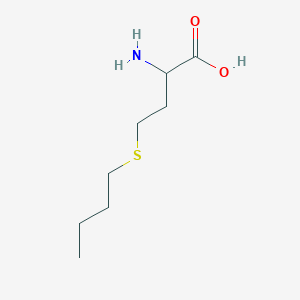
D,L-Buthionine
Übersicht
Beschreibung
D,L-Buthionine (CAS 3343-64-4) is a synthetic sulfur-containing amino acid derivative with the molecular formula C₈H₁₇NO₂S and a molecular weight of 191.29 g/mol. Structurally, it is the racemic mixture of the D- and L-isomers of S-butyl-homocysteine. This compound is primarily recognized for its role in modulating glutathione (GSH) synthesis by inhibiting γ-glutamylcysteine ligase (γ-GCL), the rate-limiting enzyme in GSH biosynthesis . Its ability to deplete intracellular glutathione has made it a tool for studying oxidative stress and apoptosis in cellular models .
Vorbereitungsmethoden
Synthetic Routes and Reaction Conditions: D,L-Buthionine can be synthesized through various methods. One common approach involves the reaction of butylamine with a suitable sulfoximine precursor under controlled conditions. The reaction typically requires a catalyst and specific temperature and pressure settings to ensure optimal yield and purity.
Industrial Production Methods: In industrial settings, the production of this compound involves large-scale chemical synthesis using automated reactors. The process is carefully monitored to maintain consistent quality and to minimize impurities. The final product is then purified through crystallization or chromatography techniques.
Analyse Chemischer Reaktionen
Types of Reactions: D,L-Buthionine undergoes several types of chemical reactions, including:
Oxidation: This reaction involves the addition of oxygen or the removal of hydrogen, often resulting in the formation of sulfoxides or sulfones.
Reduction: This reaction involves the addition of hydrogen or the removal of oxygen, leading to the formation of sulfides.
Substitution: This reaction involves the replacement of one functional group with another, such as the substitution of a hydrogen atom with a halogen.
Common Reagents and Conditions:
Oxidation: Common reagents include hydrogen peroxide and potassium permanganate, typically under acidic or basic conditions.
Reduction: Common reagents include lithium aluminum hydride and sodium borohydride, usually under anhydrous conditions.
Substitution: Common reagents include halogens and alkylating agents, often under neutral or slightly basic conditions.
Major Products: The major products formed from these reactions include sulfoxides, sulfones, and various substituted derivatives, depending on the specific reaction conditions and reagents used.
Wissenschaftliche Forschungsanwendungen
Cancer Research
Mechanism of Action
BSO inhibits the synthesis of GSH, leading to decreased intracellular GSH levels. This depletion can sensitize cancer cells to chemotherapeutic agents, particularly those that rely on GSH for detoxification.
Case Study: Reversal of Drug Resistance
A study demonstrated that BSO could reverse multidrug resistance in tumor models. In athymic nude mice with human fibrosarcoma xenografts, BSO treatment significantly reduced GSH levels in both sensitive and resistant tumors. When combined with doxorubicin, BSO restored the drug's efficacy against MRP-expressing tumors, achieving a 63% overall response rate, compared to no significant activity when doxorubicin was used alone .
Tumor Model | Response Rate with Doxorubicin Alone | Response Rate with Doxorubicin + BSO |
---|---|---|
HT1080 (sensitive) | 50% | 63% |
HT1080/DR4 (resistant) | No significant activity | 63% |
Plant Biology
Enhancement of Somatic Embryo Development
BSO has been utilized in plant tissue culture to improve somatic embryo development. In a study involving white spruce (Picea glauca), BSO treatment resulted in a tripling of embryogenic output by increasing the number of morphologically normal embryos produced . The use of BSO shifted the total glutathione pool towards its oxidized form (GSSG), promoting better embryo quality and conversion rates.
Cell Line | Embryos Produced per 100 mg Tissue | Conversion Frequency Improvement |
---|---|---|
WS1 | 65 to 154 | Increased |
WS2 | 59 to 130 | Enhanced root emergence |
Oxidative Stress Studies
Role in Hypertension Research
BSO has been studied for its effects on oxidative stress related to hypertension. In experiments with Wistar albino rats, BSO administration demonstrated significant changes in blood pressure and renal function when combined with nitric oxide synthase inhibitors. The study highlighted the role of oxidative stress in hypertension development and the potential for BSO to modulate these effects .
Treatment Group | Blood Pressure Change | Sodium Reabsorption (%) |
---|---|---|
Control | Baseline | 100% |
L-NNA | Increased | Reduced |
BSO | Moderate Increase | Enhanced |
L-NNA + BSO | Significant Increase | Improved |
Toxicology and Radiobiology
Potentiation of Radiation Effects
Research has shown that BSO can enhance the effects of radiation therapy by depleting GSH levels in cells. This depletion increases susceptibility to radiation-induced damage, making it a potential adjuvant in radiotherapy protocols .
