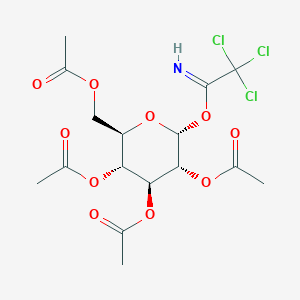
2,3,4,6-Tetra-O-acetyl-alpha-D-glucopyranosyl Trichloroacetimidate
Übersicht
Beschreibung
2,3,4,6-Tetra-O-acetyl-alpha-D-glucopyranosyl Trichloroacetimidate is a synthetic compound widely used in organic chemistry. This versatile reagent is particularly valuable for the synthesis of glycosides, glycosidic ethers, and glycosyl halides . Its unique structure allows it to participate in various chemical reactions, making it an essential tool in the field of carbohydrate chemistry.
Vorbereitungsmethoden
The synthesis of 2,3,4,6-Tetra-O-acetyl-alpha-D-glucopyranosyl Trichloroacetimidate typically involves the acetylation of D-glucose followed by the introduction of the trichloroacetimidate group. The process begins with the protection of the hydroxyl groups of D-glucose using acetic anhydride and a catalyst such as pyridine to form 2,3,4,6-tetra-O-acetyl-D-glucose. This intermediate is then reacted with trichloroacetonitrile in the presence of a base like potassium carbonate to yield the final product .
Analyse Chemischer Reaktionen
2,3,4,6-Tetra-O-acetyl-alpha-D-glucopyranosyl Trichloroacetimidate undergoes various types of chemical reactions, including:
Substitution Reactions: It can react with alcohols to form glycosides.
Hydrolysis: In the presence of water and an acid catalyst, it can hydrolyze to form the corresponding glycosyl alcohol.
Oxidation and Reduction: While specific oxidation and reduction reactions are less common, the compound can participate in these reactions under appropriate conditions.
Common reagents used in these reactions include acids, bases, and alcohols. The major products formed from these reactions are glycosides, glycosidic ethers, and glycosyl halides .
Wissenschaftliche Forschungsanwendungen
2,3,4,6-Tetra-O-acetyl-alpha-D-glucopyranosyl Trichloroacetimidate has a wide range of applications in scientific research:
Chemistry: It is used in the synthesis of complex carbohydrates and glycosylated compounds.
Biology: The compound is employed in the study of glycosylation processes and the development of glycosylated biomolecules.
Medicine: It plays a role in the synthesis of glycosylated drugs and therapeutic agents.
Industry: The compound is used in the production of various glycosylated products, including surfactants and emulsifiers
Wirkmechanismus
The mechanism of action of 2,3,4,6-Tetra-O-acetyl-alpha-D-glucopyranosyl Trichloroacetimidate involves the formation of glycosidic bonds. The trichloroacetimidate group acts as a leaving group, facilitating the nucleophilic attack by an alcohol or other nucleophile on the anomeric carbon of the glucose moiety. This results in the formation of a glycosidic bond, with the trichloroacetimidate group being replaced by the nucleophile .
Vergleich Mit ähnlichen Verbindungen
2,3,4,6-Tetra-O-acetyl-alpha-D-glucopyranosyl Trichloroacetimidate is unique due to its trichloroacetimidate group, which provides high reactivity and selectivity in glycosylation reactions. Similar compounds include:
2,3,4,6-Tetra-O-acetyl-alpha-D-glucopyranosyl Bromide: Used for similar glycosylation reactions but with different reactivity and selectivity.
2,3,4,6-Tetra-O-acetyl-beta-D-glucopyranosyl Isothiocyanate: Utilized in the synthesis of glycosylated thioureas and other derivatives
These compounds share similar structural features but differ in their leaving groups and specific applications, highlighting the versatility and uniqueness of this compound.
Biologische Aktivität
2,3,4,6-Tetra-O-acetyl-alpha-D-glucopyranosyl trichloroacetimidate is a glycosyl donor that has garnered attention in carbohydrate chemistry due to its potential applications in synthesizing oligosaccharides and other biologically active compounds. This article aims to explore the biological activity of this compound, focusing on its synthesis, mechanisms of action, and implications in pharmaceutical applications.
- IUPAC Name : [(2R,3R,4S,5R,6R)-3,4,5-tris(acetyloxy)-6-(trichloroacetamido)oxan-2-yl]methyl acetate
- Molecular Formula : C₁₄H₁₉Cl₃N₁O₉
- Molecular Weight : 411.20 g/mol
- Appearance : White to light yellow powder
- Melting Point : 87.0 to 92.0 °C
- Specific Rotation : +192° to +205° (C=3, CHCl₃)
Synthesis
The synthesis of this compound typically involves the reaction of acetylated glucopyranose with trichloroacetimidate under controlled conditions. The process can yield high-purity products suitable for further biological testing.
