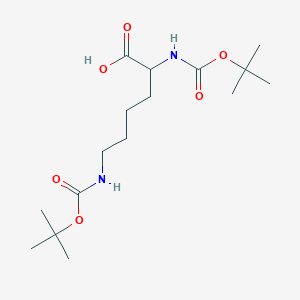
Boc-lys(boc)-OH
Übersicht
Beschreibung
(S)-2,6-Bis((tert-Butoxycarbonyl)amino)hexansäure ist ein Derivat von Lysin, einer essentiellen Aminosäure. Diese Verbindung ist durch das Vorhandensein von zwei tert-Butoxycarbonyl-geschützten Aminogruppen gekennzeichnet, was sie zu einem wertvollen Zwischenprodukt in der Peptidsynthese und anderen organischen Syntheseanwendungen macht.
Wissenschaftliche Forschungsanwendungen
(S)-2,6-Bis((tert-Butoxycarbonyl)amino)hexansäure wird in der wissenschaftlichen Forschung häufig verwendet, insbesondere in den Bereichen:
Chemie: Als Zwischenprodukt bei der Synthese von Peptiden und anderen komplexen Molekülen.
Medizin: In der Entwicklung von Peptid-basierten Medikamenten und Therapeutika.
Industrie: In der Produktion von Peptid-basierten Materialien und als Baustein für komplexere chemische Einheiten.
Wirkmechanismus
Der primäre Wirkmechanismus von (S)-2,6-Bis((tert-Butoxycarbonyl)amino)hexansäure beinhaltet seine Rolle als geschütztes Lysinderivat. Die tert-Butoxycarbonylgruppen schützen die Aminogruppen während chemischer Reaktionen und verhindern unerwünschte Nebenreaktionen. Nach der Entschützung können die freien Aminogruppen an verschiedenen biochemischen Prozessen teilnehmen, einschließlich der Peptidbindungsbildung und Enzymwechselwirkungen .
Wirkmechanismus
Target of Action
Boc-Lys(Boc)-OH, also known as Nα,Nε-Di-Boc-L-lysine , primarily targets Histone Deacetylases (HDACs) . HDACs are enzymes that catalyze the removal of acetyl groups from the ε-amino groups of conserved lysine residues in the amino terminal tail of histones . They also deacetylate non-histone proteins . Through deacetylation, HDACs regulate a variety of cellular processes including gene transcription, cell differentiation, DNA damage responses, and apoptosis .
Mode of Action
This compound interacts with its targets, the HDACs, by serving as a substrate for these enzymes . HDACs remove acetyl groups from lysine residues in histone and non-histone proteins . This deacetylation may lead to a change in the conformation and/or activity of the substrates .
Biochemical Pathways
The primary biochemical pathway affected by this compound is the lysine acetylation pathway . The lysine acetylation status of proteins is regulated dynamically through the opposing action of histone acetyltransferases (HATs) and HDACs . Deacetylation by HDACs plays an important role in the regulation of chromatin structure and gene expression . In general, hypoacetylation of histones is linked to transcriptional repression .
Pharmacokinetics
It’s known that the compound can be used in the synthesis of star poly(l-lysine) (pll) homo- and copolymers . These polymers have shown low minimum inhibitory concentration against both Gram-positive and Gram-negative bacteria , indicating potential bioavailability.
Result of Action
The result of this compound’s action is the deacetylation of histones and non-histone proteins . This can lead to changes in gene transcription, cell differentiation, DNA damage responses, and apoptosis . In the context of antimicrobial activity, star PLL homopolymers synthesized using this compound have shown enhanced antimicrobial activity and improved mammalian cell viability .
Action Environment
The action of this compound can be influenced by various environmental factors. For instance, in the synthesis of star PLL homo- and copolymers, the presence of an amine-terminated polyamidoamine dendrimer mediates the ring-opening polymerization of this compound . Additionally, the antimicrobial activity of the resulting polymers can be influenced by the presence of hydrophobic and hydroxyl groups .
Biochemische Analyse
Biochemical Properties
Boc-lys(boc)-OH is involved in the deacetylation process, a key biochemical reaction. It interacts with enzymes such as histone deacetylases (HDACs), which catalyze the removal of acetyl groups from lysine residues in histone and nonhistone proteins . The interaction between this compound and these enzymes is crucial for regulating cellular processes like gene transcription, cell differentiation, DNA damage responses, and apoptosis .
