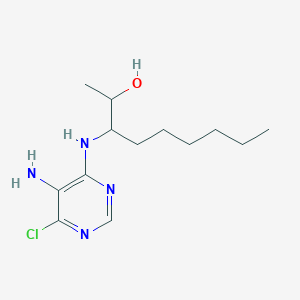
rac erythro-3-(5-Amino-6-chloropyrimidin-4-ylamino)-nonan-2-ol
Übersicht
Beschreibung
rac erythro-3-(5-Amino-6-chloropyrimidin-4-ylamino)-nonan-2-ol (CAS: 75166-65-3) is a synthetic amino alcohol derivative featuring a pyrimidine core substituted with chlorine and amino groups, linked to a nonan-2-ol chain. Its structure combines a heterocyclic aromatic system (5-amino-6-chloropyrimidine) with a branched aliphatic alcohol, imparting unique physicochemical properties. This compound has been referenced in product catalogs for biochemical research, particularly in studies involving amino acid analogs and enzyme interactions .
Vorbereitungsmethoden
Synthesewege und Reaktionsbedingungen
Cevimelinhydrochlorid wird durch eine Reihe von chemischen Reaktionen synthetisiert, die von cis-2-Methylspiro(1,3-oxathiolan-5,3’)quiniclidin ausgehen. Das Verfahren beinhaltet die Behandlung eines cis/trans-Isomerengemischs mit einer organischen Sulfonsäure, um ein weniger lösliches Säureadditionssalz zu erzeugen, das an cis-Isomeren angereichert ist. Umkristallisation oder Aufschließen mit verschiedenen organischen Lösungsmitteln ermöglicht die Anreicherung des cis-Isomeren durch Filtration. Dieses cis-Isomer wird dann durch Behandlung mit einer Base, gefolgt von der Bildung eines Salzes mit Salzsäure, in das Hydrochloridsalz umgewandelt .
Industrielle Produktionsverfahren
Die industrielle Produktion von Cevimelinhydrochlorid beinhaltet die Herstellung und Reinigung des cis-Isomeren von 2-Methylspiro(1,3-oxathiolan-5,3’)quiniclidin. Dieser Prozess stellt sicher, dass ein stabiles und hochwertiges Produkt hergestellt wird, das für den pharmazeutischen Einsatz geeignet ist .
Chemische Reaktionsanalyse
Arten von Reaktionen
Cevimelinhydrochlorid unterliegt aufgrund des Vorhandenseins seiner funktionellen Gruppen hauptsächlich Substitutionsreaktionen. Es kann auch unter bestimmten Bedingungen an Oxidations- und Reduktionsreaktionen teilnehmen.
Häufige Reagenzien und Bedingungen
Substitutionsreaktionen: Diese Reaktionen beinhalten typischerweise Nukleophile wie Hydroxidionen oder Amine.
Oxidationsreaktionen: Häufige Oxidationsmittel umfassen Kaliumpermanganat und Wasserstoffperoxid.
Reduktionsreaktionen: Reduktionsmittel wie Lithiumaluminiumhydrid oder Natriumborhydrid werden verwendet.
Hauptprodukte, die gebildet werden
Die Hauptprodukte, die aus diesen Reaktionen entstehen, hängen von den jeweiligen Reagenzien und Bedingungen ab. So können Substitutionsreaktionen verschiedene substituierte Derivate von Cevimelin ergeben, während Oxidations- und Reduktionsreaktionen zur Bildung verschiedener oxidierter oder reduzierter Formen der Verbindung führen können.
Analyse Chemischer Reaktionen
Types of Reactions
Cevimeline hydrochloride primarily undergoes substitution reactions due to the presence of its functional groups. It can also participate in oxidation and reduction reactions under specific conditions.
Common Reagents and Conditions
Substitution Reactions: These reactions typically involve nucleophiles such as hydroxide ions or amines.
Oxidation Reactions: Common oxidizing agents include potassium permanganate and hydrogen peroxide.
Reduction Reactions: Reducing agents such as lithium aluminum hydride or sodium borohydride are used.
Major Products Formed
The major products formed from these reactions depend on the specific reagents and conditions used. For example, substitution reactions may yield various substituted derivatives of cevimeline, while oxidation and reduction reactions can lead to the formation of different oxidized or reduced forms of the compound.
Wissenschaftliche Forschungsanwendungen
Medicinal Chemistry
Rac erythro-3-(5-amino-6-chloropyrimidin-4-ylamino)-nonan-2-ol has shown promise in the development of novel pharmaceuticals. Its structural features suggest potential activity against various biological targets, particularly in the realm of cancer therapeutics and anti-infective agents.
Case Study: Anticancer Activity
Research has indicated that compounds similar to rac erythro derivatives exhibit cytotoxic effects on cancer cell lines. For instance, studies have demonstrated that modifications in the pyrimidine ring can enhance the selectivity and potency of these compounds against specific tumor types.