Antimicrobial Properties
Research indicates that carbohydrate derivatives exhibit a wide range of biological activities. Specifically, studies have shown that compounds derived from glucopyranosides can possess significant antimicrobial properties. For instance, a study demonstrated that derivatives of glucopyranosides exhibited bactericidal activity against various strains of bacteria after deacetylation processes .
The biological activity of this compound can be attributed to its ability to interact with biological membranes and proteins. The lipophilic nature of the acetyl groups enhances its penetration into cellular structures, potentially leading to disruption of microbial cell walls or interference with metabolic pathways.
Study on Antibacterial Activity
A recent study utilized the compound to evaluate its antibacterial efficacy against both Gram-positive and Gram-negative bacteria. The results indicated that the compound had a minimum inhibitory concentration (MIC) significantly lower than that of commonly used antibiotics .
Bacterial Strain | MIC (µg/mL) | Comparison Antibiotic | MIC (µg/mL) |
---|---|---|---|
Staphylococcus aureus | 32 | Penicillin | 128 |
Escherichia coli | 64 | Ampicillin | 256 |
Pseudomonas aeruginosa | 128 | Ciprofloxacin | 512 |
Cytotoxicity Assessment
Another study assessed the cytotoxic effects of this compound on human cell lines. The findings revealed that while the compound effectively inhibited bacterial growth, it exhibited low cytotoxicity towards human cells at therapeutic concentrations .
Eigenschaften
IUPAC Name |
[(2R,3R,4S,5R,6R)-3,4,5-triacetyloxy-6-(2,2,2-trichloroethanimidoyl)oxyoxan-2-yl]methyl acetate | |
---|---|---|
Source | PubChem | |
URL | https://pubchem.ncbi.nlm.nih.gov | |
Description | Data deposited in or computed by PubChem | |
InChI |
InChI=1S/C16H20Cl3NO10/c1-6(21)25-5-10-11(26-7(2)22)12(27-8(3)23)13(28-9(4)24)14(29-10)30-15(20)16(17,18)19/h10-14,20H,5H2,1-4H3/t10-,11-,12+,13-,14-/m1/s1 | |
Source | PubChem | |
URL | https://pubchem.ncbi.nlm.nih.gov | |
Description | Data deposited in or computed by PubChem | |
InChI Key |
IBUZGVQIKARDAF-RKQHYHRCSA-N | |
Source | PubChem | |
URL | https://pubchem.ncbi.nlm.nih.gov | |
Description | Data deposited in or computed by PubChem | |
Canonical SMILES |
CC(=O)OCC1C(C(C(C(O1)OC(=N)C(Cl)(Cl)Cl)OC(=O)C)OC(=O)C)OC(=O)C | |
Source | PubChem | |
URL | https://pubchem.ncbi.nlm.nih.gov | |
Description | Data deposited in or computed by PubChem | |
Isomeric SMILES |
CC(=O)OC[C@@H]1[C@H]([C@@H]([C@H]([C@H](O1)OC(=N)C(Cl)(Cl)Cl)OC(=O)C)OC(=O)C)OC(=O)C | |
Source | PubChem | |
URL | https://pubchem.ncbi.nlm.nih.gov | |
Description | Data deposited in or computed by PubChem | |
Molecular Formula |
C16H20Cl3NO10 | |
Source | PubChem | |
URL | https://pubchem.ncbi.nlm.nih.gov | |
Description | Data deposited in or computed by PubChem | |
DSSTOX Substance ID |
DTXSID60447497 | |
Record name | 2,3,4,6-Tetra-O-acetyl-1-O-(2,2,2-trichloroethanimidoyl)-alpha-D-glucopyranose | |
Source | EPA DSSTox | |
URL | https://comptox.epa.gov/dashboard/DTXSID60447497 | |
Description | DSSTox provides a high quality public chemistry resource for supporting improved predictive toxicology. | |
Molecular Weight |
492.7 g/mol | |
Source | PubChem | |
URL | https://pubchem.ncbi.nlm.nih.gov | |
Description | Data deposited in or computed by PubChem | |
CAS No. |
74808-10-9 | |
Record name | α-D-Glucopyranose, 2,3,4,6-tetraacetate 1-(2,2,2-trichloroethanimidate) | |
Source | CAS Common Chemistry | |
URL | https://commonchemistry.cas.org/detail?cas_rn=74808-10-9 | |
Description | CAS Common Chemistry is an open community resource for accessing chemical information. Nearly 500,000 chemical substances from CAS REGISTRY cover areas of community interest, including common and frequently regulated chemicals, and those relevant to high school and undergraduate chemistry classes. This chemical information, curated by our expert scientists, is provided in alignment with our mission as a division of the American Chemical Society. | |
Explanation | The data from CAS Common Chemistry is provided under a CC-BY-NC 4.0 license, unless otherwise stated. | |
Record name | 2,3,4,6-Tetra-O-acetyl-1-O-(2,2,2-trichloroethanimidoyl)-alpha-D-glucopyranose | |
Source | EPA DSSTox | |
URL | https://comptox.epa.gov/dashboard/DTXSID60447497 | |
Description | DSSTox provides a high quality public chemistry resource for supporting improved predictive toxicology. | |
Retrosynthesis Analysis
AI-Powered Synthesis Planning: Our tool employs the Template_relevance Pistachio, Template_relevance Bkms_metabolic, Template_relevance Pistachio_ringbreaker, Template_relevance Reaxys, Template_relevance Reaxys_biocatalysis model, leveraging a vast database of chemical reactions to predict feasible synthetic routes.