Wirkmechanismus
D,L-Buthionine exerts its effects primarily by inhibiting the enzyme gamma-glutamylcysteine synthetase, which is essential for the synthesis of glutathione. By reducing glutathione levels, this compound increases cellular oxidative stress, leading to apoptosis in cancer cells. This mechanism makes it a valuable adjunct in cancer treatment, particularly in combination with other chemotherapeutic agents.
Vergleich Mit ähnlichen Verbindungen
L-Buthionine Sulfoximine (BSO)
- Structure and Function : BSO (NSC 326231) is a sulfoximine derivative of L-buthionine and a well-characterized irreversible inhibitor of γ-GCL. Unlike D,L-Buthionine, BSO directly binds to the enzyme’s active site, leading to prolonged glutathione depletion .
- Efficacy : Studies show that BSO reduces intracellular GSH to undetectable levels within 12–24 hours at concentrations of 5–200 mM . In contrast, this compound achieves 50% inhibition of γ-GCL activity at lower concentrations, suggesting higher potency in enzyme modulation .
- Applications : BSO is widely used in cancer research to sensitize tumors to chemotherapy and radiation by exacerbating oxidative stress . This compound, however, is less studied in therapeutic contexts but shows promise in mechanistic studies of redox biology .
L-Methionine and Derivatives
- Structural Similarities: L-Methionine (C₅H₁₁NO₂S) shares a sulfur-containing backbone with this compound but lacks the extended alkyl chain (butyl group). This structural difference impacts their biological interactions.
- Oxidative Reactivity : Oxidation studies using [FeIII-Salen]Cl complexes reveal that L-Buthionine reacts more slowly than L-Methionine due to steric hindrance from its longer side chain, highlighting differences in redox behavior .
Zinc L-Carnosine
- Antioxidant Mechanisms: Zinc L-Carnosine protects cells from BSO-induced oxidative stress by upregulating metallothionein and superoxide dismutase (SOD1), whereas this compound exacerbates oxidative damage by depleting GSH . This contrast underscores the dual roles of sulfur-containing compounds as either pro-oxidants or antioxidants depending on their mechanisms.
Other NSA Derivatives
- Inhibitory Specificity : Among straight-chain, sulfur-containing NSA derivatives, this compound stands out for its low dissociation constant (kD) and high γ-GCL inhibition efficiency compared to shorter-chain analogs like L-Ethionine .
Data Table: Key Comparative Metrics
Key Research Findings
Biologische Aktivität
D,L-Buthionine-(S,R)-sulfoximine (BSO) is a potent inhibitor of glutathione (GSH) biosynthesis, primarily affecting the enzyme γ-glutamylcysteine synthetase. This compound has garnered attention for its role in cancer research, particularly in overcoming drug resistance in tumor cells. The biological activity of BSO is primarily characterized by its ability to modulate intracellular GSH levels, which has significant implications for therapeutic strategies against various cancers.
BSO functions by irreversibly inhibiting the synthesis of GSH, a critical antioxidant that protects cells from oxidative stress and is involved in detoxifying chemotherapeutic agents. By depleting GSH levels, BSO enhances the sensitivity of cancer cells to certain chemotherapeutic drugs. The following mechanisms have been identified:
- Inhibition of Drug Resistance : BSO has been shown to reverse multidrug resistance (MDR) in various cancer cell lines by reducing GSH levels, which are necessary for the function of multidrug resistance proteins (MRPs) that extrude drugs from cells .
- Increased Oxidative Stress : The depletion of GSH leads to increased oxidative stress within cancer cells, rendering them more susceptible to cytotoxic agents .
- Enhanced DNA Cross-linking : BSO treatment has been associated with increased DNA cross-linking induced by bifunctional alkylating agents, thereby enhancing their cytotoxic effects .
In Vivo Studies
- Tumor Models : In a study involving athymic nude mice with human fibrosarcoma xenografts, BSO administration led to a significant reduction in GSH levels in both plasma and tumors. When combined with doxorubicin, BSO restored the drug's efficacy against MRP-expressing tumors, achieving an overall response rate of 63% .
- Breast Cancer Cells : Another study demonstrated that inhibiting glutamate cysteine ligase (GCL) activity with BSO during exposure to 2-deoxy-D-glucose sensitized human breast cancer cells to cytotoxicity. This was accompanied by increased markers of oxidative stress, indicating that BSO can enhance the effects of metabolic inhibitors in cancer therapy .
In Vitro Studies
- Melanoma Cell Line : Research on RPMI 8322 human melanoma cells showed that pretreatment with BSO significantly enhanced the cytotoxicity of melphalan and nitrogen mustard, correlating with increased DNA cross-linking without affecting cell proliferation rates . This suggests that BSO can be an effective adjunct in chemotherapy regimens targeting resistant melanoma.
