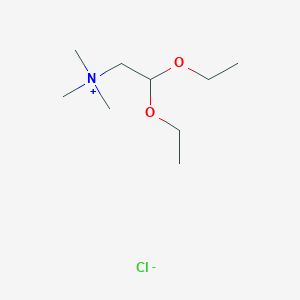
Betainaldehyd-Diethylacetal-Chlorid
Übersicht
Beschreibung
CCI-779, also known as temsirolimus, is an ester of rapamycin and an inhibitor of the mammalian target of rapamycin (mTOR). It is an anticancer drug approved by the Food and Drug Administration for the treatment of advanced renal cell carcinoma. CCI-779 has shown promising antitumor activity in various tumor types, including breast cancer, glioma, endometrial cancer, and mantle cell lymphoma .
Wissenschaftliche Forschungsanwendungen
CCI-779 hat eine breite Palette von Anwendungen in der wissenschaftlichen Forschung, insbesondere in den Bereichen Chemie, Biologie, Medizin und Industrie. In der Krebsforschung hat CCI-779 in präklinischen Modellen und klinischen Studien eine signifikante Antitumoraktivität gezeigt. Es wird verwendet, um die mTOR-Kinaseaktivität zu hemmen, was zur Hemmung der Zellproliferation und Proteinsynthese führt .
Wirkmechanismus
Der Wirkmechanismus von CCI-779 beinhaltet die Hemmung der mTOR-Kinasefunktion. mTOR ist eine Serin/Threonin-Kinase, die die Proteinsynthese, das Zellwachstum und die Proliferation reguliert. CCI-779 interagiert mit mTOR und hemmt seine Kinaseaktivität, was zur Unterdrückung von mTOR-Signalwegen führt . Diese Hemmung führt zu einer verringerten Translation von mRNAs, die an der Zellzykluskontrolle beteiligt sind, was letztendlich zu einem Zellzyklusarrest und einem verringerten Tumorwachstum führt .
Wirkmechanismus
Target of Action
Betainealdehyde Diethylacetal Chloride is a derivative of betaine . Betaine, also referred to as trimethyglycine, oxyneurine, and glycine-betaine, is a naturally occurring human nutrient . Its primary targets are the enzymes involved in the methionine cycle . Specifically, it interacts with the enzyme betaine-homocysteine methyltransferase (BHMT), a zinc thiol-enzyme expressed mainly in the liver and kidneys .
Mode of Action
Betainealdehyde Diethylacetal Chloride, like betaine, donates a methyl group to homocysteine to form N,N-dimethylglycine and L-methionine via the enzyme BHMT .
Biochemical Pathways
Betainealdehyde Diethylacetal Chloride affects the methionine cycle . In this cycle, betaine donates a methyl group to homocysteine to form N,N-dimethylglycine and L-methionine . This increases S-adenosylmethionine (SAM) levels, which serves as a methyl donor in DNA methylation and many anabolic pathways of phospholipids, hormones, and proteins .
Pharmacokinetics
Betaine, from which it is derived, is absorbed from the duodenal enterocytes into circulation and maintained between 20 and 70 µmol l −1 with a median plasma concentration of 278 µmol L −1 . It is carried to the liver and kidneys where it is catabolized by a series of enzyme-catalyzed reactions, predominantly in the mitochondria . Removal of betaine from the body is primarily by metabolism with minimal urinary excretion, even after high amounts of betaine consumption .
Result of Action
The molecular and cellular effects of Betainealdehyde Diethylacetal Chloride’s action are likely similar to those of betaine. Betaine’s ability to donate a methyl group plays a pivotal role in numerous pathways, including the methionine cycle . A betaine-deficient diet can disturb several cellular processes . Therefore, betaine supplementation to ameliorate certain pathological conditions has been envisaged .
