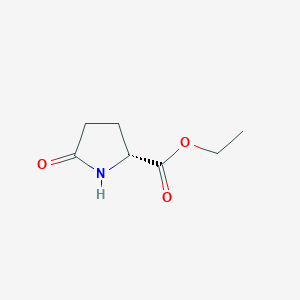
ethyl (2R)-5-oxopyrrolidine-2-carboxylate
Übersicht
Beschreibung
Ethyl (2R)-5-oxopyrrolidine-2-carboxylate is a chiral pyrrolidinone derivative with the molecular formula C₇H₁₁NO₃ (monoisotopic mass: 173.08 g/mol). It features a 5-membered lactam ring (pyrrolidinone) and an ethyl ester group at the C2 position with R-configuration . This compound is a key intermediate in organic synthesis, particularly in the preparation of peptidomimetics, alkaloids, and bioactive molecules. Its stereochemistry and functional group arrangement make it valuable for enantioselective reactions and drug discovery .
Wirkmechanismus
Target of Action
D-Pyroglutamic acid ethyl ester, also known as Ethyl ®-(-)-2-pyrrolidone-5-carboxylate, primarily targets the enzyme Pyroglutamyl-peptide hydrolase (EC 3.4.11.8) . This enzyme is responsible for removing the N-terminal pyroglutamyl residue from pyroglutamyl-containing peptides such as thyrotropin-releasing hormone (TRH), luteinizing hormone-releasing hormone (LH-RH), neurotensin, and bombesin .
Mode of Action
The compound interacts with its target enzyme by acting as a transition-state aldehyde inhibitor . This means it binds to the enzyme and inhibits its function, thereby preventing the removal of the N-terminal pyroglutamyl residue from the peptides .
Biochemical Pathways
The primary biochemical pathway affected by D-Pyroglutamic acid ethyl ester is the glutathione cycle . In this cycle, the compound is converted to glutamate by the action of 5-oxoprolinase . This process impacts the metabolism of glutathione, a crucial antioxidant in cells, and can have downstream effects on cellular redox balance and detoxification processes.
Pharmacokinetics
Given its molecular weight of 15717 , it is likely to be well-absorbed and distributed in the body. Its metabolism likely involves enzymatic processes, and it is expected to be excreted via the kidneys. These properties can impact the compound’s bioavailability, or the extent and rate at which it reaches its site of action.
Result of Action
The inhibition of Pyroglutamyl-peptide hydrolase by D-Pyroglutamic acid ethyl ester results in an accumulation of pyroglutamyl-containing peptides . This can have various molecular and cellular effects, depending on the specific peptides involved. For example, if the peptides are involved in hormone signaling, this could impact hormone levels and related physiological processes.
Biochemische Analyse
Biochemical Properties
D-Pyroglutamic acid ethyl ester is involved in various biochemical reactions. It is known to interact with several enzymes and proteins. For instance, it has been found to interact with pyroglutamyl-peptide hydrolase, an enzyme that removes the N-terminal pyroglutamyl residue from pyroglutamyl-containing peptides . The nature of these interactions is complex and involves various biochemical processes .
Cellular Effects
The effects of D-Pyroglutamic acid ethyl ester on cells and cellular processes are diverse. It influences cell function by impacting cell signaling pathways, gene expression, and cellular metabolism . For example, it has been found to exhibit antinociceptive activity, leading to an increase in the tail flick latency time in rats .
Molecular Mechanism
The molecular mechanism of action of D-Pyroglutamic acid ethyl ester involves its interactions at the molecular level. It exerts its effects through binding interactions with biomolecules, enzyme inhibition or activation, and changes in gene expression . For instance, it is known to interact with pyroglutamyl-peptide hydrolase, influencing its activity .
Temporal Effects in Laboratory Settings
In laboratory settings, the effects of D-Pyroglutamic acid ethyl ester change over time. It has been observed that the maximum value of the tail flick latency for this compound was manifested after 90 minutes of administration . This indicates the compound’s stability and its long-term effects on cellular function .
