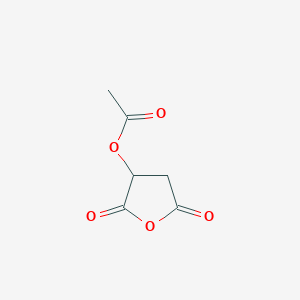
2,5-Dioxooxolan-3-YL acetate
Übersicht
Beschreibung
2,5-Dioxooxolan-3-YL acetate, also known as this compound, is a useful research compound. Its molecular formula is C6H6O5 and its molecular weight is 158.11 g/mol. The purity is usually 95%.
BenchChem offers high-quality this compound suitable for many research applications. Different packaging options are available to accommodate customers' requirements. Please inquire for more information about this compound including the price, delivery time, and more detailed information at info@benchchem.com.
Biologische Aktivität
2,5-Dioxooxolan-3-YL acetate, with the molecular formula C₆H₆O₅, is a cyclic ester characterized by its unique dioxolane structure. This compound has garnered attention in recent years for its potential biological activities, including antimicrobial and anticancer properties. Understanding its biological activity is crucial for exploring its applications in medicinal chemistry and organic synthesis.
Chemical Structure and Properties
The structural features of this compound include:
- Dioxolane Ring : The presence of a dioxolane moiety contributes to the compound's reactivity.
- Acetate Group : This functional group enhances solubility and reactivity in biological systems.
The compound's reactivity is largely attributed to the two carbonyl groups located at the 2 and 5 positions of the oxolane ring, which play a significant role in its interactions with biological molecules.
Synthesis Methods
The synthesis of this compound can be achieved through various methods, including:
- Esterification : Reaction of dioxooxolane derivatives with acetic acid or acetyl chloride.
- Cyclization Reactions : Involving precursor compounds that form the dioxolane structure under specific conditions.
These methods highlight the importance of controlling reaction conditions to optimize yield and purity.
Antimicrobial Properties
Research indicates that this compound exhibits significant antimicrobial activity against various bacterial strains. For instance, studies have shown effectiveness against both Gram-positive and Gram-negative bacteria, suggesting its potential as a lead compound for developing new antibiotics .
Anticancer Activity
In vitro studies have demonstrated that this compound may induce apoptosis in cancer cell lines. The mechanism appears to involve the inhibition of key enzymes involved in cell proliferation and survival pathways. For example, preliminary results indicated that treatment with this compound led to increased levels of apoptotic markers in cultured cancer cells .
Case Studies
Several case studies have explored the biological activity of this compound:
-
Study on Antimicrobial Effects :
- Objective : To evaluate the efficacy against Staphylococcus aureus and Escherichia coli.
- Methodology : Disk diffusion method was employed to assess inhibition zones.
- Results : Significant inhibition was observed at concentrations above 100 µg/mL.
-
Study on Anticancer Properties :
- Objective : To investigate the pro-apoptotic effects on human breast cancer cells (MCF-7).
- Methodology : Flow cytometry was used to analyze apoptotic cell death.
- Results : A dose-dependent increase in apoptosis was noted at concentrations ranging from 10 µM to 50 µM.
Comparative Analysis with Similar Compounds
A comparison table highlighting structural similarities and differences with related compounds is provided below:
Compound Name | Molecular Formula | Key Features |
---|---|---|
This compound | C₆H₆O₅ | Unique dioxolane structure; antimicrobial activity |
(R)-2,5-Dioxotetrahydrofuran-3-YL acetate | C₇H₈O₅ | Tetrahydrofuran ring; different reactivity |
Methyl 2-(2,5-dioxooxolan-3-yl)acetate | C₇H₈O₅ | Similar structure; explored for medicinal applications |
This comparison underscores the unique biological activity profile of this compound due to its specific structural attributes.
