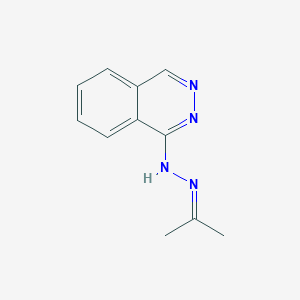
Hydralazine acetone hydrazone
Übersicht
Beschreibung
Hydralazine is a vasodilator that works by relaxing the muscles in your blood vessels to help them dilate (widen). This lowers blood pressure and allows blood to flow more easily through your veins and arteries . Acetone hydrazone (isopropylidenehydrazine) is the product of condensation of acetone and hydrazine, as typical for hydrazone formation .
Synthesis Analysis
A hydrazone ligand, 4-chlorobenzaldehyde hydralazine hydrazone (LH), has been synthesized. Initial attempts to synthesize Co+2, Ni+2, Cu+2 and Zn+2 metal complexes of LH resulted in a cyclized triazole product (T). The structure of both the LH and T is characterized by single-crystal X-ray diffraction pattern analysis .Molecular Structure Analysis
The molecular structure of Hydralazine acetone hydrazone is C11H12N4 . It is the product of condensation of acetone and hydrazine .Chemical Reactions Analysis
The reaction of hydralazine with various biogenic aldehydes and ketones (acetone, pyruvic acid, acetoacetic acid, formaldehyde, and acetaldehyde) in pH 7.4 buffer was studied for potential alterations in hydralazine pharmacokinetics secondary to alcoholism and diabetes .Physical And Chemical Properties Analysis
Hydralazine acetone hydrazone has a molecular weight of 200.24 g/mol . The pharmacokinetics of hydralazine acetone hydrazone (HAH), which is a metabolite of hydralazine (HP), was investigated after iv administration to rats .Wissenschaftliche Forschungsanwendungen
Synthesis of Quinazolines and Schiff Bases
Hydralazine acetone hydrazone is utilized in the synthesis of quinazolines and Schiff bases , which are compounds with significant medicinal properties . These substances have been studied for their potential anti-inflammatory and analgesic activities, making them valuable in pharmaceutical research.
Antimicrobial and Anticancer Activities
The compound has shown promise in the development of new medications with antimicrobial and anticancer activities . Its unique biological action and excellent coordination ability make it a subject of interest for creating treatments that target cell death pathways such as apoptosis and autophagy.
Controlled Drug Release
In biomedical applications, hydralazine acetone hydrazone can be used to link anti-inflammatory drugs for controlled release . This application is crucial for developing drug delivery systems that can provide consistent therapeutic effects over extended periods.
Colorimetric Sensors
The compound’s derivatives have been explored for their use as colorimetric sensors due to their ability to change color in response to environmental factors . This property is particularly useful in creating sensors that can detect changes in temperature or the presence of specific substances.
Antihypertensive Effects
Hydralazine acetone hydrazone itself has been identified as one of the metabolic products of the antihypertensive pharmaceutical hydralazine and has shown to possess antihypertensive effects . This application is significant for the development of new blood pressure-lowering medications.
Intermediate in Organic Synthesis
The compound serves as an intermediate in the synthesis of various organic compounds, including 2-diazopropane . Its role as an intermediate is essential in the production of other chemicals that have diverse applications in industry and research.
Safety And Hazards
Hydralazine is primarily used to treat hypertension and hypertensive urgency/emergency . Adverse effects include the anticipated tachycardia, fluid retention, and headache, caused by the vasodilation, especially in the early days of therapy, but may frequently be prevented by the concomitant use of a β-blocker .
Zukünftige Richtungen
There is a critical need to use hydralazine in some patients with resistant hypertension. Available evidence supports a significant link between genotype and NAT2 enzyme activity as 29 studies were identified with an overall concordance between genotype and phenotype of 92% . With appropriate guidance on the usage of NAT2 genotype, clinicians can adopt a personalized approach to hydralazine dosing and prescription, enabling more efficient and safe treatment of resistant hypertension .