Action Environment
Environmental factors can influence the action, efficacy, and stability of Betainealdehyde Diethylacetal Chloride. As with betaine, it is likely to act as a stress protectant against drought, osmotic, and temperature stress . Uncatabolized betaine acts as an osmolyte and confers protection to the cells against environmental stresses like osmotic irregularity, adverse temperatures, and dehydration . By regulating the surface tension of water, it aids in water retention and thereby maintains cellular volume .
Biochemische Analyse
Biochemical Properties
Betainealdehyde Diethylacetal Chloride is a derivative of betaine , a compound that plays a crucial role in various biochemical reactions. Betaine is known to interact with a variety of enzymes, proteins, and other biomolecules. For instance, it is involved in the metabolism of methyl groups in a variety of microorganisms . Betaine aldehyde dehydrogenase (BADH) is one such enzyme that interacts with betaine, catalyzing the oxidation of betaine aldehyde to betaine .
Cellular Effects
Betaine, from which Betainealdehyde Diethylacetal Chloride is derived, has significant effects on various types of cells and cellular processes. It is known to protect microbial cells against drought, osmotic stress, and temperature stress . Furthermore, betaine supplementation can improve the performance of microbial strains used for the fermentation of lactate, ethanol, lysine, pyruvate, and vitamin B12 .
Molecular Mechanism
The molecular mechanism of Betainealdehyde Diethylacetal Chloride is closely related to that of betaine. Betaine donates a methyl group to homocysteine to form methionine via the enzyme betaine-homocysteine methyltransferase (BHMT), resulting in the formation of dimethylglycine .
Dosage Effects in Animal Models
While there is limited information on the dosage effects of Betainealdehyde Diethylacetal Chloride in animal models, studies on betaine, its derivative, have shown that it improves growth performance in production animals, particularly under increased environmental temperatures .
Metabolic Pathways
Betainealdehyde Diethylacetal Chloride is involved in the metabolic pathways of betaine. Betaine is synthesized through a two-step oxidation of choline via the intermediate betaine aldehyde .
Transport and Distribution
Dietary betaine, from which it is derived, is absorbed from the duodenal enterocytes into circulation and is carried to the liver and kidneys where it is catabolized by a series of enzyme-catalyzed reactions .
Subcellular Localization
Studies on betaine aldehyde dehydrogenase (BADH), an enzyme that interacts with betaine, have shown that BADH proteins are localized to the cytosol and dot-shaped organelles in the mesophyll and bundle sheath cells of plant leaves .
Vorbereitungsmethoden
Die Herstellung von CCI-779 umfasst mehrere Syntheserouten und Reaktionsbedingungen. Ein Verfahren beinhaltet die Herstellung des Dioxans von 5 Carboxyl von 2,2,5 Trimethyl 1,3 . Die industriellen Produktionsverfahren für CCI-779 sind in der verfügbaren Literatur nicht umfassend beschrieben, beinhalten aber im Allgemeinen die Veresterung von Rapamycin mit spezifischen Reagenzien unter kontrollierten Bedingungen.
Analyse Chemischer Reaktionen
CCI-779 unterliegt verschiedenen chemischen Reaktionen, darunter Oxidation, Reduktion und Substitution. Häufige Reagenzien und Bedingungen, die in diesen Reaktionen verwendet werden, umfassen spezifische Antikörper und Inhibitoren, die mit mTOR und seinen zugehörigen Signalwegen interagieren . Die Hauptprodukte, die aus diesen Reaktionen gebildet werden, stehen typischerweise in Zusammenhang mit der Hemmung von mTOR-regulierten Translationskontrollern wie p70 s6 Kinase und 4E-BP1 .
Vergleich Mit ähnlichen Verbindungen
CCI-779 ähnelt anderen mTOR-Inhibitoren wie Rapamycin und Everolimus. CCI-779 hat einzigartige Eigenschaften, die es von diesen Verbindungen unterscheiden. Beispielsweise hat CCI-779 in klinisch relevanten Konzentrationen eine stärkere Hemmung der Proteinsynthese und Zellproliferation gezeigt . Andere ähnliche Verbindungen umfassen Sirolimus und Zotarolimus, die ebenfalls auf die mTOR-Signalübertragung abzielen, aber unterschiedliche pharmakokinetische und pharmakodynamische Profile aufweisen können .