Summary of Findings
Toxicological Considerations
While BSO is a valuable tool for sensitizing cancer cells to chemotherapy, its use must be carefully considered due to potential toxicity. Studies have indicated that high doses can lead to adverse effects, including kidney toxicity and immunotoxicity . Therefore, dosing strategies should aim to maximize therapeutic benefit while minimizing risks.
Q & A
Basic Research Questions
Q. What are the standard methodologies for synthesizing and characterizing D,L-Buthionine in experimental settings?
- Methodological Answer : Synthesis typically involves chemical condensation of cysteine derivatives with alkyl halides. Characterization requires nuclear magnetic resonance (NMR) for structural confirmation and high-performance liquid chromatography (HPLC) for purity analysis. For reproducibility, experimental protocols must detail reaction conditions (temperature, solvent, stoichiometry) and validation against reference spectra .
Q. How can researchers validate the efficacy of this compound in glutathione depletion studies?
- Methodological Answer : Use cell-based assays (e.g., HepG2 or primary hepatocytes) to measure intracellular glutathione levels via Ellman’s assay or LC-MS. Include positive controls (e.g., BSO, a known glutathione inhibitor) and account for cell viability using MTT assays. Dose-response curves and time-course studies are critical to establish dynamic range and temporal effects .
Q. What literature sources are essential for contextualizing this compound’s role in oxidative stress research?
- Methodological Answer : Prioritize primary literature from journals like Free Radical Biology and Medicine or Redox Biology. Use specialized databases (e.g., SciFinder, PubMed) with keywords like “glutathione synthesis inhibition” or “ROS modulation.” Cross-reference citations in review articles to identify foundational studies and recent advances .
Advanced Research Questions
Q. How should experimental designs address conflicting data on this compound’s off-target effects in mammalian systems?
- Methodological Answer : Employ orthogonal validation techniques (e.g., CRISPR-based gene editing to knock out alternative glutathione pathways) and multi-omics approaches (transcriptomics/proteomics) to identify unintended targets. Use the FINER criteria to refine hypotheses: ensure feasibility by piloting small-scale screens and assess relevance through mechanistic studies .
Q. What strategies optimize this compound delivery in in vivo models while minimizing toxicity?
- Methodological Answer : Conduct pharmacokinetic studies to determine bioavailability and tissue distribution. Use nanoparticle encapsulation or prodrug formulations to enhance stability. Monitor toxicity via serum biomarkers (ALT/AST for liver damage) and histological analysis. Compare routes of administration (intraperitoneal vs. oral) for efficacy-toxicity trade-offs .
Q. How can researchers reconcile discrepancies in this compound’s potency across different cell lines?
- Methodological Answer : Standardize culture conditions (e.g., media composition, passage number) and quantify baseline glutathione levels in each cell line. Use RNA-seq to identify variability in γ-glutamylcysteine synthetase (GCL) expression, the enzyme targeted by this compound. Include isogenic cell lines to isolate genetic factors .
Q. Data Analysis and Reproducibility
Q. What statistical frameworks are appropriate for analyzing dose-dependent effects of this compound?
- Methodological Answer : Apply nonlinear regression models (e.g., sigmoidal dose-response curves) using tools like GraphPad Prism. Report IC₅₀ values with 95% confidence intervals and use ANOVA for multi-group comparisons. Validate assumptions of normality and homogeneity of variance with Shapiro-Wilk and Levene’s tests .
Q. How should researchers document experimental protocols to ensure reproducibility in this compound studies?
- Methodological Answer : Follow the Beilstein Journal of Organic Chemistry guidelines: provide step-by-step synthesis procedures, instrument calibration details, and raw data in supplementary materials. Use FAIR (Findable, Accessible, Interoperable, Reusable) principles for data deposition in repositories like Zenodo or Figshare .
Q. Ethical and Collaborative Considerations
Q. What ethical guidelines apply to studies using this compound in animal models?
- Methodological Answer : Adhere to ARRIVE 2.0 guidelines for preclinical studies: justify sample sizes, report exclusion criteria, and disclose humane endpoints. Obtain approval from institutional animal care committees (IACUC) and include statements on compliance in the manuscript’s ethics section .
Q. How can interdisciplinary teams streamline research on this compound’s therapeutic potential?
- Methodological Answer : Define roles using a Gantt chart to allocate tasks (e.g., chemists for synthesis, biologists for assays). Hold weekly data review meetings to align interpretations. Use collaborative platforms (e.g., LabArchives) for real-time data sharing and version control. Cite contributions transparently in authorship agreements .