Eigenschaften
IUPAC Name |
N-(propan-2-ylideneamino)phthalazin-1-amine | |
---|---|---|
Source | PubChem | |
URL | https://pubchem.ncbi.nlm.nih.gov | |
Description | Data deposited in or computed by PubChem | |
InChI |
InChI=1S/C11H12N4/c1-8(2)13-15-11-10-6-4-3-5-9(10)7-12-14-11/h3-7H,1-2H3,(H,14,15) | |
Source | PubChem | |
URL | https://pubchem.ncbi.nlm.nih.gov | |
Description | Data deposited in or computed by PubChem | |
InChI Key |
AKFJXINWDQGPHO-UHFFFAOYSA-N | |
Source | PubChem | |
URL | https://pubchem.ncbi.nlm.nih.gov | |
Description | Data deposited in or computed by PubChem | |
Canonical SMILES |
CC(=NNC1=NN=CC2=CC=CC=C21)C | |
Source | PubChem | |
URL | https://pubchem.ncbi.nlm.nih.gov | |
Description | Data deposited in or computed by PubChem | |
Molecular Formula |
C11H12N4 | |
Source | PubChem | |
URL | https://pubchem.ncbi.nlm.nih.gov | |
Description | Data deposited in or computed by PubChem | |
DSSTOX Substance ID |
DTXSID60204704 | |
Record name | Hydralazine acetone hydrazone | |
Source | EPA DSSTox | |
URL | https://comptox.epa.gov/dashboard/DTXSID60204704 | |
Description | DSSTox provides a high quality public chemistry resource for supporting improved predictive toxicology. | |
Molecular Weight |
200.24 g/mol | |
Source | PubChem | |
URL | https://pubchem.ncbi.nlm.nih.gov | |
Description | Data deposited in or computed by PubChem | |
Product Name |
Hydralazine acetone hydrazone | |
CAS RN |
56173-18-3 | |
Record name | 1(2H)-Phthalazinone, (1-methylethylidene)hydrazone | |
Source | CAS Common Chemistry | |
URL | https://commonchemistry.cas.org/detail?cas_rn=56173-18-3 | |
Description | CAS Common Chemistry is an open community resource for accessing chemical information. Nearly 500,000 chemical substances from CAS REGISTRY cover areas of community interest, including common and frequently regulated chemicals, and those relevant to high school and undergraduate chemistry classes. This chemical information, curated by our expert scientists, is provided in alignment with our mission as a division of the American Chemical Society. | |
Explanation | The data from CAS Common Chemistry is provided under a CC-BY-NC 4.0 license, unless otherwise stated. | |
Record name | Hydralazine acetone hydrazone | |
Source | ChemIDplus | |
URL | https://pubchem.ncbi.nlm.nih.gov/substance/?source=chemidplus&sourceid=0056173183 | |
Description | ChemIDplus is a free, web search system that provides access to the structure and nomenclature authority files used for the identification of chemical substances cited in National Library of Medicine (NLM) databases, including the TOXNET system. | |
Record name | Hydralazine acetone hydrazone | |
Source | EPA DSSTox | |
URL | https://comptox.epa.gov/dashboard/DTXSID60204704 | |
Description | DSSTox provides a high quality public chemistry resource for supporting improved predictive toxicology. | |
Record name | Hydralazine acetone hydrazone | |
Source | Human Metabolome Database (HMDB) | |
URL | http://www.hmdb.ca/metabolites/HMDB0060606 | |
Description | The Human Metabolome Database (HMDB) is a freely available electronic database containing detailed information about small molecule metabolites found in the human body. | |
Explanation | HMDB is offered to the public as a freely available resource. Use and re-distribution of the data, in whole or in part, for commercial purposes requires explicit permission of the authors and explicit acknowledgment of the source material (HMDB) and the original publication (see the HMDB citing page). We ask that users who download significant portions of the database cite the HMDB paper in any resulting publications. | |
Retrosynthesis Analysis
AI-Powered Synthesis Planning: Our tool employs the Template_relevance Pistachio, Template_relevance Bkms_metabolic, Template_relevance Pistachio_ringbreaker, Template_relevance Reaxys, Template_relevance Reaxys_biocatalysis model, leveraging a vast database of chemical reactions to predict feasible synthetic routes.
One-Step Synthesis Focus: Specifically designed for one-step synthesis, it provides concise and direct routes for your target compounds, streamlining the synthesis process.