Referenzen
Biologische Aktivität
Betainealdehyde diethylacetal chloride is a compound of interest due to its potential biological activities and applications in various fields, including agriculture and medicine. This article explores the biological activity of this compound, focusing on its enzymatic interactions, metabolic pathways, and potential therapeutic benefits.
Overview of Betainealdehyde Diethylacetal Chloride
Betainealdehyde diethylacetal chloride is derived from betaine aldehyde, which plays a significant role in various biological processes. It is important to understand how this compound interacts with biological systems and its implications for health and disease.
Enzymatic Activity
Betaine Aldehyde Dehydrogenase (BADH)
One of the key enzymes associated with betainealdehyde diethylacetal chloride is betaine aldehyde dehydrogenase (BADH). BADH catalyzes the oxidation of betaine aldehyde to glycine betaine, a process crucial for osmotic regulation in plants and microorganisms.
- Kinetics : Studies have shown that BADH exhibits a high affinity for betaine aldehyde, with an apparent value of approximately molar, indicating its specificity for this substrate . The enzyme's activity is influenced by various factors, including the presence of coenzymes such as NAD+.
- Physiological Role : BADH plays a vital role in helping organisms cope with osmotic stress. For example, plants expressing BADH have demonstrated increased tolerance to salinity and drought conditions .
Metabolic Pathways
Betainealdehyde diethylacetal chloride is involved in several metabolic pathways that are essential for maintaining cellular homeostasis.
- Transmethylation Pathway : This pathway links betaine to the metabolism of homocysteine and methionine. The conversion of homocysteine to methionine via methylation is crucial for DNA methylation processes, impacting gene expression and cellular function .
- Fatty Acid Metabolism : Research indicates that compounds related to betaine can influence lipid metabolism by reducing fat accumulation in the liver. This effect is mediated through enhanced fatty acid oxidation and reduced lipogenesis .
Biological Effects
Health Benefits
The biological activity of betainealdehyde diethylacetal chloride extends to several health-promoting effects:
- Liver Health : Studies have shown that betaine supplementation can significantly lower hepatic transaminases and improve liver function markers in patients with chronic liver conditions .
- Antioxidant Properties : Betaine has been associated with increased levels of antioxidant enzymes such as glutathione peroxidase and superoxide dismutase (SOD), which help mitigate oxidative stress .
Case Studies
- Chronic Hepatitis B Treatment : In a clinical study involving children with chronic hepatitis B, treatment with a betaine-arginine complex resulted in notable improvements in liver function and reduction in pain syndromes .
- Osmotic Stress in Plants : Transgenic plants expressing BADH showed enhanced growth under osmotic stress conditions, demonstrating the enzyme's role in stress tolerance mechanisms .