Eigenschaften
IUPAC Name |
2-amino-4-butylsulfanylbutanoic acid | |
---|---|---|
Source | PubChem | |
URL | https://pubchem.ncbi.nlm.nih.gov | |
Description | Data deposited in or computed by PubChem | |
InChI |
InChI=1S/C8H17NO2S/c1-2-3-5-12-6-4-7(9)8(10)11/h7H,2-6,9H2,1H3,(H,10,11) | |
Source | PubChem | |
URL | https://pubchem.ncbi.nlm.nih.gov | |
Description | Data deposited in or computed by PubChem | |
InChI Key |
LAXXPOJCFVMVAX-UHFFFAOYSA-N | |
Source | PubChem | |
URL | https://pubchem.ncbi.nlm.nih.gov | |
Description | Data deposited in or computed by PubChem | |
Canonical SMILES |
CCCCSCCC(C(=O)O)N | |
Source | PubChem | |
URL | https://pubchem.ncbi.nlm.nih.gov | |
Description | Data deposited in or computed by PubChem | |
Molecular Formula |
C8H17NO2S | |
Source | PubChem | |
URL | https://pubchem.ncbi.nlm.nih.gov | |
Description | Data deposited in or computed by PubChem | |
DSSTOX Substance ID |
DTXSID60955062 | |
Record name | 2-Amino-4-(butylsulfanyl)butanoic acid | |
Source | EPA DSSTox | |
URL | https://comptox.epa.gov/dashboard/DTXSID60955062 | |
Description | DSSTox provides a high quality public chemistry resource for supporting improved predictive toxicology. | |
Molecular Weight |
191.29 g/mol | |
Source | PubChem | |
URL | https://pubchem.ncbi.nlm.nih.gov | |
Description | Data deposited in or computed by PubChem | |
CAS No. |
4378-14-7 | |
Record name | Buthionine | |
Source | ChemIDplus | |
URL | https://pubchem.ncbi.nlm.nih.gov/substance/?source=chemidplus&sourceid=0004378147 | |
Description | ChemIDplus is a free, web search system that provides access to the structure and nomenclature authority files used for the identification of chemical substances cited in National Library of Medicine (NLM) databases, including the TOXNET system. | |
Record name | 2-Amino-4-(butylsulfanyl)butanoic acid | |
Source | EPA DSSTox | |
URL | https://comptox.epa.gov/dashboard/DTXSID60955062 | |
Description | DSSTox provides a high quality public chemistry resource for supporting improved predictive toxicology. | |
Retrosynthesis Analysis
AI-Powered Synthesis Planning: Our tool employs the Template_relevance Pistachio, Template_relevance Bkms_metabolic, Template_relevance Pistachio_ringbreaker, Template_relevance Reaxys, Template_relevance Reaxys_biocatalysis model, leveraging a vast database of chemical reactions to predict feasible synthetic routes.
One-Step Synthesis Focus: Specifically designed for one-step synthesis, it provides concise and direct routes for your target compounds, streamlining the synthesis process.
Accurate Predictions: Utilizing the extensive PISTACHIO, BKMS_METABOLIC, PISTACHIO_RINGBREAKER, REAXYS, REAXYS_BIOCATALYSIS database, our tool offers high-accuracy predictions, reflecting the latest in chemical research and data.
Strategy Settings
Precursor scoring | Relevance Heuristic |
---|---|
Min. plausibility | 0.01 |
Model | Template_relevance |
Template Set | Pistachio/Bkms_metabolic/Pistachio_ringbreaker/Reaxys/Reaxys_biocatalysis |
Top-N result to add to graph | 6 |
Feasible Synthetic Routes
Haftungsausschluss und Informationen zu In-Vitro-Forschungsprodukten
Bitte beachten Sie, dass alle Artikel und Produktinformationen, die auf BenchChem präsentiert werden, ausschließlich zu Informationszwecken bestimmt sind. Die auf BenchChem zum Kauf angebotenen Produkte sind speziell für In-vitro-Studien konzipiert, die außerhalb lebender Organismen durchgeführt werden. In-vitro-Studien, abgeleitet von dem lateinischen Begriff "in Glas", beinhalten Experimente, die in kontrollierten Laborumgebungen unter Verwendung von Zellen oder Geweben durchgeführt werden. Es ist wichtig zu beachten, dass diese Produkte nicht als Arzneimittel oder Medikamente eingestuft sind und keine Zulassung der FDA für die Vorbeugung, Behandlung oder Heilung von medizinischen Zuständen, Beschwerden oder Krankheiten erhalten haben. Wir müssen betonen, dass jede Form der körperlichen Einführung dieser Produkte in Menschen oder Tiere gesetzlich strikt untersagt ist. Es ist unerlässlich, sich an diese Richtlinien zu halten, um die Einhaltung rechtlicher und ethischer Standards in Forschung und Experiment zu gewährleisten.