSTox | |
URL | https://comptox.epa.gov/dashboard/DTXSID90907958 | |
Description | DSSTox provides a high quality public chemistry resource for supporting improved predictive toxicology. | |
Molecular Weight |
417.4 g/mol | |
Source | PubChem | |
URL | https://pubchem.ncbi.nlm.nih.gov | |
Description | Data deposited in or computed by PubChem | |
CAS No. |
102623-18-7 | |
Record name | β-D-Glucopyranuronic acid, 1-[2-[(2,3-dimethylphenyl)amino]benzoate] | |
Source | CAS Common Chemistry | |
URL | https://commonchemistry.cas.org/detail?cas_rn=102623-18-7 | |
Description | CAS Common Chemistry is an open community resource for accessing chemical information. Nearly 500,000 chemical substances from CAS REGISTRY cover areas of community interest, including common and frequently regulated chemicals, and those relevant to high school and undergraduate chemistry classes. This chemical information, curated by our expert scientists, is provided in alignment with our mission as a division of the American Chemical Society. | |
Explanation | The data from CAS Common Chemistry is provided under a CC-BY-NC 4.0 license, unless otherwise stated. | |
Record name | 1-O-(2-(2,3-Dimethylphenyl)aminobenzoyl)glucopyranuronic acid | |
Source | ChemIDplus | |
URL | https://pubchem.ncbi.nlm.nih.gov/substance/?source=chemidplus&sourceid=0102623187 | |
Description | ChemIDplus is a free, web search system that provides access to the structure and nomenclature authority files used for the identification of chemical substances cited in National Library of Medicine (NLM) databases, including the TOXNET system. | |
Record name | 1-O-[2-(2,3-Dimethylanilino)benzoyl]hexopyranuronic acid | |
Source | EPA DSSTox | |
URL | https://comptox.epa.gov/dashboard/DTXSID90907958 | |
Description | DSSTox provides a high quality public chemistry resource for supporting improved predictive toxicology. | |
Record name | MEFENAMIC ACID GLUCURONIDE | |
Source | FDA Global Substance Registration System (GSRS) | |
URL | https://gsrs.ncats.nih.gov/ginas/app/beta/substances/OX5H10G1RG | |
Description | The FDA Global Substance Registration System (GSRS) enables the efficient and accurate exchange of information on what substances are in regulated products. Instead of relying on names, which vary across regulatory domains, countries, and regions, the GSRS knowledge base makes it possible for substances to be defined by standardized, scientific descriptions. | |
Explanation | Unless otherwise noted, the contents of the FDA website (www.fda.gov), both text and graphics, are not copyrighted. They are in the public domain and may be republished, reprinted and otherwise used freely by anyone without the need to obtain permission from FDA. Credit to the U.S. Food and Drug Administration as the source is appreciated but not required. | |
Retrosynthesis Analysis
AI-Powered Synthesis Planning: Our tool employs the Template_relevance Pistachio, Template_relevance Bkms_metabolic, Template_relevance Pistachio_ringbreaker, Template_relevance Reaxys, Template_relevance Reaxys_biocatalysis model, leveraging a vast database of chemical reactions to predict feasible synthetic routes.
One-Step Synthesis Focus: Specifically designed for one-step synthesis, it provides concise and direct routes for your target compounds, streamlining the synthesis process.
Accurate Predictions: Utilizing the extensive PISTACHIO, BKMS_METABOLIC, PISTACHIO_RINGBREAKER, REAXYS, REAXYS_BIOCATALYSIS database, our tool offers high-accuracy predictions, reflecting the latest in chemical research and data.
Strategy Settings
Precursor scoring | Relevance Heuristic |
---|---|
Min. plausibility | 0.01 |
Model | Template_relevance |
Template Set | Pistachio/Bkms_metabolic/Pistachio_ringbreaker/Reaxys/Reaxys_biocatalysis |
Top-N result to add to graph | 6 |
Feasible Synthetic Routes
Q1: How does mefenamic acid glucuronide interact with proteins, and what are the implications of this interaction?
A1: Mefenamic acid glucuronide, despite its relative stability at physiological pH [], has been shown to bind irreversibly to proteins. This binding was observed both in vitro with human serum albumin and in cultured cells expressing human UDP-glucuronosyltransferase UGT1*02 []. This irreversible binding to cellular proteins, particularly in renal tissues, is suggested as a potential mechanism for mefenamic acid-induced nephrotoxicity. The formation of mefenamic acid-protein adducts could trigger an immune response, leading to inflammation and tissue damage in the kidneys [].
Haftungsausschluss und Informationen zu In-Vitro-Forschungsprodukten
Bitte beachten Sie, dass alle Artikel und Produktinformationen, die auf BenchChem präsentiert werden, ausschließlich zu Informationszwecken bestimmt sind. Die auf BenchChem zum Kauf angebotenen Produkte sind speziell für In-vitro-Studien konzipiert, die außerhalb lebender Organismen durchgeführt werden. In-vitro-Studien, abgeleitet von dem lateinischen Begriff "in Glas", beinhalten Experimente, die in kontrollierten Laborumgebungen unter Verwendung von Zellen oder Geweben durchgeführt werden. Es ist wichtig zu beachten, dass diese Produkte nicht als Arzneimittel oder Medikamente eingestuft sind und keine Zulassung der FDA für die Vorbeugung, Behandlung oder Heilung von medizinischen Zuständen, Beschwerden oder Krankheiten erhalten haben. Wir müssen betonen, dass jede Form der körperlichen Einführung dieser Produkte in Menschen oder Tiere gesetzlich strikt untersagt ist. Es ist unerlässlich, sich an diese Richtlinien zu halten, um die Einhaltung rechtlicher und ethischer Standards in Forschung und Experiment zu gewährleisten.