Cellular Effects
This compound has profound effects on various types of cells and cellular processes. It influences cell function by regulating gene transcription and cellular metabolism through its interaction with HDACs . Its impact on cell signaling pathways is also significant, as it can lead to changes in the conformation and/or activity of the substrates .
Molecular Mechanism
At the molecular level, this compound exerts its effects through binding interactions with biomolecules and influencing enzyme activity. It is involved in the deacetylation process, where it interacts with HDACs to remove acetyl groups from lysine residues in histone and nonhistone proteins . This can lead to changes in gene expression and influence various cellular processes .
Metabolic Pathways
This compound is involved in metabolic pathways related to the deacetylation process. It interacts with enzymes like HDACs and can influence metabolic flux or metabolite levels
Vorbereitungsmethoden
Synthesewege und Reaktionsbedingungen
Die Synthese von (S)-2,6-Bis((tert-Butoxycarbonyl)amino)hexansäure umfasst typischerweise die Schutz der Aminogruppen von Lysin mit tert-Butoxycarbonylgruppen. Dies kann unter Verwendung von Di-tert-butyl-dicarbonat in Gegenwart einer Base wie Natriumhydroxid oder 4-Dimethylaminopyridin in Acetonitril erfolgen . Die Reaktion wird normalerweise bei Umgebungstemperatur oder leicht erhöhten Temperaturen durchgeführt, um einen vollständigen Schutz der Aminogruppen zu gewährleisten.
Industrielle Produktionsverfahren
In industriellen Umgebungen erfolgt die Produktion von (S)-2,6-Bis((tert-Butoxycarbonyl)amino)hexansäure nach ähnlichen Synthesewegen, jedoch in größerem Maßstab. Der Prozess beinhaltet die Verwendung großer Reaktoren und eine präzise Steuerung der Reaktionsbedingungen, um eine hohe Ausbeute und Reinheit zu gewährleisten. Das Produkt wird dann durch Techniken wie Kristallisation oder Chromatographie gereinigt.
Chemische Reaktionsanalyse
Reaktionstypen
(S)-2,6-Bis((tert-Butoxycarbonyl)amino)hexansäure unterliegt verschiedenen chemischen Reaktionen, darunter:
Entschützungsreaktionen: Entfernung der tert-Butoxycarbonylgruppen unter Verwendung starker Säuren wie Trifluoressigsäure oder Salzsäure in Methanol.
Kupplungsreaktionen: Bildung von Peptidbindungen unter Verwendung von Kupplungsreagenzien wie N,N'-Diisopropylcarbodiimid und 1-Hydroxybenzotriazol.
Häufige Reagenzien und Bedingungen
Entschützung: Trifluoressigsäure in Dichlormethan oder Salzsäure in Methanol.
Kupplung: N,N'-Diisopropylcarbodiimid und 1-Hydroxybenzotriazol in wasserfreien Lösungsmitteln wie Dimethylformamid.
Hauptprodukte
Entschützung: Lysin oder seine Derivate.
Kupplung: Peptide oder Peptidderivate.
Analyse Chemischer Reaktionen
Types of Reactions
(S)-2,6-Bis((tert-butoxycarbonyl)amino)hexanoic acid undergoes various chemical reactions, including:
Deprotection Reactions: Removal of the tert-butoxycarbonyl groups using strong acids such as trifluoroacetic acid or hydrochloric acid in methanol.
Coupling Reactions: Formation of peptide bonds using coupling reagents like N,N’-diisopropylcarbodiimide and 1-hydroxybenzotriazole.
Common Reagents and Conditions
Deprotection: Trifluoroacetic acid in dichloromethane or hydrochloric acid in methanol.
Coupling: N,N’-diisopropylcarbodiimide and 1-hydroxybenzotriazole in anhydrous solvents like dimethylformamide.
Major Products
Deprotection: Lysine or its derivatives.
Coupling: Peptides or peptide derivatives.
Vergleich Mit ähnlichen Verbindungen
Ähnliche Verbindungen
- (S)-2-Amino-6-((tert-Butoxycarbonyl)amino)hexansäure
- (S)-2-((tert-Butoxycarbonyl)amino)-5-((2,2,10,10-Tetramethyl-4,8-dioxo-3,9-dioxa-5,7-diazaundecan-6-ylidene)amino)pentansäure
Einzigartigkeit
(S)-2,6-Bis((tert-Butoxycarbonyl)amino)hexansäure ist durch ihren doppelten Schutz der Aminogruppen einzigartig, der im Vergleich zu Verbindungen mit einer einzigen geschützten Aminogruppe eine höhere Stabilität und Vielseitigkeit in synthetischen Anwendungen bietet. Dieser doppelte Schutz ermöglicht eine komplexere und kontrollierte Synthese von Peptiden und anderen Derivaten.