Accurate Predictions: Utilizing the extensive PISTACHIO, BKMS_METABOLIC, PISTACHIO_RINGBREAKER, REAXYS, REAXYS_BIOCATALYSIS database, our tool offers high-accuracy predictions, reflecting the latest in chemical research and data.
Strategy Settings
Precursor scoring | Relevance Heuristic |
---|---|
Min. plausibility | 0.01 |
Model | Template_relevance |
Template Set | Pistachio/Bkms_metabolic/Pistachio_ringbreaker/Reaxys/Reaxys_biocatalysis |
Top-N result to add to graph | 6 |
Feasible Synthetic Routes
Q & A
Q1: What is the mechanism of action of Hydralazine acetone hydrazone (HAH) as a vasodilator?
A: While HAH itself possesses some vasodilatory activity, its primary mechanism involves conversion back to its parent compound, Hydralazine (H), in vivo. [, ] Both HAH and H relax pre-contracted vascular smooth muscle and inhibit the development of contractions induced by potassium and norepinephrine. [] This effect is attributed to their interaction with calcium (Ca++) flux, both from extracellular sources and intracellular stores. [] Essentially, HAH acts as a prodrug, being metabolized into the active compound, Hydralazine.
Q2: How does the vasodilatory potency of Hydralazine acetone hydrazone (HAH) compare to Hydralazine (H)?
A: Research suggests that HAH exhibits a lower vasodilatory potency compared to its parent compound, H. In hypertensive rabbits, HAH demonstrated a potency ratio of approximately 0.2 relative to H, indicating that a higher dose of HAH is required to achieve a comparable hypotensive effect. []
Q3: What is the metabolic fate of Hydralazine acetone hydrazone (HAH) in vivo?
A: Studies in rats [] and rabbits [] demonstrate that HAH undergoes enzymatic hydrolysis to yield Hydralazine (H) in vivo. Furthermore, HAH can be converted to Hydralazine pyruvic acid hydrazone (HPH) through two pathways: direct reaction with pyruvic acid or indirectly via conversion to H first. [] This metabolic conversion of HAH to both H and HPH plays a crucial role in its overall pharmacological activity.
Q4: Are there analytical methods available to study the pharmacokinetics of HAH and its metabolites?
A: Researchers have successfully employed high-performance liquid chromatography (HPLC) to simultaneously quantify plasma concentrations of HAH, H, and HPH. [] This method allows for a detailed understanding of the pharmacokinetic profile of HAH and its metabolites, providing insights into absorption, distribution, metabolism, and excretion.
Q5: What is the significance of studying the structure-activity relationship (SAR) of Hydralazine and its derivatives?
A: Understanding the SAR is crucial for designing more effective and safer vasodilators. Studies comparing HAH with Hydralazine butanone hydrazone (HBH) [] contribute to this understanding. Investigating how structural modifications impact the interactions with contractile mechanisms in vascular smooth muscle can guide the development of novel therapeutic agents with improved pharmacological profiles.
Haftungsausschluss und Informationen zu In-Vitro-Forschungsprodukten
Bitte beachten Sie, dass alle Artikel und Produktinformationen, die auf BenchChem präsentiert werden, ausschließlich zu Informationszwecken bestimmt sind. Die auf BenchChem zum Kauf angebotenen Produkte sind speziell für In-vitro-Studien konzipiert, die außerhalb lebender Organismen durchgeführt werden. In-vitro-Studien, abgeleitet von dem lateinischen Begriff "in Glas", beinhalten Experimente, die in kontrollierten Laborumgebungen unter Verwendung von Zellen oder Geweben durchgeführt werden. Es ist wichtig zu beachten, dass diese Produkte nicht als Arzneimittel oder Medikamente eingestuft sind und keine Zulassung der FDA für die Vorbeugung, Behandlung oder Heilung von medizinischen Zuständen, Beschwerden oder Krankheiten erhalten haben. Wir müssen betonen, dass jede Form der körperlichen Einführung dieser Produkte in Menschen oder Tiere gesetzlich strikt untersagt ist. Es ist unerlässlich, sich an diese Richtlinien zu halten, um die Einhaltung rechtlicher und ethischer Standards in Forschung und Experiment zu gewährleisten.