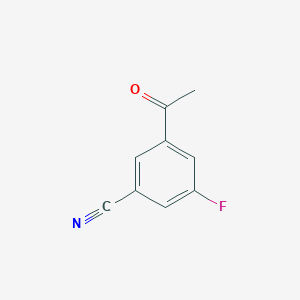
3-Acetyl-5-fluorobenzonitrile
Übersicht
Beschreibung
2-Cyano-3,12-dioxoolean-1,9-dien-28-carbonsäure (CDDO) ist ein synthetisches Triterpenoid, das von Oleanolsäure abgeleitet ist, einer natürlichen Verbindung, die in verschiedenen Pflanzen vorkommt. CDDO wurde entwickelt, um die entzündungshemmenden Eigenschaften von Oleanolsäure zu verbessern und hat in verschiedenen therapeutischen Anwendungen, einschließlich Antikrebs-, Entzündungshemmungs- und antioxidativen Aktivitäten, ein großes Potenzial gezeigt .
Vorbereitungsmethoden
Synthesewege und Reaktionsbedingungen
CDDO wird aus Oleanolsäure durch eine Reihe chemischer Reaktionen synthetisiert. Die Synthese beinhaltet die Einführung einer Cyanogruppe an der C-2-Position und die Bildung einer Dioxogruppe an den C-3- und C-12-Positionen. Die wichtigsten Schritte der Synthese sind:
Oxidation: Oleanolsäure wird zu 3-Oxo-oleanolsäure oxidiert.
Cyanierung: Die 3-Oxo-oleanolsäure wird dann mit einem Cyanierungsmittel umgesetzt, um die Cyanogruppe an der C-2-Position einzuführen.
Bildung von Dioxogruppen: Der letzte Schritt beinhaltet die Bildung von Dioxogruppen an den C-3- und C-12-Positionen durch eine Reihe von Oxidationsreaktionen
Industrielle Produktionsmethoden
Die industrielle Produktion von CDDO folgt ähnlichen Synthesewegen, wird aber für die großtechnische Produktion optimiert. Dies beinhaltet die Verwendung effizienter Katalysatoren und Reaktionsbedingungen, um die Ausbeute zu maximieren und Nebenprodukte zu minimieren. Der Prozess wird typischerweise in Batchreaktoren durchgeführt, wobei Temperatur, Druck und Reaktionszeit streng kontrolliert werden, um eine gleichbleibende Produktqualität zu gewährleisten .
Chemische Reaktionsanalyse
Arten von Reaktionen
CDDO durchläuft verschiedene Arten von chemischen Reaktionen, darunter:
Oxidation: CDDO kann weiter oxidiert werden, um zusätzliche funktionelle Gruppen zu bilden.
Reduktion: Reduktionsreaktionen können die Dioxogruppen zu Hydroxylgruppen modifizieren.
Substitution: Die Cyanogruppe kann durch andere funktionelle Gruppen substituiert werden, um Derivate mit unterschiedlichen Eigenschaften zu erzeugen
Häufige Reagenzien und Bedingungen
Häufige Reagenzien, die bei den Reaktionen von CDDO verwendet werden, sind:
Oxidationsmittel: Wie Kaliumpermanganat und Chromtrioxid.
Reduktionsmittel: Wie Natriumborhydrid und Lithiumaluminiumhydrid.
Cyanierungsmittel: Wie Cyanbromid und Natriumcyanid
Hauptprodukte
Die Hauptprodukte, die aus den Reaktionen von CDDO gebildet werden, umfassen verschiedene Derivate mit modifizierten funktionellen Gruppen. Diese Derivate können verstärkte oder veränderte biologische Aktivitäten haben, was sie für verschiedene therapeutische Anwendungen nützlich macht .
Wissenschaftliche Forschungsanwendungen
Chemie: CDDO und seine Derivate werden als Zwischenprodukte bei der Synthese anderer komplexer Moleküle verwendet.
Biologie: CDDO hat gezeigt, dass es verschiedene biologische Pfade moduliert, einschließlich derer, die an Entzündungen und oxidativem Stress beteiligt sind.
Medizin: CDDO hat sich als potenzieller Antikrebswirkstoff erwiesen, indem es Apoptose in Krebszellen induziert. Es hat auch entzündungshemmende und antioxidative Eigenschaften, was es für die Behandlung chronischer Krankheiten wie Diabetes und Nierenerkrankungen nützlich macht.
Industrie: CDDO wird bei der Entwicklung neuer Medikamente und als Forschungswerkzeug in der Medikamentenforschung eingesetzt
Wirkmechanismus
CDDO übt seine Wirkungen durch mehrere Mechanismen aus:
Nrf2-Signalweg: CDDO aktiviert den Kernfaktor Erythroid 2-assoziierten Faktor 2 (Nrf2)-Signalweg, was zur Hochregulierung von antioxidativen Antwortgenen führt. Dies trägt zum Schutz der Zellen vor oxidativem Stress bei.
Apoptose-Induktion: CDDO induziert Apoptose in Krebszellen durch sowohl intrinsische als auch extrinsische Signalwege. .