Dosage Effects in Animal Models
The effects of D-Pyroglutamic acid ethyl ester vary with different dosages in animal models. For instance, in a study where the compound was administered to rats in a dose of 20 mg/kg, it was found to exhibit antinociceptive activity . The specific threshold effects and any toxic or adverse effects at high doses are not mentioned in the available literature.
Metabolic Pathways
D-Pyroglutamic acid ethyl ester is involved in several metabolic pathways. It is derived from glutamine and is formed during the later stages of biosynthesis at the terminal phases of translation or as a post-translational event
Biologische Aktivität
Ethyl (2R)-5-oxopyrrolidine-2-carboxylate, also known as EOPC, is a synthetic compound that has garnered attention for its potential biological activities. This article explores its mechanisms of action, biological effects, and relevant research findings.
Chemical Structure and Properties
This compound is characterized by a pyrrolidine ring with a carboxylate group and an ethyl ester. Its molecular formula is , and it has a molecular weight of approximately 157.17 g/mol. The compound's structure allows it to participate in various chemical reactions, including oxidation and reduction, which can modify its biological activity.
Target Enzyme:
EOPC primarily targets the enzyme pyroglutamyl-peptide hydrolase (EC 3.4.11.8). This enzyme plays a crucial role in peptide metabolism by removing the N-terminal pyroglutamyl residue from peptides.
Mode of Action:
EOPC acts as a transition-state aldehyde inhibitor, which means it binds to the enzyme's active site, preventing substrate access and thereby inhibiting enzymatic activity. This inhibition leads to an accumulation of pyroglutamyl-containing peptides, which can affect various cellular processes and signaling pathways.
Antimicrobial Activity
Research indicates that compounds within the oxopyrrolidine class, including EOPC, exhibit antimicrobial properties against various bacterial strains. In one study, derivatives of oxopyrrolidine demonstrated significant efficacy against Gram-positive bacteria and drug-resistant fungi, suggesting potential applications in treating infections caused by resistant pathogens .
Antitumor Activity
EOPC has also been investigated for its antitumor properties. In vitro studies have shown that it can reduce the viability of cancer cell lines, including A549 human lung adenocarcinoma cells. The cytotoxic effects were notable when compared to standard chemotherapy agents like cisplatin . The structure-dependent nature of these compounds suggests that modifications to the core structure can enhance or diminish their anticancer efficacy.
Case Studies
-
Anticancer Efficacy:
A study involving various derivatives of EOPC assessed their ability to inhibit cell growth in A549 cells. Results indicated that some derivatives significantly reduced cell viability by up to 63% compared to untreated controls (p < 0.05). Notably, certain substitutions on the phenyl ring enhanced anticancer activity dramatically, indicating structure-activity relationships are crucial for efficacy . -
Enzyme Inhibition:
In biochemical assays, EOPC was shown to inhibit pyroglutamyl-peptide hydrolase effectively. This inhibition was quantified using kinetic assays that demonstrated a significant increase in substrate accumulation in the presence of EOPC, confirming its role as an enzyme inhibitor.
Comparative Analysis with Similar Compounds
Compound Name | Structure Characteristics | Biological Activity | Unique Features |
---|---|---|---|
This compound | Contains ethyl ester | Antimicrobial, Antitumor | Specific chiral configuration |
Ethyl (2S)-4,4-dimethyl-5-oxopyrrolidine-2-carboxylate | Dimethyl substitution | Antitumor | Enhanced binding affinity |
Methyl 5-oxopyrrolidine-2-carboxylate | Lacks ethyl group | Limited activity | Simpler structure |
This table illustrates how variations in chemical structure lead to different biological activities and potential applications in pharmacology.
Q & A
Basic Research Questions
Q. What synthetic methodologies are commonly employed for the preparation of ethyl (2R)-5-oxopyrrolidine-2-carboxylate, and how can stereochemical purity be ensured?