One-Step Synthesis Focus: Specifically designed for one-step synthesis, it provides concise and direct routes for your target compounds, streamlining the synthesis process.
Accurate Predictions: Utilizing the extensive PISTACHIO, BKMS_METABOLIC, PISTACHIO_RINGBREAKER, REAXYS, REAXYS_BIOCATALYSIS database, our tool offers high-accuracy predictions, reflecting the latest in chemical research and data.
Strategy Settings
Precursor scoring | Relevance Heuristic |
---|---|
Min. plausibility | 0.01 |
Model | Template_relevance |
Template Set | Pistachio/Bkms_metabolic/Pistachio_ringbreaker/Reaxys/Reaxys_biocatalysis |
Top-N result to add to graph | 6 |
Feasible Synthetic Routes
Q1: Why is 2,3,4,6-Tetra-O-acetyl-α-D-glucopyranosyl trichloroacetimidate preferred as a glucosyl donor in oligosaccharide synthesis?
A: This compound is a highly effective glucosyl donor due to the trichloroacetimidate group, which readily activates the anomeric carbon upon treatment with a Lewis acid catalyst. This activation facilitates the transfer of the glucosyl moiety to a suitable acceptor molecule (typically an alcohol), forming a new glycosidic linkage. [, , , , ]
Q2: Can you provide an example of a successful application of this compound in the synthesis of a natural product?
A: Certainly. Researchers successfully utilized 2,3,4,6-tetra-O-acetyl-α-D-glucopyranosyl trichloroacetimidate in the final stages of synthesizing kwanzoquinone C, a promising anticancer agent isolated from orange daylilies. The protected glucosyl donor reacted with the aglycon portion of the molecule, and subsequent deprotection steps yielded the target natural product. []
Q3: Are there any limitations to using 2,3,4,6-tetra-O-acetyl-α-D-glucopyranosyl trichloroacetimidate as a glucosyl donor?
A: While highly effective, the compound necessitates the use of a Lewis acid catalyst, which may not be compatible with all substrates or reaction conditions. Additionally, achieving high stereoselectivity in glycosylation reactions can be challenging, especially when the acceptor molecule possesses multiple reactive sites. [, ]
Q4: Are there any alternative glucosyl donors with different reactivity or selectivity profiles?
A: Research has shown that thioglycosides, acting as glycosyl donors, can be advantageous for specific applications, particularly when dealing with deactivated glycosyl acceptors. In contrast, trichloroacetimidates, like the compound in question, were found to be more suitable for deactivated (e.g., fluorine-containing) synthons. [] The choice between different donor types depends on the specific synthetic target and the overall strategy employed.
Haftungsausschluss und Informationen zu In-Vitro-Forschungsprodukten
Bitte beachten Sie, dass alle Artikel und Produktinformationen, die auf BenchChem präsentiert werden, ausschließlich zu Informationszwecken bestimmt sind. Die auf BenchChem zum Kauf angebotenen Produkte sind speziell für In-vitro-Studien konzipiert, die außerhalb lebender Organismen durchgeführt werden. In-vitro-Studien, abgeleitet von dem lateinischen Begriff "in Glas", beinhalten Experimente, die in kontrollierten Laborumgebungen unter Verwendung von Zellen oder Geweben durchgeführt werden. Es ist wichtig zu beachten, dass diese Produkte nicht als Arzneimittel oder Medikamente eingestuft sind und keine Zulassung der FDA für die Vorbeugung, Behandlung oder Heilung von medizinischen Zuständen, Beschwerden oder Krankheiten erhalten haben. Wir müssen betonen, dass jede Form der körperlichen Einführung dieser Produkte in Menschen oder Tiere gesetzlich strikt untersagt ist. Es ist unerlässlich, sich an diese Richtlinien zu halten, um die Einhaltung rechtlicher und ethischer Standards in Forschung und Experiment zu gewährleisten.