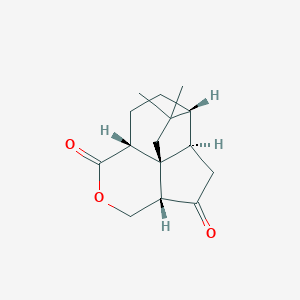
Quadrone
Übersicht
Beschreibung
A Quadrone is a cube-shaped construct with four arms and four wings . It is one of the base modrons, the mechanical inhabitants of the plane of Mechanus . Quadrones have six sides, each with a sensory organ that grants it exceptional vision in various spectrums .
Synthesis Analysis
The synthesis of Quadrone has been documented in several studies . For instance, the total synthesis of Quadrone was performed by Steven D. Burke in 1982 . The process involved 21 linear steps, including various chemical reactions such as the Claisen Rearrangement and Jones Oxidation . Another total synthesis was performed by Samuel J. Danishefsky in 1981 .
Chemical Reactions Analysis
The chemical reactions involved in the synthesis of Quadrone have been described in the studies by Burke and Danishefsky . These reactions include the Claisen Rearrangement, Jones Oxidation, Michael Addition, and others .
Wissenschaftliche Forschungsanwendungen
Synthesis of Natural Quadrone
Quadrone has been synthesized in its natural form starting from the ammonium salt of (-)-10-camphorsulfonic acid . This synthesis provides direct evidence for its absolute configuration . The interesting cytotoxic properties and the challenging structural features of these novel sesquiterpenes have elicited extensive synthetic activity .
Metabolite of Aspergillus terreus
Quadrone is a metabolite isolated from Aspergillus terreus . The structural features of these novel sesquiterpenes have elicited extensive synthetic activity .
Cytotoxic Properties
Quadrone has interesting cytotoxic properties . These properties have led to extensive synthetic activity and a large number of elegant total syntheses have been achieved .
Unmanned Aerial Vehicle (UAV) Development
Quadrone is used in the development of remotely controlled UAVs . The Quadrone is controlled with the help of a graphical user interface (GUI) where the communication between GUI and Quadrone is made by using wireless communication system .
Traffic Control and Surveillance
Quadrone has potential use in traffic control and its surveillance . It can minimize traffic accidents by remotely searching wide areas without the risks of traditional manned aircraft .
Aerial Photography
Quadrone is used in aerial photography . It is an unpiloted aircraft which can be remotely controlled and is often used in civil applications such as aerial photography .
Safety and Hazards
Safety data for Quadrone suggests that dust formation should be avoided and contact with skin and eyes should be prevented . It is recommended to use personal protective equipment, ensure adequate ventilation, and remove all sources of ignition . In case of accidental release, personnel should be evacuated to safe areas .
Wirkmechanismus
Target of Action
Quadrone is a sesquiterpene originally isolated from the fungus Aspergillus terreus . It has been found to be cytotoxic to human carcinoma KB cells in vitro . This suggests that the primary targets of Quadrone are likely to be cellular components or processes that are crucial for the survival and proliferation of these cancer cells.
Mode of Action
Its cytotoxicity suggests that it may interact with its targets in a way that disrupts normal cellular functions, leading to cell death
Biochemical Pathways
The biosynthesis of Quadrone involves a multistep carbocation rearrangement , but the downstream effects of this process on other biochemical pathways remain to be elucidated.
Result of Action
Quadrone has been found to be cytotoxic to human carcinoma KB cells in vitro . This suggests that the molecular and cellular effects of Quadrone’s action include disruption of normal cellular functions and induction of cell death in these cancer cells.