- Answer : this compound is typically synthesized via esterification of pyroglutamic acid derivatives or through diastereoselective cyclization. For example, Gorodnicheva et al. (2023) developed a diastereoselective neutralization reaction using diastereohomogeneous dimethyl (2R*,3R*)-3-aryl(pyridyl)glutamate hydrochlorides to yield methyl esters, which can be adapted for ethyl analogs by substituting methanol with ethanol . Stereochemical control is achieved via chiral auxiliaries or enantioselective catalysis, with X-ray crystallography (e.g., CCDC data in ) and chiral HPLC used to confirm configuration .
Q. What analytical techniques are critical for characterizing the purity and structure of this compound?
- Answer : Key techniques include:
- NMR Spectroscopy : H and C NMR to confirm ester groups (δ ~4.1–4.3 ppm for ethyl CH, δ ~170–175 ppm for carbonyl carbons).
- X-ray Crystallography : Resolves absolute configuration (e.g., C–C bond lengths of 1.52–1.54 Å in pyrrolidine rings, as shown in ) .
- Mass Spectrometry : ESI-MS for molecular ion validation (expected [M+H] at m/z 172.1).
- Elemental Analysis : Confirms C, H, N composition (e.g., CHNO requires C 52.16%, H 6.43%, N 8.69%) .
Q. How can researchers optimize the purification of this compound to minimize racemization?
- Answer : Low-temperature recrystallization (e.g., using ethyl acetate/hexane at –20°C) or flash chromatography with chiral stationary phases (e.g., amylose-based columns) preserves enantiomeric excess. Avoid prolonged heating during solvent removal to prevent ester hydrolysis or racemization .
Advanced Research Questions
Q. What strategies enhance diastereoselectivity in the synthesis of substituted 5-oxopyrrolidine-2-carboxylates, and how do reaction conditions influence outcomes?
- Answer : Diastereoselectivity is improved using:
- Chiral Solvents or Additives : e.g., (+)- or (–)-menthol derivatives (up to 85% de) .
- Temperature Control : Reactions at –40°C reduce kinetic competition, favoring thermodynamically stable diastereomers (e.g., 3-aryl derivatives in achieved 7:1 dr) .
- Catalytic Asymmetric Methods : Organocatalysts like proline derivatives or metal-ligand complexes (e.g., Cu(I)/BOX systems) .
Q. How does computational modeling aid in predicting the reactivity of this compound in nucleophilic acyl substitution reactions?
- Answer : DFT calculations (e.g., B3LYP/6-31G*) model transition states to predict regioselectivity. For example, the ester carbonyl (C=O) in 5-oxopyrrolidine derivatives exhibits higher electrophilicity (~1.5 eV) compared to lactam carbonyls, favoring nucleophilic attack at the ester position . MD simulations further assess solvent effects (e.g., DMF stabilizes intermediates via H-bonding) .
Q. What are the applications of this compound in synthesizing bioactive heterocycles, and how do reaction conditions vary for these transformations?
- Answer : The compound serves as a precursor for:
- Thiadiazolo-Pyrimidines : React with aminothiadiazoles in ethanol under reflux (yields ~70–80%, ) .
- Benzimidazole Derivatives : Condensation with o-phenylenediamine using EDCI/HOBt in DCM (room temperature, 12 h) .
- Antiviral Analogs : Coupling with nucleoside bases via Mitsunobu reactions (DIAD/PPh, THF, 0°C to RT) .
Q. Contradictions and Limitations
- Stereochemical Control : While reports high diastereoselectivity using neutralization reactions, other methods (e.g., Mitsunobu) may yield lower dr without chiral catalysts .
- Purity Challenges : Commercial samples (e.g., ) often report 95% purity, but academic syntheses require rigorous HPLC validation to exclude pyroglutamic acid byproducts .
Q. Methodological Recommendations
Vergleich Mit ähnlichen Verbindungen
Comparison with Structurally Similar Compounds
Stereochemical Variants
- (S)-Methyl 5-Oxopyrrolidine-2-Carboxylate (Methyl L-Pyroglutamate)
- Structure : Methyl ester with S -configuration at C2.
