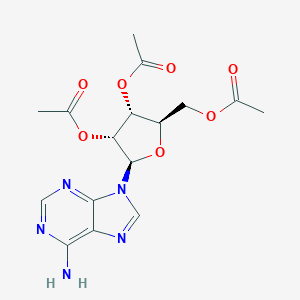
2',3',5'-Tri-O-acetyladenosin
Übersicht
Beschreibung
Adenosine, 2’,3’,5’-triacetate is a derivative of adenosine, a nucleoside composed of adenine and ribose. This compound is characterized by the acetylation of the hydroxyl groups at the 2’, 3’, and 5’ positions of the ribose moiety. The molecular formula of adenosine, 2’,3’,5’-triacetate is C16H19N5O7, and it has a molecular weight of 393.35 g/mol . This compound is often used in biochemical research due to its stability and ability to permeate cell membranes more effectively than adenosine itself.
Wissenschaftliche Forschungsanwendungen
Adenosin, 2’,3’,5’-Triacetat hat eine große Bandbreite an Anwendungen in der wissenschaftlichen Forschung:
Chemie: Es wird als Vorläufer bei der Synthese verschiedener Nukleosid-Analoga und -Derivate verwendet.
Biologie: Die Verbindung wird in Studien zum Nukleosidtransport und -stoffwechsel verwendet.
Medizin: Es dient als Modellverbindung für die Entwicklung von Adenosin-basierten Medikamenten und Therapeutika.
Industrie: Adenosin, 2’,3’,5’-Triacetat wird bei der Herstellung von Nukleosid-Analoga für die antivirale und krebshemmende Forschung verwendet
5. Wirkmechanismus
Der Wirkmechanismus von Adenosin, 2’,3’,5’-Triacetat beinhaltet seine Umwandlung in Adenosin innerhalb der Zelle. Sobald es in der Zelle ist, werden die Acetylgruppen hydrolysiert und Adenosin freigesetzt. Adenosin interagiert dann mit spezifischen Rezeptoren auf der Zelloberfläche, wie z. B. A1, A2A, A2B und A3 Rezeptoren. Diese Interaktionen lösen verschiedene intrazelluläre Signalwege aus, die zu physiologischen Effekten wie Vasodilatation, entzündungshemmenden Reaktionen und Modulation der Neurotransmitterfreisetzung führen .
Wirkmechanismus
Target of Action
2’,3’,5’-Tri-O-acetyladenosine, also known as (2R,3R,4R,5R)-2-(acetoxymethyl)-5-(6-amino-9H-purin-9-yl)tetrahydrofuran-3,4-diyl diacetate, is an adenosine analog . Adenosine analogs are known to target various receptors in the body, including adenosine receptors, which play a crucial role in numerous physiological processes such as vasodilation and inhibition of platelet aggregation .
Mode of Action
As an adenosine analog, 2’,3’,5’-Tri-O-acetyladenosine likely interacts with its targets, the adenosine receptors, by mimicking the action of adenosine. This can result in various physiological changes, depending on the specific type of adenosine receptor that is activated .
Biochemical Pathways
For instance, they can influence the activity of adenylate cyclase, an enzyme that converts ATP to cyclic AMP, a key secondary messenger in many biological processes .
Pharmacokinetics
It’s worth noting that the compound’s solubility in chloroform and methanol may influence its bioavailability and distribution within the body.
Result of Action
The molecular and cellular effects of 2’,3’,5’-Tri-O-acetyladenosine’s action are likely to be diverse, given the wide range of physiological processes that adenosine and its analogs can influence. For example, adenosine analogs have been shown to act as smooth muscle vasodilators , which could lead to a decrease in blood pressure. They have also been shown to inhibit cancer progression .