Eigenschaften
IUPAC Name |
13,13-dimethyl-7-oxatetracyclo[7.5.0.01,5.02,12]tetradecane-4,8-dione | |
---|---|---|
Source | PubChem | |
URL | https://pubchem.ncbi.nlm.nih.gov | |
Description | Data deposited in or computed by PubChem | |
InChI |
InChI=1S/C15H20O3/c1-14(2)7-15-9-4-3-8(14)10(15)5-12(16)11(15)6-18-13(9)17/h8-11H,3-7H2,1-2H3 | |
Source | PubChem | |
URL | https://pubchem.ncbi.nlm.nih.gov | |
Description | Data deposited in or computed by PubChem | |
InChI Key |
BBIDMUQZCCGABN-UHFFFAOYSA-N | |
Source | PubChem | |
URL | https://pubchem.ncbi.nlm.nih.gov | |
Description | Data deposited in or computed by PubChem | |
Canonical SMILES |
CC1(CC23C4CCC1C2CC(=O)C3COC4=O)C | |
Source | PubChem | |
URL | https://pubchem.ncbi.nlm.nih.gov | |
Description | Data deposited in or computed by PubChem | |
Molecular Formula |
C15H20O3 | |
Source | PubChem | |
URL | https://pubchem.ncbi.nlm.nih.gov | |
Description | Data deposited in or computed by PubChem | |
Molecular Weight |
248.32 g/mol | |
Source | PubChem | |
URL | https://pubchem.ncbi.nlm.nih.gov | |
Description | Data deposited in or computed by PubChem | |
Product Name |
CID 197204 | |
CAS RN |
66550-08-1, 87480-01-1 | |
Record name | (3aS,5aR,6R,8aR,8bR)-Octahydro-10,10-dimethyl-6,8b-ethano-8bH-cyclopenta[de]-2-benzopyran-1,4-dione | |
Source | CAS Common Chemistry | |
URL | https://commonchemistry.cas.org/detail?cas_rn=66550-08-1 | |
Description | CAS Common Chemistry is an open community resource for accessing chemical information. Nearly 500,000 chemical substances from CAS REGISTRY cover areas of community interest, including common and frequently regulated chemicals, and those relevant to high school and undergraduate chemistry classes. This chemical information, curated by our expert scientists, is provided in alignment with our mission as a division of the American Chemical Society. | |
Explanation | The data from CAS Common Chemistry is provided under a CC-BY-NC 4.0 license, unless otherwise stated. | |
Record name | Quadrone | |
Source | DTP/NCI | |
URL | https://dtp.cancer.gov/dtpstandard/servlet/dwindex?searchtype=NSC&outputformat=html&searchlist=284437 | |
Description | The NCI Development Therapeutics Program (DTP) provides services and resources to the academic and private-sector research communities worldwide to facilitate the discovery and development of new cancer therapeutic agents. | |
Explanation | Unless otherwise indicated, all text within NCI products is free of copyright and may be reused without our permission. Credit the National Cancer Institute as the source. | |
Retrosynthesis Analysis
AI-Powered Synthesis Planning: Our tool employs the Template_relevance Pistachio, Template_relevance Bkms_metabolic, Template_relevance Pistachio_ringbreaker, Template_relevance Reaxys, Template_relevance Reaxys_biocatalysis model, leveraging a vast database of chemical reactions to predict feasible synthetic routes.
One-Step Synthesis Focus: Specifically designed for one-step synthesis, it provides concise and direct routes for your target compounds, streamlining the synthesis process.
Accurate Predictions: Utilizing the extensive PISTACHIO, BKMS_METABOLIC, PISTACHIO_RINGBREAKER, REAXYS, REAXYS_BIOCATALYSIS database, our tool offers high-accuracy predictions, reflecting the latest in chemical research and data.
Strategy Settings
Precursor scoring | Relevance Heuristic |
---|---|
Min. plausibility | 0.01 |
Model | Template_relevance |
Template Set | Pistachio/Bkms_metabolic/Pistachio_ringbreaker/Reaxys/Reaxys_biocatalysis |
Top-N result to add to graph | 6 |
Feasible Synthetic Routes
Haftungsausschluss und Informationen zu In-Vitro-Forschungsprodukten
Bitte beachten Sie, dass alle Artikel und Produktinformationen, die auf BenchChem präsentiert werden, ausschließlich zu Informationszwecken bestimmt sind. Die auf BenchChem zum Kauf angebotenen Produkte sind speziell für In-vitro-Studien konzipiert, die außerhalb lebender Organismen durchgeführt werden. In-vitro-Studien, abgeleitet von dem lateinischen Begriff "in Glas", beinhalten Experimente, die in kontrollierten Laborumgebungen unter Verwendung von Zellen oder Geweben durchgeführt werden. Es ist wichtig zu beachten, dass diese Produkte nicht als Arzneimittel oder Medikamente eingestuft sind und keine Zulassung der FDA für die Vorbeugung, Behandlung oder Heilung von medizinischen Zuständen, Beschwerden oder Krankheiten erhalten haben. Wir müssen betonen, dass jede Form der körperlichen Einführung dieser Produkte in Menschen oder Tiere gesetzlich strikt untersagt ist. Es ist unerlässlich, sich an diese Richtlinien zu halten, um die Einhaltung rechtlicher und ethischer Standards in Forschung und Experiment zu gewährleisten.