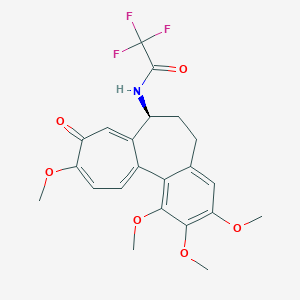
N-Trifluoroacetyl Deacetylcolchicine
Übersicht
Beschreibung
Colchicine, 17,17,17-trifluoro- is a synthetic derivative of colchicine, a naturally occurring alkaloid extracted from plants such as Colchicum autumnale (autumn crocus) and Gloriosa superba (glory lily). This compound is known for its potent biological activities, particularly its ability to disrupt microtubule formation, making it valuable in various scientific and medical applications .
Vorbereitungsmethoden
Synthetic Routes and Reaction Conditions
The synthesis of colchicine, 17,17,17-trifluoro-, involves the introduction of trifluoromethyl groups into the colchicine molecule. One common method includes the use of trifluoromethylation reagents such as trifluoromethyl iodide or trifluoromethyl sulfonates in the presence of a base like potassium carbonate.
Industrial Production Methods
Industrial production of colchicine, 17,17,17-trifluoro-, follows similar synthetic routes but on a larger scale. The process involves optimizing reaction conditions to ensure high yield and purity. Techniques such as continuous flow reactors and automated synthesis systems are employed to enhance efficiency and scalability. Purification steps, including crystallization and chromatography, are crucial to obtain the final product with the desired specifications .
Analyse Chemischer Reaktionen
Types of Reactions
Colchicine, 17,17,17-trifluoro-, undergoes various chemical reactions, including:
Oxidation: This reaction can be facilitated by oxidizing agents such as hydrogen peroxide or potassium permanganate, leading to the formation of oxidized derivatives.
Reduction: Reduction reactions involve the use of reducing agents like sodium borohydride or lithium aluminum hydride to produce reduced forms of the compound.
Common Reagents and Conditions
Oxidation: Hydrogen peroxide, potassium permanganate; typically conducted in aqueous or organic solvents at moderate temperatures.
Reduction: Sodium borohydride, lithium aluminum hydride; reactions are usually carried out in anhydrous solvents under inert atmosphere.
Substitution: Nucleophiles such as amines or thiols; reactions often require a base and are performed in polar aprotic solvents.
Major Products Formed
The major products formed from these reactions include various oxidized, reduced, and substituted derivatives of colchicine, 17,17,17-trifluoro-. These derivatives can exhibit different biological activities and properties, making them useful for further research and development .
Wissenschaftliche Forschungsanwendungen
Introduction to N-Trifluoroacetyl Deacetylcolchicine
This compound is a derivative of colchicine, a well-known alkaloid primarily used for its anti-inflammatory properties and as a treatment for gout. This compound has gained attention in various scientific research applications due to its unique chemical structure and biological activity.
Vascular Disruption and Cancer Treatment
This compound has been studied for its potential as a vascular disrupting agent (VDA). VDAs are compounds that target and disrupt the blood supply to tumors, leading to tumor cell death. Research indicates that colchicine and its derivatives can destabilize microtubules, which is crucial in preventing the formation of the mitotic spindle during cell division. This property is being explored in the development of targeted therapies for cancer treatment.
- Case Study : A study demonstrated that deacetylcolchicine labeled with technetium-99m showed significant tumor targeting properties in a breast cancer model, suggesting that similar derivatives could enhance imaging and therapeutic efficacy in oncology .
Analytical Chemistry
This compound is utilized in analytical methods for drug development, particularly in the pharmaceutical industry. Its role includes:
- Quality Control (QC) : Used as a reference standard in the validation of analytical methods.
- Method Development : Assists in developing new methods for the quantification of colchicine and its analogs in various formulations.
This application is crucial during the Abbreviated New Drug Application (ANDA) process, where accurate measurement and validation of drug components are required .
Drug Delivery Systems
Research has indicated that this compound can be incorporated into nanoparticle formulations for drug delivery. The compound's ability to inhibit microtubule polymerization makes it an attractive candidate for targeting cancer cells while minimizing side effects on healthy tissues.
- Case Study : Nanoparticle formulations containing colchicine derivatives have shown enhanced therapeutic effects against resistant cancer cell lines, highlighting their potential in overcoming drug resistance .
Immunomodulatory Effects
Colchicine derivatives, including this compound, have been investigated for their immunomodulatory effects. They can modulate immune responses by inhibiting neutrophil migration and cytokine release, making them potential candidates for treating autoimmune diseases.
- Research Findings : Studies have shown that colchicine can reduce inflammation in conditions such as familial Mediterranean fever and Behçet's disease, indicating that its derivatives may also provide similar benefits .
Comparative Analysis Table
Wirkmechanismus
The primary mechanism of action of colchicine, 17,17,17-trifluoro-, involves binding to tubulin, a protein that forms microtubules. By binding to tubulin, the compound prevents the polymerization of microtubules, leading to the disruption of the cytoskeleton and inhibition of cell division. This mechanism is particularly effective in rapidly dividing cells, such as cancer cells. Additionally, colchicine, 17,17,17-trifluoro-, can modulate inflammatory pathways by inhibiting the activation of the inflammasome complex in neutrophils and monocytes .