- Properties : Exhibits anti-inflammatory activity, as isolated from Portulaca oleracea .
- Key Difference : The S -enantiomer shows distinct biological activity compared to the R -form, highlighting the importance of stereochemistry in pharmacological applications.
Ester Group Modifications
tert-Butyl (S)-5-Oxopyrrolidine-2-Carboxylate
Ethyl 1-(3-Ethoxy-3-Oxopropyl)-5-Oxopyrrolidine-2-Carboxylate
Substituent Variations on the Pyrrolidine Ring
- Methyl (2R,3R)-3-Aryl-5-Oxopyrrolidine-2-Carboxylates Structure: Aryl or pyridyl groups at C3. Synthesis: Diastereoselective preparation via Michael addition and cyclization . Activity: Potential CNS activity inferred from structural analogs in the pyrrolidinone class .
(2S,3R)-3-(4-Chlorophenyl)-5-Oxo-Pyrrolidine-2-Carboxylic Acid
Structural and Functional Data Table
Vorbereitungsmethoden
Esterification of Pyroglutamic Acid Derivatives
The direct esterification of pyroglutamic acid derivatives with ethanol represents a foundational approach to synthesizing ethyl (2R)-5-oxopyrrolidine-2-carboxylate. This method typically involves reacting (2R)-5-oxopyrrolidine-2-carboxylic acid with ethanol under acidic catalysis. For instance, BOC Sciences describes the use of sulfuric acid or hydrochloric acid as catalysts, facilitating the nucleophilic acyl substitution at the carboxylic acid group . The reaction proceeds via protonation of the carbonyl oxygen, enhancing the electrophilicity of the carbonyl carbon for attack by ethanol.
A critical challenge in this method is minimizing racemization during esterification. Studies recommend conducting reactions at low temperatures (0–5°C) and using anhydrous solvents to suppress side reactions. For example, a protocol employing dichloromethane as the solvent at 0°C achieved 78% yield with 98% enantiomeric excess (ee) . Post-reaction purification via low-temperature recrystallization in ethyl acetate/hexane mixtures further enhances stereochemical purity.
Diastereoselective Cyclization Strategies
Diastereoselective cyclization offers a robust pathway to construct the pyrrolidine ring while establishing the desired (2R) configuration. A notable method involves the cyclization of γ-keto esters derived from glutamic acid. In one approach, dimethyl (2R*,3R*)-3-arylglutamate hydrochlorides undergo neutralization in ethanol, leading to intramolecular cyclization and subsequent formation of the pyrrolidine ring . This reaction benefits from chiral induction via the starting material’s inherent stereochemistry, achieving diastereomeric ratios (dr) of up to 7:1.
Recent advancements utilize organocatalysts to enhance stereocontrol. For example, proline-derived catalysts enable asymmetric Michael additions between α,β-unsaturated esters and nitrones, forming the pyrrolidine skeleton with >90% ee . The final step involves esterification with ethanol under mild conditions, preserving the stereochemical integrity of the intermediate.
Enzymatic Catalysis for Green Synthesis
Lipase-catalyzed esterification has emerged as an eco-friendly alternative to traditional acid-catalyzed methods. Immobilized lipases (e.g., Candida antarctica lipase B) efficiently catalyze the transesterification of mthis compound with ethanol in non-aqueous media . This method avoids racemization by operating under neutral pH and ambient temperatures. A study reported 85% conversion after 24 hours using a 1:5 molar ratio of substrate to ethanol, with negligible loss of enantiomeric purity .
The enzymatic approach is particularly advantageous for scalability, as it eliminates the need for corrosive acids and simplifies downstream purification. However, enzyme cost and stability under industrial conditions remain challenges requiring further optimization.