Antiviral Research
The compound's unique structure may also contribute to antiviral activity. Preliminary studies suggest that it could inhibit viral replication through mechanisms that involve interference with viral polymerases or proteases.
Case Study: Inhibition of Viral Replication
A study evaluated the efficacy of rac erythro derivatives against a range of viruses, including influenza and HIV. The results indicated significant reductions in viral load in treated cell cultures compared to controls, highlighting its potential as a lead compound for antiviral drug development.
Biochemical Research
In biochemical assays, rac erythro compounds have been utilized as probes to study enzyme activities and interactions within metabolic pathways. Their ability to mimic natural substrates makes them valuable tools for elucidating biochemical mechanisms.
Case Study: Enzyme Inhibition Studies
Research involving rac erythro derivatives has shown their effectiveness in inhibiting specific enzymes involved in metabolic disorders. For example, inhibition assays demonstrated that these compounds could modulate enzyme activity linked to glucose metabolism, providing insights into diabetes treatment strategies.
Wirkmechanismus
Cevimeline hydrochloride exerts its effects by binding to and activating muscarinic acetylcholine receptors M1 and M3. The activation of M1 receptors, which are common in secretory glands, results in increased secretion from these glands. The M3 receptors, found on smooth muscles and various glands, stimulate secretion in salivary glands and cause smooth muscle contraction .
Vergleich Mit ähnlichen Verbindungen
Below is a detailed comparison:
Table 1: Structural and Functional Comparison
Key Observations :
Structural Complexity: The target compound’s pyrimidine-aliphatic hybrid structure distinguishes it from simpler heterocycles like pyridazinone or oxazolidinone derivatives. This complexity may enhance binding specificity in biochemical assays but complicates synthesis and stability .
Availability and Cost: Discontinuation of the compound (vs. commercially available analogs like 5-amino-4-chloro-2-phenylpyridazin-3(2H)-one) suggests challenges in scalability or niche demand .
Research Findings :
- Hydrogen Bonding Patterns: The amino and hydroxyl groups in this compound likely form intramolecular hydrogen bonds, stabilizing its conformation. This contrasts with 3-Amino-2-Oxazolidinone, where hydrogen bonding is intermolecular and critical for crystallinity .
Biologische Aktivität
Rac erythro-3-(5-Amino-6-chloropyrimidin-4-ylamino)-nonan-2-ol, also known as AC-93253, is a heterocyclic organic compound with potential therapeutic applications. Its molecular formula is C13H23ClN4O, and it has garnered interest for its biological activity, particularly in cancer research and other therapeutic areas.
- Molecular Weight : 286.80 g/mol
- CAS Number : 75166-65-3
- IUPAC Name : 3-[(5-amino-6-chloropyrimidin-4-yl)amino]nonan-2-ol
Biological Activity Overview
The biological activity of this compound has been investigated in various studies, focusing primarily on its anticancer properties and interactions with biological targets.
The compound is believed to exert its effects by interacting with specific enzymes or receptors, potentially modulating their activity. This interaction may lead to various biological effects, including apoptosis in cancer cells.
Anticancer Activity
-
Cell Line Studies : In vitro studies have shown that rac erythro can induce cytotoxic effects on various cancer cell lines. For instance, similar compounds have demonstrated IC50 values ranging from 2.32 to 91.00 µg/mL against MCF-7 and HepG2 cell lines, indicating significant anticancer potential .
Compound IC50 (MCF-7) IC50 (HepG2) 4e 5.36 µg/mL 3.13 µg/mL 4i 2.32 µg/mL 6.51 µg/mL 5-FU 6.80 µg/mL 8.40 µg/mL - Mechanistic Studies : Flow cytometric analysis has indicated that treatment with compounds similar to rac erythro leads to cell cycle arrest at the S and G2/M phases in cancer cells, along with increased levels of pro-apoptotic markers such as Bax and caspase 9 .
Pharmacokinetics
Pharmacokinetic studies suggest that rac erythro can effectively cross the blood-brain barrier, enhancing its potential for neurological applications in addition to its anticancer properties .
Case Studies
- In Vivo Efficacy : In a sarcoma-bearing mouse model, the pharmacokinetics of rac erythro were assessed using radiolabeling techniques, showing promising targeting ability towards solid tumors .
- Combination Therapies : Recent studies have explored the combination of rac erythro with other chemotherapeutic agents, revealing synergistic effects that enhance overall efficacy against resistant cancer cell lines.
Q & A
Basic Research Questions
Q. What are the primary synthetic routes for rac erythro-3-(5-Amino-6-chloropyrimidin-4-ylamino)-nonan-2-ol, and how are stereochemical outcomes controlled?