Vergleich Mit ähnlichen Verbindungen
Colchicine, 17,17,17-trifluoro-, can be compared with other colchicine derivatives and microtubule inhibitors:
Colchicine: The parent compound, known for its anti-inflammatory and anticancer properties.
Thiocolchicine: A sulfur-containing derivative with similar biological activities but different pharmacokinetic properties.
Combretastatin: Another microtubule inhibitor with a different chemical structure but similar mechanism of action.
Vinca Alkaloids: A class of compounds that also target microtubules but have distinct chemical structures and clinical applications .
Conclusion
Colchicine, 17,17,17-trifluoro-, is a valuable compound in scientific research and medicine due to its potent biological activities and versatile applications. Its ability to disrupt microtubule formation and modulate inflammatory pathways makes it a promising candidate for further investigation and development in various fields.
Biologische Aktivität
N-Trifluoroacetyl Deacetylcolchicine is a synthetic derivative of deacetylcolchicine, an active metabolite of the natural alkaloid colchicine, which is derived from the plant Colchicum autumnale. This compound has garnered attention in the field of cancer research due to its significant biological activities, particularly its ability to inhibit microtubule polymerization.
Chemical Structure and Properties
This compound features a trifluoroacetyl group that enhances its solubility and potentially its biological activity. The presence of three fluorine atoms in the trifluoroacetyl moiety contributes to its unique chemical properties, making it a valuable tool for researchers studying cellular processes.
The primary mechanism of action for this compound involves its binding to tubulin, a key protein in microtubule formation. By binding to the colchicine-binding site on tubulin, this compound prevents tubulin polymerization into microtubules, leading to disruption in mitotic spindle formation and ultimately inducing apoptosis in rapidly dividing cells. This action is crucial for its potential as an anticancer agent.
Key Mechanisms:
- Inhibition of Microtubule Polymerization : Prevents the assembly of microtubules essential for cell division.
- Induction of Apoptosis : Triggers programmed cell death in cancer cells.
- Activation of Signaling Pathways : Stimulates pathways such as JNK/SAPK, which are involved in cellular stress responses.
Biological Activity
Research has demonstrated that this compound exhibits potent antitumor activity across various cancer cell lines. Its ability to induce cell death and arrest cell division positions it as a promising candidate for cancer therapy.
Comparative Biological Activity Table
Compound Name | Mechanism of Action | Key Features |
---|---|---|
This compound | Inhibits microtubule polymerization | Enhanced solubility and bioavailability |
Colchicine | Disrupts microtubule assembly | Well-known natural alkaloid |
Demecolcine | Similar mechanism with reduced toxicity | Methylated derivative of colchicine |
N-Glutaryl Deacetylcolchicine | Enhanced targeting capabilities | Glycoconjugate with improved specificity |
Case Studies and Research Findings
- Antitumor Efficacy : In vitro studies have shown that this compound can effectively induce apoptosis in various cancer cell lines, including breast and lung cancers. For instance, one study demonstrated a significant reduction in cell viability in treated cancer cells compared to controls, indicating its potential therapeutic efficacy .
- Microtubule Disruption Studies : Experimental assays utilizing live-cell imaging revealed that this compound disrupts normal microtubule dynamics, leading to mitotic arrest. This was evidenced by the accumulation of cells in the G2/M phase of the cell cycle, highlighting its role as an antimitotic agent .
- Pharmacokinetics and Toxicity : Preliminary pharmacokinetic studies suggest that the trifluoroacetyl modification may enhance the compound's bioavailability compared to traditional colchicine derivatives. However, further investigations are necessary to fully assess its safety profile and potential toxic effects at varying dosages .
