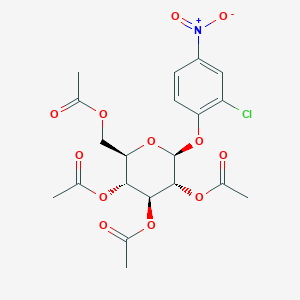
2-Chloro-4-nitrophenyl-2,3,4,6-tetra-O-acetyl-beta-D-glucopyranoside
Übersicht
Beschreibung
2-Chloro-4-nitrophenyl-2,3,4,6-tetra-O-acetyl-β-D-glucopyranoside (CAS 35023-71-3) is a chromogenic substrate widely used in enzymology and carbohydrate chemistry. Its structure features a β-D-glucopyranose core with acetyl protecting groups at the 2, 3, 4, and 6 positions, and a 2-chloro-4-nitrophenyl aglycone. The acetyl groups enhance stability during synthesis and storage, while the chloro-nitrophenyl moiety acts as a chromophore, releasing a yellow product (λmax ~400–420 nm) upon enzymatic hydrolysis by β-glucosidases . This compound is pivotal in high-throughput screening for enzyme inhibitors and activators, particularly in drug discovery targeting lysosomal storage disorders and glycosidase-related pathologies .
Vorbereitungsmethoden
Phase Transfer Glycosylation
Glycosyl Donor Preparation
The synthesis typically begins with the preparation of 2,3,4,6-tetra-O-acetyl-β-D-glucopyranosyl chloride (3a/b ), derived from peracetylated glucose via treatment with acetyl chloride in methanol under argon . This step ensures the preservation of the β-anomeric configuration, critical for subsequent glycosylation. The glycosyl chloride is purified via silica gel chromatography (heptane/acetone, 3:7) to achieve >95% purity, as confirmed by thin-layer chromatography (TLC) .
Coupling with 2-Chloro-4-nitrophenol
Phase transfer conditions using tetrabutylammonium bromide (TBAB) as a catalyst and sodium hydroxide as a base in dichloromethane at 20°C for 18 hours facilitate the stereoselective glycosylation . The reaction proceeds via an SN2 mechanism, yielding the target compound with 89% efficiency . Key parameters include:
Parameter | Optimal Value | Impact on Yield |
---|---|---|
Temperature | 20°C | Maximizes SN2 pathway |
Reaction Time | 18 hours | Completes anion exchange |
Molar Ratio (Donor:Acceptor) | 1:1.2 | Minimizes side products |
The crude product is purified via column chromatography (ethyl acetate/hexane, 1:3), yielding a crystalline solid with a melting point of 144–145°C .
Direct Glycosylation with Activated Donors
Trichloroacetimidate Approach
An alternative method employs 2,3,4,6-tetra-O-acetyl-β-D-glucopyranosyl trichloroacetimidate as the donor, activated by trimethylsilyl triflate (TMSOTf) in anhydrous dichloromethane . This method achieves 78% yield but requires stringent anhydrous conditions to prevent hydrolysis. The β-selectivity arises from the participating acetyl groups, which stabilize the oxocarbenium ion intermediate .
Silver Triflate-Mediated Coupling
Silver triflate (AgOTf) catalyzes the glycosylation of peracetylated glucose with 2-chloro-4-nitrophenol in dichloromethane at −15°C . This low-temperature protocol suppresses side reactions, achieving 85% yield with >20:1 β/α selectivity .
Protecting Group Strategies
Acetylation-Deprotection Sequences
The tetra-O-acetyl protecting groups are introduced via reaction with acetic anhydride in pyridine, achieving full substitution at the 2, 3, 4, and 6 positions . Post-glycosylation deprotection is performed using Zemplén conditions (NaOMe/MeOH), though this step is unnecessary for the acetylated target compound .
Stability of Protecting Groups
The acetyl groups remain intact under phase transfer conditions (pH 10–12), as demonstrated by NMR monitoring . However, prolonged exposure to strong bases (>24 hours) may lead to partial deacetylation at the C-6 position .
Reaction Optimization and Scalability
Solvent Effects
Dichloromethane outperforms THF and acetonitrile in glycosylation efficiency due to its low polarity, which stabilizes the glycosyl oxocarbenium ion . A comparative study reveals:
Solvent | Yield (%) | β/α Ratio |
---|---|---|
Dichloromethane | 89 | 20:1 |
Acetonitrile | 62 | 12:1 |
THF | 45 | 8:1 |
Analytical Characterization
Spectroscopic Validation
-
1H NMR (CDCl3): δ 5.21 (t, J = 9.6 Hz, H-3), 5.08 (t, J = 9.4 Hz, H-4), 4.93 (d, J = 8.1 Hz, H-1), confirming β-configuration .