Biologische Aktivität
Boc-Lys(Boc)-OH, or Nα,Nε-Di-Boc-L-lysine, is a derivative of lysine that plays a significant role in biochemical and pharmaceutical applications. This compound is characterized by its two tert-butoxycarbonyl (Boc) protecting groups, which enhance its utility in peptide synthesis and other organic reactions. The following sections detail the biological activity, mechanisms of action, and relevant research findings associated with this compound.
This compound primarily functions as a substrate for histone deacetylases (HDACs). These enzymes catalyze the removal of acetyl groups from lysine residues in proteins, influencing gene expression and cellular metabolism. The interaction with HDACs is crucial for the regulation of various cellular processes, including:
- Gene Transcription : By modulating histone acetylation levels, this compound affects chromatin structure and gene accessibility.
- Cellular Metabolism : The deacetylation process impacts metabolic pathways that are essential for energy production and cellular function .
This compound is known to participate in several biochemical pathways:
- Deacetylation Pathway : It is involved in the deacetylation of histones and non-histone proteins, which is crucial for transcriptional regulation.
- Synthesis of Peptides : As an intermediate in peptide synthesis, this compound facilitates the formation of complex peptides used in therapeutic applications .
3.1 Peptide Synthesis
This compound is extensively used in the synthesis of peptides due to its stability and ease of deprotection. It serves as a building block for various peptide sequences utilized in drug development and research:
3.2 Case Studies
Several studies have highlighted the biological activity of this compound:
- Study on HDAC Inhibition : Research demonstrated that this compound can inhibit HDAC activity, leading to increased acetylation levels in cancer cell lines, which may enhance the expression of tumor suppressor genes.
- Peptide Drug Development : A study outlined the synthesis of a peptide drug using this compound as a key intermediate, showcasing its role in generating compounds with enhanced biological activity against specific targets .
4. Pharmacokinetics
The pharmacokinetic properties of this compound indicate its potential for use in drug formulations:
- Stability : The Boc protecting groups confer stability under physiological conditions, making it suitable for various applications.
- Metabolic Pathways : Its interaction with metabolic enzymes can influence drug metabolism and efficacy .
5. Conclusion
This compound is a versatile compound with significant biological activity primarily through its interactions with HDACs and its role in peptide synthesis. Its applications span therapeutic development, biochemical research, and potential use in advanced drug delivery systems. Ongoing research continues to explore its full potential in various scientific fields.
Eigenschaften
IUPAC Name |
(2S)-2,6-bis[(2-methylpropan-2-yl)oxycarbonylamino]hexanoic acid | |
---|---|---|
Source | PubChem | |
URL | https://pubchem.ncbi.nlm.nih.gov | |
Description | Data deposited in or computed by PubChem | |
InChI |
InChI=1S/C16H30N2O6/c1-15(2,3)23-13(21)17-10-8-7-9-11(12(19)20)18-14(22)24-16(4,5)6/h11H,7-10H2,1-6H3,(H,17,21)(H,18,22)(H,19,20)/t11-/m0/s1 | |
Source | PubChem | |
URL | https://pubchem.ncbi.nlm.nih.gov | |
Description | Data deposited in or computed by PubChem | |
InChI Key |
FBVSXKMMQOZUNU-NSHDSACASA-N | |
Source | PubChem | |
URL | https://pubchem.ncbi.nlm.nih.gov | |
Description | Data deposited in or computed by PubChem | |
Canonical SMILES |
CC(C)(C)OC(=O)NCCCCC(C(=O)O)NC(=O)OC(C)(C)C | |
Source | PubChem | |
URL | https://pubchem.ncbi.nlm.nih.gov | |
Description | Data deposited in or computed by PubChem | |
Isomeric SMILES |
CC(C)(C)OC(=O)NCCCC[C@@H](C(=O)O)NC(=O)OC(C)(C)C | |
Source | PubChem | |
URL | https://pubchem.ncbi.nlm.nih.gov | |
Description | Data deposited in or computed by PubChem | |
Molecular Formula |
C16H30N2O6 | |
Source | PubChem | |
URL | https://pubchem.ncbi.nlm.nih.gov | |