Entzündungshemmende Wirkungen: CDDO hemmt die Produktion von proinflammatorischen Zytokinen und reduziert Entzündungen, indem es die Aktivität des Kernfaktors Kappa B (NF-κB) und anderer Entzündungsmediatoren moduliert
Analyse Chemischer Reaktionen
Types of Reactions
CDDO undergoes various types of chemical reactions, including:
Oxidation: CDDO can be further oxidized to form additional functional groups.
Reduction: Reduction reactions can modify the dioxo groups to hydroxyl groups.
Substitution: The cyano group can be substituted with other functional groups to create derivatives with different properties
Common Reagents and Conditions
Common reagents used in the reactions of CDDO include:
Oxidizing Agents: Such as potassium permanganate and chromium trioxide.
Reducing Agents: Such as sodium borohydride and lithium aluminum hydride.
Cyanating Agents: Such as cyanogen bromide and sodium cyanide
Major Products
The major products formed from the reactions of CDDO include various derivatives with modified functional groups. These derivatives can have enhanced or altered biological activities, making them useful for different therapeutic applications .
Wissenschaftliche Forschungsanwendungen
Chemistry: CDDO and its derivatives are used as intermediates in the synthesis of other complex molecules.
Biology: CDDO has been shown to modulate various biological pathways, including those involved in inflammation and oxidative stress.
Medicine: CDDO has demonstrated potential as an anti-cancer agent by inducing apoptosis in cancer cells. It also has anti-inflammatory and antioxidant properties, making it useful in the treatment of chronic diseases such as diabetes and kidney disease.
Industry: CDDO is used in the development of new pharmaceuticals and as a research tool in drug discovery
Wirkmechanismus
CDDO exerts its effects through multiple mechanisms:
Nrf2 Pathway: CDDO activates the nuclear factor erythroid 2-related factor 2 (Nrf2) pathway, leading to the upregulation of antioxidant response genes. This helps protect cells from oxidative stress.
Apoptosis Induction: CDDO induces apoptosis in cancer cells through both intrinsic and extrinsic pathways. .
Anti-inflammatory Effects: CDDO inhibits the production of pro-inflammatory cytokines and reduces inflammation by modulating the activity of nuclear factor kappa B (NF-κB) and other inflammatory mediators
Vergleich Mit ähnlichen Verbindungen
CDDO gehört zu einer Klasse von Verbindungen, die als Triterpenoide bekannt sind. Ähnliche Verbindungen sind:
CDDO-Me (Bardoxolon Methyl): Ein Methylester-Derivat von CDDO mit verbesserten entzündungshemmenden und antikankereigenschaften.
CDDO-Im (Imidazolid-Derivat): Ein Derivat mit verbesserter Löslichkeit und Bioverfügbarkeit.
Oleanolsäure: Der natürliche Vorläufer von CDDO mit einer schwächeren entzündungshemmenden Aktivität
CDDO zeichnet sich durch seine starken biologischen Aktivitäten und seine Vielseitigkeit in therapeutischen Anwendungen aus. Seine Fähigkeit, mehrere Signalwege zu modulieren und Apoptose in Krebszellen zu induzieren, macht es zu einem vielversprechenden Kandidaten für die Medikamentenentwicklung .
Eigenschaften
CAS-Nummer |
105515-21-7 |
---|---|
Molekularformel |
C9H6FNO |
Molekulargewicht |
163.15 g/mol |
IUPAC-Name |
3-acetyl-5-fluorobenzonitrile |
InChI |
InChI=1S/C9H6FNO/c1-6(12)8-2-7(5-11)3-9(10)4-8/h2-4H,1H3 |
InChI-Schlüssel |
NVTOIWPMCLKTFC-UHFFFAOYSA-N |
SMILES |
CC(=O)C1=CC(=CC(=C1)C#N)F |
Kanonische SMILES |
CC(=O)C1=CC(=CC(=C1)C#N)F |
Herkunft des Produkts |
United States |
Synthesis routes and methods I
Procedure details
Synthesis routes and methods II
Procedure details
Haftungsausschluss und Informationen zu In-Vitro-Forschungsprodukten
Bitte beachten Sie, dass alle Artikel und Produktinformationen, die auf BenchChem präsentiert werden, ausschließlich zu Informationszwecken bestimmt sind. Die auf BenchChem zum Kauf angebotenen Produkte sind speziell für In-vitro-Studien konzipiert, die außerhalb lebender Organismen durchgeführt werden. In-vitro-Studien, abgeleitet von dem lateinischen Begriff "in Glas", beinhalten Experimente, die in kontrollierten Laborumgebungen unter Verwendung von Zellen oder Geweben durchgeführt werden. Es ist wichtig zu beachten, dass diese Produkte nicht als Arzneimittel oder Medikamente eingestuft sind und keine Zulassung der FDA für die Vorbeugung, Behandlung oder Heilung von medizinischen Zuständen, Beschwerden oder Krankheiten erhalten haben. Wir müssen betonen, dass jede Form der körperlichen Einführung dieser Produkte in Menschen oder Tiere gesetzlich strikt untersagt ist. Es ist unerlässlich, sich an diese Richtlinien zu halten, um die Einhaltung rechtlicher und ethischer Standards in Forschung und Experiment zu gewährleisten.