Data Summary
Parameter | Value/Observation |
---|---|
BADH for betaine aldehyde | molar |
Effect on hepatic transaminases | Significant reduction observed |
Antioxidant enzyme activity | Increased glutathione peroxidase and SOD levels |
Impact on plant stress tolerance | Enhanced growth under osmotic stress conditions |
Eigenschaften
IUPAC Name |
2,2-diethoxyethyl(trimethyl)azanium;chloride | |
---|---|---|
Source | PubChem | |
URL | https://pubchem.ncbi.nlm.nih.gov | |
Description | Data deposited in or computed by PubChem | |
InChI |
InChI=1S/C9H22NO2.ClH/c1-6-11-9(12-7-2)8-10(3,4)5;/h9H,6-8H2,1-5H3;1H/q+1;/p-1 | |
Source | PubChem | |
URL | https://pubchem.ncbi.nlm.nih.gov | |
Description | Data deposited in or computed by PubChem | |
InChI Key |
PJKADRIYSJAEIV-UHFFFAOYSA-M | |
Source | PubChem | |
URL | https://pubchem.ncbi.nlm.nih.gov | |
Description | Data deposited in or computed by PubChem | |
Canonical SMILES |
CCOC(C[N+](C)(C)C)OCC.[Cl-] | |
Source | PubChem | |
URL | https://pubchem.ncbi.nlm.nih.gov | |
Description | Data deposited in or computed by PubChem | |
Molecular Formula |
C9H22ClNO2 | |
Source | PubChem | |
URL | https://pubchem.ncbi.nlm.nih.gov | |
Description | Data deposited in or computed by PubChem | |
DSSTOX Substance ID |
DTXSID10616954 | |
Record name | 2,2-Diethoxy-N,N,N-trimethylethan-1-aminium chloride | |
Source | EPA DSSTox | |
URL | https://comptox.epa.gov/dashboard/DTXSID10616954 | |
Description | DSSTox provides a high quality public chemistry resource for supporting improved predictive toxicology. | |
Molecular Weight |
211.73 g/mol | |
Source | PubChem | |
URL | https://pubchem.ncbi.nlm.nih.gov | |
Description | Data deposited in or computed by PubChem | |
CAS No. |
110675-66-6 | |
Record name | 2,2-Diethoxy-N,N,N-trimethylethan-1-aminium chloride | |
Source | EPA DSSTox | |
URL | https://comptox.epa.gov/dashboard/DTXSID10616954 | |
Description | DSSTox provides a high quality public chemistry resource for supporting improved predictive toxicology. | |
Retrosynthesis Analysis
AI-Powered Synthesis Planning: Our tool employs the Template_relevance Pistachio, Template_relevance Bkms_metabolic, Template_relevance Pistachio_ringbreaker, Template_relevance Reaxys, Template_relevance Reaxys_biocatalysis model, leveraging a vast database of chemical reactions to predict feasible synthetic routes.
One-Step Synthesis Focus: Specifically designed for one-step synthesis, it provides concise and direct routes for your target compounds, streamlining the synthesis process.
Accurate Predictions: Utilizing the extensive PISTACHIO, BKMS_METABOLIC, PISTACHIO_RINGBREAKER, REAXYS, REAXYS_BIOCATALYSIS database, our tool offers high-accuracy predictions, reflecting the latest in chemical research and data.
Strategy Settings
Precursor scoring | Relevance Heuristic |
---|---|
Min. plausibility | 0.01 |
Model | Template_relevance |
Template Set | Pistachio/Bkms_metabolic/Pistachio_ringbreaker/Reaxys/Reaxys_biocatalysis |
Top-N result to add to graph | 6 |
Feasible Synthetic Routes
Haftungsausschluss und Informationen zu In-Vitro-Forschungsprodukten
Bitte beachten Sie, dass alle Artikel und Produktinformationen, die auf BenchChem präsentiert werden, ausschließlich zu Informationszwecken bestimmt sind. Die auf BenchChem zum Kauf angebotenen Produkte sind speziell für In-vitro-Studien konzipiert, die außerhalb lebender Organismen durchgeführt werden. In-vitro-Studien, abgeleitet von dem lateinischen Begriff "in Glas", beinhalten Experimente, die in kontrollierten Laborumgebungen unter Verwendung von Zellen oder Geweben durchgeführt werden. Es ist wichtig zu beachten, dass diese Produkte nicht als Arzneimittel oder Medikamente eingestuft sind und keine Zulassung der FDA für die Vorbeugung, Behandlung oder Heilung von medizinischen Zuständen, Beschwerden oder Krankheiten erhalten haben. Wir müssen betonen, dass jede Form der körperlichen Einführung dieser Produkte in Menschen oder Tiere gesetzlich strikt untersagt ist. Es ist unerlässlich, sich an diese Richtlinien zu halten, um die Einhaltung rechtlicher und ethischer Standards in Forschung und Experiment zu gewährleisten.