- The compound’s synthesis involves coupling pyrimidine derivatives with nonanol-based intermediates. Stereochemical control (erythro configuration) is achieved via chiral catalysts or diastereomeric crystallization. Patents (e.g., EP 4 374 877 A2) highlight analogous pyrimidine coupling strategies using nucleophilic substitution or palladium-catalyzed cross-coupling . Computational reaction path searches (e.g., ICReDD’s quantum chemical methods) can optimize stereoselectivity by predicting transition states .
Q. Which analytical techniques are critical for characterizing this compound’s purity and structure?
- NMR spectroscopy resolves stereochemistry and confirms substitution patterns on the pyrimidine ring. HPLC-MS identifies impurities (e.g., chlorinated byproducts) and quantifies enantiomeric excess. X-ray crystallography is definitive for absolute configuration determination. Pharmacopeial standards (e.g., PHARMACOPEIAL FORUM) emphasize rigorous validation of analytical methods for structurally complex amines .
Q. How can researchers address solubility challenges during in vitro assays for this compound?
- Use co-solvents like DMSO (≤1% v/v) or cyclodextrin-based encapsulation to enhance aqueous solubility. Pre-formulation studies should assess pH-dependent stability (e.g., via UV-Vis spectroscopy) to avoid degradation in buffer systems .
Advanced Research Questions
Q. What experimental design strategies optimize reaction yields while minimizing diastereomer formation?
- Factorial design (e.g., 2^k designs) systematically evaluates variables like temperature, solvent polarity, and catalyst loading. For example, higher temperatures may accelerate coupling but increase racemization. ICReDD’s feedback loop integrates computational predictions (e.g., activation energies for stereochemical pathways) with empirical data to narrow optimal conditions .
Q. How can computational modeling resolve contradictions in stereochemical assignments from NMR data?
- Conflicting NOE (Nuclear Overhauser Effect) signals or coupling constants may arise from dynamic equilibria. Density Functional Theory (DFT) simulations predict NMR chemical shifts for proposed stereoisomers, which are compared to experimental data. This approach validated the erythro configuration in related pyrimidine derivatives .
Q. What methodologies separate trace impurities or diastereomers in bulk synthesis?
- Preparative HPLC with chiral stationary phases (e.g., cellulose-based columns) achieves baseline separation. Membrane technologies (e.g., nanofiltration) selectively remove low-molecular-weight impurities while retaining the target compound. Recent advances in CRDC-classified separation engineering highlight scalability for chiral amines .
Q. How do researchers validate the compound’s stability under long-term storage conditions?
- Forced degradation studies (ICH guidelines) expose the compound to heat (40–60°C), humidity (75% RH), and light (UV-A/B). LC-MS monitors degradation products, such as dechlorinated pyrimidines or oxidized nonanol chains. Stability-indicating methods must comply with pharmacopeial standards for impurity profiling .
Q. Data Analysis and Contradiction Resolution
Q. How to interpret conflicting bioactivity data across different assay platforms?
- Discrepancies may arise from assay-specific solvent effects or protein binding. Normalize data using standardized positive controls (e.g., known kinase inhibitors for enzyme assays) and apply multivariate analysis to isolate confounding variables. ICReDD’s data-circulation framework reconciles computational predictions (e.g., binding affinity) with experimental IC50 values .
Q. What statistical approaches quantify reproducibility in synthetic batches?
- Principal Component Analysis (PCA) identifies batch-to-batch variability sources (e.g., catalyst lot differences). Control charts (e.g., X-bar charts) monitor critical quality attributes (e.g., enantiomeric purity) against predefined tolerances. CRDC guidelines emphasize process simulation to preempt deviations .