-
13C NMR : δ 170.2–169.4 (acetyl carbonyls), 99.3 (C-1), 72.3 (C-5) .
Crystallographic Analysis
Single-crystal X-ray diffraction confirms the β-anomeric configuration and tetra-O-acetyl substitution pattern. The nitro group adopts a coplanar orientation with the phenyl ring, minimizing steric hindrance .
Analyse Chemischer Reaktionen
Reaktionstypen
TBTA unterliegt hauptsächlich Koordinationsreaktionen mit Kupfer(I)-Ionen und bildet stabile Komplexe, die Azid-Alkin-Cycloadditionsreaktionen erleichtern. Diese Reaktionen sind hochspezifisch und effizient, was TBTA zu einem idealen Liganden für die Klickchemie macht .
Häufige Reagenzien und Bedingungen
Kupfer(I)-Ionen: Stabilisiert durch TBTA, um Oxidation und Disproportionierung zu verhindern.
Azide und Alkine: Reaktanten in der Klickreaktion.
Natriumascorbat: Reduktionsmittel zur in situ-Erzeugung von Kupfer(I)-Ionen.
Acetonitril: Lösungsmittel für die Reaktion.
Hauptprodukte
Das Hauptprodukt der durch TBTA katalysierten Klickreaktion ist ein 1,2,3-Triazolderivat. Dieses Produkt entsteht durch die regioselektive Cycloaddition von Aziden und Alkinen .
Wissenschaftliche Forschungsanwendungen
Chromogenic Enzyme Substrate
One of the primary applications of 2-Chloro-4-nitrophenyl-2,3,4,6-tetra-O-acetyl-beta-D-glucopyranoside is as a chromogenic substrate for enzymes. It is particularly useful in the characterization of glycosidases, which are enzymes that hydrolyze glycosidic bonds in carbohydrates. The compound undergoes hydrolysis to release a colored product that can be quantitatively measured, making it an excellent tool for enzyme activity assays .
Glycobiology Studies
In glycobiology, this compound serves as a valuable tool for studying glycan interactions and metabolism. Researchers utilize it to investigate the roles of specific glycosidases in biological processes, including cell signaling and pathogen recognition . Its ability to mimic natural substrates allows for the exploration of enzyme specificity and kinetics.
Drug Development
The compound's structure allows it to be employed in drug discovery processes. By modifying the glucopyranoside structure or its substituents, researchers can create analogs that may exhibit enhanced bioactivity or selectivity against specific targets. This application is particularly relevant in the development of antiviral and anticancer agents where carbohydrate recognition plays a crucial role .
Bioconjugation Techniques
This compound is also used in bioconjugation strategies where it can be linked to proteins or other biomolecules. This facilitates the study of protein function and interactions through labeling techniques that allow for visualization and tracking within biological systems .
Case Studies
Wirkmechanismus
TBTA exerts its effects by stabilizing copper(I) ions, which are essential for the catalysis of azide-alkyne cycloaddition reactions. The ligand forms a complex with copper(I), preventing its oxidation and disproportionation. This stabilization allows the catalytic cycle of the click reaction to proceed efficiently, resulting in the formation of 1,2,3-triazole derivatives .
Vergleich Mit ähnlichen Verbindungen
Comparison with Structurally Similar Compounds
Nitrophenyl Derivatives
4-Nitrophenyl 2-acetamido-3,6-di-O-acetyl-2-deoxy-4-O-(2,3,4,6-tetra-O-acetyl-β-D-galactopyranosyl)-β-D-glucopyranoside (4c)
- Structure : Contains a 4-nitrophenyl group and a disaccharide core (glucose-galactose) with acetyl and acetamido substituents.
- Enzyme Specificity: Targets α/β-glucosidases and galactosidases due to the galactopyranosyl unit.
- Applications : Used in glycosylation studies and as a model for oligosaccharide synthesis.
- Synthesis: Yield of 45% via Koenigs-Knorr glycosylation, with TLC RF = 0.52 .
- Key Difference: The disaccharide structure and acetamido group reduce hydrolysis rates compared to the monosaccharide-based target compound, limiting its utility in rapid enzyme assays .