Description | Data deposited in or computed by PubChem | |
DSSTOX Substance ID |
DTXSID10428554 | |
Record name | BOC-LYS(BOC)-OH | |
Source | EPA DSSTox | |
URL | https://comptox.epa.gov/dashboard/DTXSID10428554 | |
Description | DSSTox provides a high quality public chemistry resource for supporting improved predictive toxicology. | |
Molecular Weight |
346.42 g/mol | |
Source | PubChem | |
URL | https://pubchem.ncbi.nlm.nih.gov | |
Description | Data deposited in or computed by PubChem | |
CAS No. |
2483-46-7 | |
Record name | BOC-LYS(BOC)-OH | |
Source | EPA DSSTox | |
URL | https://comptox.epa.gov/dashboard/DTXSID10428554 | |
Description | DSSTox provides a high quality public chemistry resource for supporting improved predictive toxicology. | |
Record name | (2S)-2,6-bis({[(tert-butoxy)carbonyl]amino})hexanoic acid | |
Source | European Chemicals Agency (ECHA) | |
URL | https://echa.europa.eu/information-on-chemicals | |
Description | The European Chemicals Agency (ECHA) is an agency of the European Union which is the driving force among regulatory authorities in implementing the EU's groundbreaking chemicals legislation for the benefit of human health and the environment as well as for innovation and competitiveness. | |
Explanation | Use of the information, documents and data from the ECHA website is subject to the terms and conditions of this Legal Notice, and subject to other binding limitations provided for under applicable law, the information, documents and data made available on the ECHA website may be reproduced, distributed and/or used, totally or in part, for non-commercial purposes provided that ECHA is acknowledged as the source: "Source: European Chemicals Agency, http://echa.europa.eu/". Such acknowledgement must be included in each copy of the material. ECHA permits and encourages organisations and individuals to create links to the ECHA website under the following cumulative conditions: Links can only be made to webpages that provide a link to the Legal Notice page. | |
Retrosynthesis Analysis
AI-Powered Synthesis Planning: Our tool employs the Template_relevance Pistachio, Template_relevance Bkms_metabolic, Template_relevance Pistachio_ringbreaker, Template_relevance Reaxys, Template_relevance Reaxys_biocatalysis model, leveraging a vast database of chemical reactions to predict feasible synthetic routes.
One-Step Synthesis Focus: Specifically designed for one-step synthesis, it provides concise and direct routes for your target compounds, streamlining the synthesis process.
Accurate Predictions: Utilizing the extensive PISTACHIO, BKMS_METABOLIC, PISTACHIO_RINGBREAKER, REAXYS, REAXYS_BIOCATALYSIS database, our tool offers high-accuracy predictions, reflecting the latest in chemical research and data.
Strategy Settings
Precursor scoring | Relevance Heuristic |
---|---|
Min. plausibility | 0.01 |
Model | Template_relevance |
Template Set | Pistachio/Bkms_metabolic/Pistachio_ringbreaker/Reaxys/Reaxys_biocatalysis |
Top-N result to add to graph | 6 |
Feasible Synthetic Routes
Haftungsausschluss und Informationen zu In-Vitro-Forschungsprodukten
Bitte beachten Sie, dass alle Artikel und Produktinformationen, die auf BenchChem präsentiert werden, ausschließlich zu Informationszwecken bestimmt sind. Die auf BenchChem zum Kauf angebotenen Produkte sind speziell für In-vitro-Studien konzipiert, die außerhalb lebender Organismen durchgeführt werden. In-vitro-Studien, abgeleitet von dem lateinischen Begriff "in Glas", beinhalten Experimente, die in kontrollierten Laborumgebungen unter Verwendung von Zellen oder Geweben durchgeführt werden. Es ist wichtig zu beachten, dass diese Produkte nicht als Arzneimittel oder Medikamente eingestuft sind und keine Zulassung der FDA für die Vorbeugung, Behandlung oder Heilung von medizinischen Zuständen, Beschwerden oder Krankheiten erhalten haben. Wir müssen betonen, dass jede Form der körperlichen Einführung dieser Produkte in Menschen oder Tiere gesetzlich strikt untersagt ist. Es ist unerlässlich, sich an diese Richtlinien zu halten, um die Einhaltung rechtlicher und ethischer Standards in Forschung und Experiment zu gewährleisten.