Vorbereitungsmethoden
Synthesewege und Reaktionsbedingungen: Die Synthese von Adenosin, 2’,3’,5’-Triacetat erfolgt typischerweise durch Acetylierung von Adenosin. Der Prozess beginnt mit der Reaktion von Adenosin mit Essigsäureanhydrid in Gegenwart einer Base wie Pyridin. Die Reaktion wird unter wasserfreien Bedingungen durchgeführt, um die Hydrolyse der Acetylgruppen zu verhindern. Das Produkt wird dann durch Umkristallisation oder Chromatographie gereinigt, um reines Adenosin, 2’,3’,5’-Triacetat zu erhalten .
Industrielle Produktionsmethoden: In der industriellen Produktion von Adenosin, 2’,3’,5’-Triacetat folgt man einem ähnlichen Syntheseweg, jedoch in größerem Maßstab. Die Reaktionsbedingungen werden optimiert, um die Ausbeute und Reinheit zu maximieren. Der Einsatz von automatisierten Reaktoren und Durchflussanlagen kann die Effizienz des Prozesses verbessern. Das Endprodukt wird strengen Qualitätskontrollen unterzogen, um seine Eignung für Forschungs- und pharmazeutische Anwendungen zu gewährleisten .
Analyse Chemischer Reaktionen
Arten von Reaktionen: Adenosin, 2’,3’,5’-Triacetat unterliegt verschiedenen chemischen Reaktionen, darunter:
Hydrolyse: Die Acetylgruppen können unter sauren oder basischen Bedingungen hydrolysiert werden, um Adenosin zu ergeben.
Oxidation: Die Verbindung kann oxidiert werden, um Adenosinderivate mit modifizierten funktionellen Gruppen zu bilden.
Substitution: Die Acetylgruppen können durch andere funktionelle Gruppen durch nucleophile Substitutionsreaktionen substituiert werden.
Häufige Reagenzien und Bedingungen:
Hydrolyse: Natriumhydroxid oder Salzsäure können als Reagenzien verwendet werden.
Oxidation: Kaliumpermanganat oder Wasserstoffperoxid sind übliche Oxidationsmittel.
Substitution: Nucleophile wie Amine oder Thiole können für Substitutionsreaktionen verwendet werden.
Hauptprodukte:
Hydrolyse: Adenosin.
Oxidation: Adenosinderivate mit oxidierten funktionellen Gruppen.
Substitution: Adenosinderivate mit substituierten funktionellen Gruppen.
Vergleich Mit ähnlichen Verbindungen
Adenosin, 2’,3’,5’-Triacetat ist aufgrund seiner acetylierten Struktur einzigartig, die seine Stabilität und Membranpermeabilität im Vergleich zu Adenosin erhöht. Ähnliche Verbindungen umfassen:
Adenosin: Die Stammverbindung, der die Acetylgruppen fehlen.
Inosin, 2’,3’,5’-Triacetat: Eine ähnliche Verbindung mit Inosin anstelle von Adenosin.
Uridin, 2’,3’,5’-Triacetat: Ein weiteres Analog mit Uridin als Nukleosid .
Diese Verbindungen teilen ähnliche Eigenschaften, unterscheiden sich aber in ihren Nukleosidbasen, was zu Variationen in ihren biologischen Aktivitäten und Anwendungen führt.