2-Nitrophenyl Analog (4d)
- Structure : Ortho-nitrophenyl substitution instead of para.
- Performance : Lower enzymatic hydrolysis efficiency due to steric hindrance from the ortho-nitro group, yielding 44% in synthesis .
- Detection Sensitivity : Releases a less intense chromophore (λmax shifts), making it less favorable for spectrophotometric assays compared to the target compound .
Anomeric Variants
2-Chloro-4-nitrophenyl-2,3,4,6-tetra-O-acetyl-α-D-glucopyranoside
- Structure: α-anomeric configuration.
- Enzyme Interaction : Poor substrate for β-glucosidases but may interact with α-glucosidases.
- Applications: Limited to specialized studies on α-glucosidase mechanisms. Priced at $300/100 mg, reflecting niche demand .
Acylated Glucopyranosides with Alternative Aryl Groups
Guaiacol-2,3,4,6-tetra-O-acetyl-β-D-glucopyranoside
- Structure: Guaiacol (2-methoxyphenol) aglycone.
- Applications : Intermediate in synthesizing guaiacol conjugates for studying smoke-tainted wine fermentation. Lacks chromogenic utility but valuable in natural product research .
4-Formylphenyl Derivative
- Structure : 4-formylphenyl group.
- Reactivity : The formyl group enables further chemical modifications (e.g., Schiff base formation), making it a versatile intermediate for pharmaceutical conjugates .
Alkyl and Aromatic Esters
2'-Bromoethyl 2,3,4,6-Tetra-O-acetyl-β-D-glucopyranoside
- Structure : Bromoethyl group as a leaving group.
- Applications: Primarily a glycosyl donor in synthesis. The bromine atom facilitates nucleophilic displacement reactions, unlike the target compound’s focus on enzymatic hydrolysis .
Methyl 6-O-(4-nitrobenzoyl)-2,3,4-tri-O-octanoyl-α-D-glucopyranoside (6)
- Structure: Long-chain acyl groups (octanoyl) and a nitrobenzoyl group.
- Properties : Enhanced lipophilicity improves membrane permeability, useful in cellular uptake studies. However, bulky acyl chains reduce enzymatic hydrolysis rates .
Data Tables
Table 1: Structural and Functional Comparison
Critical Analysis
- Chromogenic Efficiency: The target compound’s para-nitro group and β-configuration maximize hydrolysis rates and chromophore intensity, outperforming ortho-substituted (4d) and non-nitrophenyl analogs .
- Synthetic Flexibility : Compounds like the 4-formylphenyl derivative and bromoethyl analog prioritize chemical reactivity over enzymatic utility, highlighting a trade-off between assay compatibility and synthetic versatility.
- Commercial Viability : The target compound’s availability from suppliers like Glentham Life Sciences underscores its established role in research, whereas niche analogs (e.g., guaiacol derivative ) remain specialized.
Biologische Aktivität
2-Chloro-4-nitrophenyl-2,3,4,6-tetra-O-acetyl-beta-D-glucopyranoside (CAS No. 35023-71-3) is a synthetic compound that exhibits a range of biological activities. Its structure consists of a glucopyranoside moiety with multiple acetyl groups and a chloro-nitrophenyl substituent, which contributes to its reactivity and potential applications in biochemical research and medicinal chemistry.
- Molecular Formula : CHClN O
- Molecular Weight : 503.84 g/mol
- Solubility : Soluble in organic solvents; limited solubility in water.
Biological Activity Overview
The biological activities of this compound can be categorized into several key areas based on existing research:
-
Enzyme Inhibition
- This compound has been studied for its inhibitory effects on various glycosidases. The presence of the acetyl groups enhances its interaction with the active sites of these enzymes, making it a useful tool for studying carbohydrate metabolism.
-
Antimicrobial Properties
- Preliminary studies suggest that this compound exhibits antimicrobial activity against certain bacterial strains. The nitrophenyl group is known to contribute to such activities by disrupting bacterial cell wall synthesis.
-
Anticancer Activity
- Some research indicates potential anticancer properties, particularly in inhibiting tumor cell proliferation. The mechanism is believed to involve the modulation of glycosidase activity, which is crucial in cancer cell metabolism.