Q. Methodological Tables
Table 1. Key Analytical Parameters for This compound
Parameter | Method | Acceptance Criteria | Reference |
---|---|---|---|
Enantiomeric purity | Chiral HPLC | ≥98% erythro isomer | |
Residual solvent (DMSO) | GC-FID | ≤500 ppm | |
Degradation products | LC-MS/MS | ≤0.5% individual impurity |
Table 2. Computational Tools for Reaction Optimization
Tool | Application | Output Metrics |
---|---|---|
Quantum chemical path search | Predicts stereoselective pathways | Activation energy, ΔG‡ |
Machine learning (ML) | Optimizes solvent/catalyst pairs | Predicted yield, selectivity |
Eigenschaften
IUPAC Name |
3-[(5-amino-6-chloropyrimidin-4-yl)amino]nonan-2-ol | |
---|---|---|
Source | PubChem | |
URL | https://pubchem.ncbi.nlm.nih.gov | |
Description | Data deposited in or computed by PubChem | |
InChI |
InChI=1S/C13H23ClN4O/c1-3-4-5-6-7-10(9(2)19)18-13-11(15)12(14)16-8-17-13/h8-10,19H,3-7,15H2,1-2H3,(H,16,17,18) | |
Source | PubChem | |
URL | https://pubchem.ncbi.nlm.nih.gov | |
Description | Data deposited in or computed by PubChem | |
InChI Key |
SBFQZPXJGBALKB-UHFFFAOYSA-N | |
Source | PubChem | |
URL | https://pubchem.ncbi.nlm.nih.gov | |
Description | Data deposited in or computed by PubChem | |
Canonical SMILES |
CCCCCCC(C(C)O)NC1=C(C(=NC=N1)Cl)N | |
Source | PubChem | |
URL | https://pubchem.ncbi.nlm.nih.gov | |
Description | Data deposited in or computed by PubChem | |
Molecular Formula |
C13H23ClN4O | |
Source | PubChem | |
URL | https://pubchem.ncbi.nlm.nih.gov | |
Description | Data deposited in or computed by PubChem | |
DSSTOX Substance ID |
DTXSID40974690 | |
Record name | 3-[(5-Amino-6-chloropyrimidin-4-yl)amino]nonan-2-ol | |
Source | EPA DSSTox | |
URL | https://comptox.epa.gov/dashboard/DTXSID40974690 | |
Description | DSSTox provides a high quality public chemistry resource for supporting improved predictive toxicology. | |
Molecular Weight |
286.80 g/mol | |
Source | PubChem | |
URL | https://pubchem.ncbi.nlm.nih.gov | |
Description | Data deposited in or computed by PubChem | |
CAS No. |
59262-85-0 | |
Record name | NSC272455 | |
Source | DTP/NCI | |
URL | https://dtp.cancer.gov/dtpstandard/servlet/dwindex?searchtype=NSC&outputformat=html&searchlist=272455 | |
Description | The NCI Development Therapeutics Program (DTP) provides services and resources to the academic and private-sector research communities worldwide to facilitate the discovery and development of new cancer therapeutic agents. | |
Explanation | Unless otherwise indicated, all text within NCI products is free of copyright and may be reused without our permission. Credit the National Cancer Institute as the source. | |
Record name | 3-[(5-Amino-6-chloropyrimidin-4-yl)amino]nonan-2-ol | |
Source | EPA DSSTox | |
URL | https://comptox.epa.gov/dashboard/DTXSID40974690 | |
Description | DSSTox provides a high quality public chemistry resource for supporting improved predictive toxicology. | |
Retrosynthesis Analysis
AI-Powered Synthesis Planning: Our tool employs the Template_relevance Pistachio, Template_relevance Bkms_metabolic, Template_relevance Pistachio_ringbreaker, Template_relevance Reaxys, Template_relevance Reaxys_biocatalysis model, leveraging a vast database of chemical reactions to predict feasible synthetic routes.
One-Step Synthesis Focus: Specifically designed for one-step synthesis, it provides concise and direct routes for your target compounds, streamlining the synthesis process.
Accurate Predictions: Utilizing the extensive PISTACHIO, BKMS_METABOLIC, PISTACHIO_RINGBREAKER, REAXYS, REAXYS_BIOCATALYSIS database, our tool offers high-accuracy predictions, reflecting the latest in chemical research and data.
Strategy Settings
Precursor scoring | Relevance Heuristic |
---|---|
Min. plausibility | 0.01 |
Model | Template_relevance |
Template Set | Pistachio/Bkms_metabolic/Pistachio_ringbreaker/Reaxys/Reaxys_biocatalysis |
Top-N result to add to graph | 6 |
Feasible Synthetic Routes
Haftungsausschluss und Informationen zu In-Vitro-Forschungsprodukten
Bitte beachten Sie, dass alle Artikel und Produktinformationen, die auf BenchChem präsentiert werden, ausschließlich zu Informationszwecken bestimmt sind. Die auf BenchChem zum Kauf angebotenen Produkte sind speziell für In-vitro-Studien konzipiert, die außerhalb lebender Organismen durchgeführt werden. In-vitro-Studien, abgeleitet von dem lateinischen Begriff "in Glas", beinhalten Experimente, die in kontrollierten Laborumgebungen unter Verwendung von Zellen oder Geweben durchgeführt werden. Es ist wichtig zu beachten, dass diese Produkte nicht als Arzneimittel oder Medikamente eingestuft sind und keine Zulassung der FDA für die Vorbeugung, Behandlung oder Heilung von medizinischen Zuständen, Beschwerden oder Krankheiten erhalten haben. Wir müssen betonen, dass jede Form der körperlichen Einführung dieser Produkte in Menschen oder Tiere gesetzlich strikt untersagt ist. Es ist unerlässlich, sich an diese Richtlinien zu halten, um die Einhaltung rechtlicher und ethischer Standards in Forschung und Experiment zu gewährleisten.