Eigenschaften
IUPAC Name |
[(2R,3R,4R,5R)-3,4-diacetyloxy-5-(6-aminopurin-9-yl)oxolan-2-yl]methyl acetate | |
---|---|---|
Source | PubChem | |
URL | https://pubchem.ncbi.nlm.nih.gov | |
Description | Data deposited in or computed by PubChem | |
InChI |
InChI=1S/C16H19N5O7/c1-7(22)25-4-10-12(26-8(2)23)13(27-9(3)24)16(28-10)21-6-20-11-14(17)18-5-19-15(11)21/h5-6,10,12-13,16H,4H2,1-3H3,(H2,17,18,19)/t10-,12-,13-,16-/m1/s1 | |
Source | PubChem | |
URL | https://pubchem.ncbi.nlm.nih.gov | |
Description | Data deposited in or computed by PubChem | |
InChI Key |
GCVZNVTXNUTBFB-XNIJJKJLSA-N | |
Source | PubChem | |
URL | https://pubchem.ncbi.nlm.nih.gov | |
Description | Data deposited in or computed by PubChem | |
Canonical SMILES |
CC(=O)OCC1C(C(C(O1)N2C=NC3=C(N=CN=C32)N)OC(=O)C)OC(=O)C | |
Source | PubChem | |
URL | https://pubchem.ncbi.nlm.nih.gov | |
Description | Data deposited in or computed by PubChem | |
Isomeric SMILES |
CC(=O)OC[C@@H]1[C@H]([C@H]([C@@H](O1)N2C=NC3=C(N=CN=C32)N)OC(=O)C)OC(=O)C | |
Source | PubChem | |
URL | https://pubchem.ncbi.nlm.nih.gov | |
Description | Data deposited in or computed by PubChem | |
Molecular Formula |
C16H19N5O7 | |
Source | PubChem | |
URL | https://pubchem.ncbi.nlm.nih.gov | |
Description | Data deposited in or computed by PubChem | |
Molecular Weight |
393.35 g/mol | |
Source | PubChem | |
URL | https://pubchem.ncbi.nlm.nih.gov | |
Description | Data deposited in or computed by PubChem | |
CAS No. |
7387-57-7 | |
Record name | 2',3',5'-Tri-O-acetyladenosine | |
Source | ChemIDplus | |
URL | https://pubchem.ncbi.nlm.nih.gov/substance/?source=chemidplus&sourceid=0007387577 | |
Description | ChemIDplus is a free, web search system that provides access to the structure and nomenclature authority files used for the identification of chemical substances cited in National Library of Medicine (NLM) databases, including the TOXNET system. | |
Retrosynthesis Analysis
AI-Powered Synthesis Planning: Our tool employs the Template_relevance Pistachio, Template_relevance Bkms_metabolic, Template_relevance Pistachio_ringbreaker, Template_relevance Reaxys, Template_relevance Reaxys_biocatalysis model, leveraging a vast database of chemical reactions to predict feasible synthetic routes.
One-Step Synthesis Focus: Specifically designed for one-step synthesis, it provides concise and direct routes for your target compounds, streamlining the synthesis process.
Accurate Predictions: Utilizing the extensive PISTACHIO, BKMS_METABOLIC, PISTACHIO_RINGBREAKER, REAXYS, REAXYS_BIOCATALYSIS database, our tool offers high-accuracy predictions, reflecting the latest in chemical research and data.
Strategy Settings
Precursor scoring | Relevance Heuristic |
---|---|
Min. plausibility | 0.01 |
Model | Template_relevance |
Template Set | Pistachio/Bkms_metabolic/Pistachio_ringbreaker/Reaxys/Reaxys_biocatalysis |
Top-N result to add to graph | 6 |
Feasible Synthetic Routes
Haftungsausschluss und Informationen zu In-Vitro-Forschungsprodukten
Bitte beachten Sie, dass alle Artikel und Produktinformationen, die auf BenchChem präsentiert werden, ausschließlich zu Informationszwecken bestimmt sind. Die auf BenchChem zum Kauf angebotenen Produkte sind speziell für In-vitro-Studien konzipiert, die außerhalb lebender Organismen durchgeführt werden. In-vitro-Studien, abgeleitet von dem lateinischen Begriff "in Glas", beinhalten Experimente, die in kontrollierten Laborumgebungen unter Verwendung von Zellen oder Geweben durchgeführt werden. Es ist wichtig zu beachten, dass diese Produkte nicht als Arzneimittel oder Medikamente eingestuft sind und keine Zulassung der FDA für die Vorbeugung, Behandlung oder Heilung von medizinischen Zuständen, Beschwerden oder Krankheiten erhalten haben. Wir müssen betonen, dass jede Form der körperlichen Einführung dieser Produkte in Menschen oder Tiere gesetzlich strikt untersagt ist. Es ist unerlässlich, sich an diese Richtlinien zu halten, um die Einhaltung rechtlicher und ethischer Standards in Forschung und Experiment zu gewährleisten.