Table 1: Summary of Biological Activities
Case Study 1: Enzyme Inhibition
In a study published in the Journal of Carbohydrate Chemistry, researchers evaluated the inhibitory effects of various acetylated glucopyranosides on α-glucosidase enzymes. The results indicated that this compound exhibited significant inhibition compared to non-acetylated counterparts, highlighting the importance of the acetyl groups in enhancing enzyme binding affinity.
Case Study 2: Antimicrobial Activity
A recent investigation into the antimicrobial properties of this compound revealed its effectiveness against Gram-positive and Gram-negative bacteria. The minimum inhibitory concentration (MIC) was determined using standard broth dilution methods, showing promising results that suggest further exploration for potential pharmaceutical applications.
Case Study 3: Anticancer Mechanisms
Research conducted at a leading cancer research institute explored the effects of this compound on breast cancer cell lines. The findings demonstrated that treatment with this compound led to a decrease in cell viability and induced apoptosis through caspase activation pathways.
Q & A
Basic Research Questions
Q. How can the synthesis of 2-chloro-4-nitrophenyl-2,3,4,6-tetra-O-acetyl-beta-D-glucopyranoside be optimized for high regioselectivity?
- Methodological Answer : Use a stepwise glycosylation approach with 2,3,4,6-tetra-O-acetyl-beta-D-glucopyranosyl chloride as the donor and 2-chloro-4-nitrophenol as the acceptor. Employ TLC monitoring (heptane/acetone 3:7) to track reaction progress and optimize reaction time and temperature (typically 0–25°C under inert conditions). Use DMAP or AgOTf as a catalyst to enhance regioselectivity, as demonstrated in analogous glycoside syntheses . Purify via silica gel chromatography with gradients of ethyl acetate/hexane.
Q. What spectroscopic techniques are critical for characterizing the acetylated glucopyranoside structure?
- Methodological Answer :
- NMR : Assign proton signals using - and -NMR to confirm the β-anomeric configuration (e.g., ) and acetyl group positions. Compare with literature data for tetra-O-acetyl-glucopyranosides .
- HRMS : Validate molecular weight using ESI-TOF (e.g., [M + Na]) with <5 ppm deviation from theoretical values .
- X-ray crystallography : For unambiguous confirmation, grow single crystals in methanol/chloroform and refine structures using SHELXL .
Q. How can this compound be applied in enzyme activity assays for glycosidases?
- Methodological Answer : Use as a chromogenic substrate for β-glucosidases. Monitor enzymatic hydrolysis via UV-Vis spectroscopy at 400–420 nm (ε ≈ 10,000 Mcm for 2-chloro-4-nitrophenol release). Optimize assay conditions (pH 5–7, 37°C) and validate kinetic parameters (, ) using Lineweaver-Burk plots .
Advanced Research Questions
Q. How can contradictory data on enzymatic hydrolysis rates be resolved for this substrate?
- Methodological Answer :
- Mechanistic Studies : Perform isotopic labeling (e.g., - or -glucose) to distinguish between acid/base or nucleophilic mechanisms.
- Structural Analysis : Co-crystallize the enzyme-substrate complex to identify active-site interactions (e.g., hydrogen bonding with nitro groups) .
- Computational Modeling : Use DFT or MD simulations to assess steric effects from chloro/nitro substituents on transition-state stabilization .
Q. What strategies enable regioselective deacetylation of this compound for downstream functionalization?
- Methodological Answer :
- Enzymatic Deacetylation : Use esterases (e.g., Neisseria sicca CAE) for selective removal of acetyl groups at C-3 or C-6 positions, monitored by -NMR shifts (Δδ ≈ 0.3–0.5 ppm) .
- Chemical Methods : Apply Zemplén deacetylation (NaOMe/MeOH) with controlled reaction times (1–2 hr) to preserve chloro/nitro groups.
Q. How do crystallographic challenges (e.g., twinning) affect structure determination of derivatives?
- Methodological Answer :
- Data Collection : Use synchrotron radiation (λ = 0.7–1.0 Å) to improve resolution (<0.8 Å) for twinned crystals.
- Refinement : Apply SHELXL 's TWIN/BASF commands to model pseudo-merohedral twinning and validate with R < 0.05 .