Eigenschaften
IUPAC Name |
(2,5-dioxooxolan-3-yl) acetate | |
---|---|---|
Source | PubChem | |
URL | https://pubchem.ncbi.nlm.nih.gov | |
Description | Data deposited in or computed by PubChem | |
InChI |
InChI=1S/C6H6O5/c1-3(7)10-4-2-5(8)11-6(4)9/h4H,2H2,1H3 | |
Source | PubChem | |
URL | https://pubchem.ncbi.nlm.nih.gov | |
Description | Data deposited in or computed by PubChem | |
InChI Key |
SSWJHSASZZAIAU-UHFFFAOYSA-N | |
Source | PubChem | |
URL | https://pubchem.ncbi.nlm.nih.gov | |
Description | Data deposited in or computed by PubChem | |
Canonical SMILES |
CC(=O)OC1CC(=O)OC1=O | |
Source | PubChem | |
URL | https://pubchem.ncbi.nlm.nih.gov | |
Description | Data deposited in or computed by PubChem | |
Molecular Formula |
C6H6O5 | |
Source | PubChem | |
URL | https://pubchem.ncbi.nlm.nih.gov | |
Description | Data deposited in or computed by PubChem | |
DSSTOX Substance ID |
DTXSID50337171 | |
Record name | 2,5-Dioxooxolan-3-yl acetate | |
Source | EPA DSSTox | |
URL | https://comptox.epa.gov/dashboard/DTXSID50337171 | |
Description | DSSTox provides a high quality public chemistry resource for supporting improved predictive toxicology. | |
Molecular Weight |
158.11 g/mol | |
Source | PubChem | |
URL | https://pubchem.ncbi.nlm.nih.gov | |
Description | Data deposited in or computed by PubChem | |
CAS No. |
24766-96-9 | |
Record name | 2,5-Dioxooxolan-3-yl acetate | |
Source | EPA DSSTox | |
URL | https://comptox.epa.gov/dashboard/DTXSID50337171 | |
Description | DSSTox provides a high quality public chemistry resource for supporting improved predictive toxicology. | |
Synthesis routes and methods
Procedure details
Retrosynthesis Analysis
AI-Powered Synthesis Planning: Our tool employs the Template_relevance Pistachio, Template_relevance Bkms_metabolic, Template_relevance Pistachio_ringbreaker, Template_relevance Reaxys, Template_relevance Reaxys_biocatalysis model, leveraging a vast database of chemical reactions to predict feasible synthetic routes.
One-Step Synthesis Focus: Specifically designed for one-step synthesis, it provides concise and direct routes for your target compounds, streamlining the synthesis process.
Accurate Predictions: Utilizing the extensive PISTACHIO, BKMS_METABOLIC, PISTACHIO_RINGBREAKER, REAXYS, REAXYS_BIOCATALYSIS database, our tool offers high-accuracy predictions, reflecting the latest in chemical research and data.
Strategy Settings
Precursor scoring | Relevance Heuristic |
---|---|
Min. plausibility | 0.01 |
Model | Template_relevance |
Template Set | Pistachio/Bkms_metabolic/Pistachio_ringbreaker/Reaxys/Reaxys_biocatalysis |
Top-N result to add to graph | 6 |
Feasible Synthetic Routes
Q1: How does the modification of β-CD COFs with (3R,4R)-4-acetyloxy-2,5-dioxooxolan-3-yl acetate impact their chiral recognition abilities?
A1: The research paper demonstrates that modifying β-CD COFs with (3R,4R)-4-acetyloxy-2,5-dioxooxolan-3-yl acetate significantly enhances their chiral recognition abilities for specific drug enantiomers, such as dihydropyridines and fluoroquinolones []. This enhancement is attributed to the introduction of specific functional groups that interact differently with each enantiomer. While unmodified β-CD COFBPDA lacked chiral recognition for these drugs, the modified frameworks successfully separated the enantiomers in capillary electrochromatography. This difference suggests that the functional groups introduced by (3R,4R)-4-acetyloxy-2,5-dioxooxolan-3-yl acetate play a crucial role in creating a chiral environment within the β-CD COF structure, enabling selective interaction and separation of enantiomers. Molecular docking simulations further support this mechanism by revealing distinct binding affinities and orientations for each enantiomer within the modified framework [].
Haftungsausschluss und Informationen zu In-Vitro-Forschungsprodukten
Bitte beachten Sie, dass alle Artikel und Produktinformationen, die auf BenchChem präsentiert werden, ausschließlich zu Informationszwecken bestimmt sind. Die auf BenchChem zum Kauf angebotenen Produkte sind speziell für In-vitro-Studien konzipiert, die außerhalb lebender Organismen durchgeführt werden. In-vitro-Studien, abgeleitet von dem lateinischen Begriff "in Glas", beinhalten Experimente, die in kontrollierten Laborumgebungen unter Verwendung von Zellen oder Geweben durchgeführt werden. Es ist wichtig zu beachten, dass diese Produkte nicht als Arzneimittel oder Medikamente eingestuft sind und keine Zulassung der FDA für die Vorbeugung, Behandlung oder Heilung von medizinischen Zuständen, Beschwerden oder Krankheiten erhalten haben. Wir müssen betonen, dass jede Form der körperlichen Einführung dieser Produkte in Menschen oder Tiere gesetzlich strikt untersagt ist. Es ist unerlässlich, sich an diese Richtlinien zu halten, um die Einhaltung rechtlicher und ethischer Standards in Forschung und Experiment zu gewährleisten.