Eigenschaften
IUPAC Name |
2,2,2-trifluoro-N-[(7S)-1,2,3,10-tetramethoxy-9-oxo-6,7-dihydro-5H-benzo[a]heptalen-7-yl]acetamide | |
---|---|---|
Source | PubChem | |
URL | https://pubchem.ncbi.nlm.nih.gov | |
Description | Data deposited in or computed by PubChem | |
InChI |
InChI=1S/C22H22F3NO6/c1-29-16-8-6-12-13(10-15(16)27)14(26-21(28)22(23,24)25)7-5-11-9-17(30-2)19(31-3)20(32-4)18(11)12/h6,8-10,14H,5,7H2,1-4H3,(H,26,28)/t14-/m0/s1 | |
Source | PubChem | |
URL | https://pubchem.ncbi.nlm.nih.gov | |
Description | Data deposited in or computed by PubChem | |
InChI Key |
MCHADYPYCJQLMV-AWEZNQCLSA-N | |
Source | PubChem | |
URL | https://pubchem.ncbi.nlm.nih.gov | |
Description | Data deposited in or computed by PubChem | |
Canonical SMILES |
COC1=CC=C2C(=CC1=O)C(CCC3=CC(=C(C(=C32)OC)OC)OC)NC(=O)C(F)(F)F | |
Source | PubChem | |
URL | https://pubchem.ncbi.nlm.nih.gov | |
Description | Data deposited in or computed by PubChem | |
Isomeric SMILES |
COC1=CC=C2C(=CC1=O)[C@H](CCC3=CC(=C(C(=C32)OC)OC)OC)NC(=O)C(F)(F)F | |
Source | PubChem | |
URL | https://pubchem.ncbi.nlm.nih.gov | |
Description | Data deposited in or computed by PubChem | |
Molecular Formula |
C22H22F3NO6 | |
Source | PubChem | |
URL | https://pubchem.ncbi.nlm.nih.gov | |
Description | Data deposited in or computed by PubChem | |
DSSTOX Substance ID |
DTXSID90180825 | |
Record name | Colchicine, 17,17,17-trifluoro- | |
Source | EPA DSSTox | |
URL | https://comptox.epa.gov/dashboard/DTXSID90180825 | |
Description | DSSTox provides a high quality public chemistry resource for supporting improved predictive toxicology. | |
Molecular Weight |
453.4 g/mol | |
Source | PubChem | |
URL | https://pubchem.ncbi.nlm.nih.gov | |
Description | Data deposited in or computed by PubChem | |
CAS No. |
26195-65-3 | |
Record name | Colchicine, 17,17,17-trifluoro- | |
Source | ChemIDplus | |
URL | https://pubchem.ncbi.nlm.nih.gov/substance/?source=chemidplus&sourceid=0026195653 | |
Description | ChemIDplus is a free, web search system that provides access to the structure and nomenclature authority files used for the identification of chemical substances cited in National Library of Medicine (NLM) databases, including the TOXNET system. | |
Record name | NSC305987 | |
Source | DTP/NCI | |
URL | https://dtp.cancer.gov/dtpstandard/servlet/dwindex?searchtype=NSC&outputformat=html&searchlist=305987 | |
Description | The NCI Development Therapeutics Program (DTP) provides services and resources to the academic and private-sector research communities worldwide to facilitate the discovery and development of new cancer therapeutic agents. | |
Explanation | Unless otherwise indicated, all text within NCI products is free of copyright and may be reused without our permission. Credit the National Cancer Institute as the source. | |
Record name | Colchicine, 17,17,17-trifluoro- | |
Source | EPA DSSTox | |
URL | https://comptox.epa.gov/dashboard/DTXSID90180825 | |
Description | DSSTox provides a high quality public chemistry resource for supporting improved predictive toxicology. | |
Retrosynthesis Analysis
AI-Powered Synthesis Planning: Our tool employs the Template_relevance Pistachio, Template_relevance Bkms_metabolic, Template_relevance Pistachio_ringbreaker, Template_relevance Reaxys, Template_relevance Reaxys_biocatalysis model, leveraging a vast database of chemical reactions to predict feasible synthetic routes.
One-Step Synthesis Focus: Specifically designed for one-step synthesis, it provides concise and direct routes for your target compounds, streamlining the synthesis process.
Accurate Predictions: Utilizing the extensive PISTACHIO, BKMS_METABOLIC, PISTACHIO_RINGBREAKER, REAXYS, REAXYS_BIOCATALYSIS database, our tool offers high-accuracy predictions, reflecting the latest in chemical research and data.
Strategy Settings
Precursor scoring | Relevance Heuristic |
---|---|
Min. plausibility | 0.01 |
Model | Template_relevance |
Template Set | Pistachio/Bkms_metabolic/Pistachio_ringbreaker/Reaxys/Reaxys_biocatalysis |
Top-N result to add to graph | 6 |
Feasible Synthetic Routes
Haftungsausschluss und Informationen zu In-Vitro-Forschungsprodukten
Bitte beachten Sie, dass alle Artikel und Produktinformationen, die auf BenchChem präsentiert werden, ausschließlich zu Informationszwecken bestimmt sind. Die auf BenchChem zum Kauf angebotenen Produkte sind speziell für In-vitro-Studien konzipiert, die außerhalb lebender Organismen durchgeführt werden. In-vitro-Studien, abgeleitet von dem lateinischen Begriff "in Glas", beinhalten Experimente, die in kontrollierten Laborumgebungen unter Verwendung von Zellen oder Geweben durchgeführt werden. Es ist wichtig zu beachten, dass diese Produkte nicht als Arzneimittel oder Medikamente eingestuft sind und keine Zulassung der FDA für die Vorbeugung, Behandlung oder Heilung von medizinischen Zuständen, Beschwerden oder Krankheiten erhalten haben. Wir müssen betonen, dass jede Form der körperlichen Einführung dieser Produkte in Menschen oder Tiere gesetzlich strikt untersagt ist. Es ist unerlässlich, sich an diese Richtlinien zu halten, um die Einhaltung rechtlicher und ethischer Standards in Forschung und Experiment zu gewährleisten.