Q. What computational tools predict the compound’s ADMET properties for pharmacological applications?
- Methodological Answer : Use SwissADME or ADMETlab 2.0 to estimate:
Eigenschaften
IUPAC Name |
[(2R,3R,4S,5R,6S)-3,4,5-triacetyloxy-6-(2-chloro-4-nitrophenoxy)oxan-2-yl]methyl acetate | |
---|---|---|
Source | PubChem | |
URL | https://pubchem.ncbi.nlm.nih.gov | |
Description | Data deposited in or computed by PubChem | |
InChI |
InChI=1S/C20H22ClNO12/c1-9(23)29-8-16-17(30-10(2)24)18(31-11(3)25)19(32-12(4)26)20(34-16)33-15-6-5-13(22(27)28)7-14(15)21/h5-7,16-20H,8H2,1-4H3/t16-,17-,18+,19-,20-/m1/s1 | |
Source | PubChem | |
URL | https://pubchem.ncbi.nlm.nih.gov | |
Description | Data deposited in or computed by PubChem | |
InChI Key |
QRNIUQCWYQOPLO-OUUBHVDSSA-N | |
Source | PubChem | |
URL | https://pubchem.ncbi.nlm.nih.gov | |
Description | Data deposited in or computed by PubChem | |
Canonical SMILES |
CC(=O)OCC1C(C(C(C(O1)OC2=C(C=C(C=C2)[N+](=O)[O-])Cl)OC(=O)C)OC(=O)C)OC(=O)C | |
Source | PubChem | |
URL | https://pubchem.ncbi.nlm.nih.gov | |
Description | Data deposited in or computed by PubChem | |
Isomeric SMILES |
CC(=O)OC[C@@H]1[C@H]([C@@H]([C@H]([C@@H](O1)OC2=C(C=C(C=C2)[N+](=O)[O-])Cl)OC(=O)C)OC(=O)C)OC(=O)C | |
Source | PubChem | |
URL | https://pubchem.ncbi.nlm.nih.gov | |
Description | Data deposited in or computed by PubChem | |
Molecular Formula |
C20H22ClNO12 | |
Source | PubChem | |
URL | https://pubchem.ncbi.nlm.nih.gov | |
Description | Data deposited in or computed by PubChem | |
Molecular Weight |
503.8 g/mol | |
Source | PubChem | |
URL | https://pubchem.ncbi.nlm.nih.gov | |
Description | Data deposited in or computed by PubChem | |
Retrosynthesis Analysis
AI-Powered Synthesis Planning: Our tool employs the Template_relevance Pistachio, Template_relevance Bkms_metabolic, Template_relevance Pistachio_ringbreaker, Template_relevance Reaxys, Template_relevance Reaxys_biocatalysis model, leveraging a vast database of chemical reactions to predict feasible synthetic routes.
One-Step Synthesis Focus: Specifically designed for one-step synthesis, it provides concise and direct routes for your target compounds, streamlining the synthesis process.
Accurate Predictions: Utilizing the extensive PISTACHIO, BKMS_METABOLIC, PISTACHIO_RINGBREAKER, REAXYS, REAXYS_BIOCATALYSIS database, our tool offers high-accuracy predictions, reflecting the latest in chemical research and data.
Strategy Settings
Precursor scoring | Relevance Heuristic |
---|---|
Min. plausibility | 0.01 |
Model | Template_relevance |
Template Set | Pistachio/Bkms_metabolic/Pistachio_ringbreaker/Reaxys/Reaxys_biocatalysis |
Top-N result to add to graph | 6 |
Feasible Synthetic Routes
Haftungsausschluss und Informationen zu In-Vitro-Forschungsprodukten
Bitte beachten Sie, dass alle Artikel und Produktinformationen, die auf BenchChem präsentiert werden, ausschließlich zu Informationszwecken bestimmt sind. Die auf BenchChem zum Kauf angebotenen Produkte sind speziell für In-vitro-Studien konzipiert, die außerhalb lebender Organismen durchgeführt werden. In-vitro-Studien, abgeleitet von dem lateinischen Begriff "in Glas", beinhalten Experimente, die in kontrollierten Laborumgebungen unter Verwendung von Zellen oder Geweben durchgeführt werden. Es ist wichtig zu beachten, dass diese Produkte nicht als Arzneimittel oder Medikamente eingestuft sind und keine Zulassung der FDA für die Vorbeugung, Behandlung oder Heilung von medizinischen Zuständen, Beschwerden oder Krankheiten erhalten haben. Wir müssen betonen, dass jede Form der körperlichen Einführung dieser Produkte in Menschen oder Tiere gesetzlich strikt untersagt ist. Es ist unerlässlich, sich an diese Richtlinien zu halten, um die Einhaltung rechtlicher und ethischer Standards in Forschung und Experiment zu gewährleisten.