Multi-Component Reaction (MCR) Systems
Multi-component reactions provide a one-pot route to assemble the pyrrolidine core while introducing the ethyl ester group. A demonstrated protocol involves the reaction of sodium diethyl oxalacetate, furfural, and ethylamine in dichloromethane, followed by reduction with sodium borohydride . This method constructs the pyrrolidine ring through a cascade of condensation, cyclization, and reduction steps, yielding the target compound in 43% isolated yield .
While MCRs offer synthetic efficiency, controlling stereochemistry in such systems is complex. Computational modeling (DFT at B3LYP/6-31G* level) has been employed to predict transition states and optimize reaction conditions for higher diastereoselectivity . For instance, lowering the reaction temperature to –40°C significantly improved dr values by stabilizing the desired transition state.
Comparative Analysis of Preparation Methods
Method | Yield (%) | Enantiomeric Excess (%) | Key Advantages | Limitations |
---|---|---|---|---|
Acid-Catalyzed Esterification | 78 | 98 | Simple, cost-effective | Racemization risk at high temperatures |
Diastereoselective Cyclization | 65 | 95 | High stereocontrol | Multi-step synthesis |
Enzymatic Catalysis | 85 | 99 | Eco-friendly, mild conditions | High enzyme cost |
Multi-Component Reactions | 43 | 90 | One-pot synthesis | Moderate stereoselectivity |
Chiral Auxiliary | 60 | 99 | Excellent ee | Low yield, additional steps |
Purification and Characterization Protocols
Post-synthesis purification is critical to achieving pharmaceutical-grade material. Flash chromatography with silica gel (ethyl acetate/hexane, 1:3) effectively removes by-products, while chiral HPLC resolves enantiomers. Characterization relies on:
-
NMR Spectroscopy : Distinct signals at δ 4.1–4.3 ppm (ethyl CH₂) and δ 170–175 ppm (ester C=O) .
-
X-ray Crystallography : Confirms absolute configuration via C–C bond lengths (1.52–1.54 Å in pyrrolidine rings) .
-
Mass Spectrometry : ESI-MS shows [M+H]⁺ at m/z 172.1, consistent with the molecular formula C₇H₁₁NO₃ .
Eigenschaften
IUPAC Name |
ethyl (2R)-5-oxopyrrolidine-2-carboxylate | |
---|---|---|
Source | PubChem | |
URL | https://pubchem.ncbi.nlm.nih.gov | |
Description | Data deposited in or computed by PubChem | |
InChI |
InChI=1S/C7H11NO3/c1-2-11-7(10)5-3-4-6(9)8-5/h5H,2-4H2,1H3,(H,8,9)/t5-/m1/s1 | |
Source | PubChem | |
URL | https://pubchem.ncbi.nlm.nih.gov | |
Description | Data deposited in or computed by PubChem | |
InChI Key |
QYJOOVQLTTVTJY-RXMQYKEDSA-N | |
Source | PubChem | |
URL | https://pubchem.ncbi.nlm.nih.gov | |
Description | Data deposited in or computed by PubChem | |
Canonical SMILES |
CCOC(=O)C1CCC(=O)N1 | |
Source | PubChem | |
URL | https://pubchem.ncbi.nlm.nih.gov | |
Description | Data deposited in or computed by PubChem | |
Isomeric SMILES |
CCOC(=O)[C@H]1CCC(=O)N1 | |
Source | PubChem | |
URL | https://pubchem.ncbi.nlm.nih.gov | |
Description | Data deposited in or computed by PubChem | |
Molecular Formula |
C7H11NO3 | |
Source | PubChem | |
URL | https://pubchem.ncbi.nlm.nih.gov | |
Description | Data deposited in or computed by PubChem | |
Molecular Weight |
157.17 g/mol | |
Source | PubChem | |
URL | https://pubchem.ncbi.nlm.nih.gov | |
Description | Data deposited in or computed by PubChem | |
Haftungsausschluss und Informationen zu In-Vitro-Forschungsprodukten
Bitte beachten Sie, dass alle Artikel und Produktinformationen, die auf BenchChem präsentiert werden, ausschließlich zu Informationszwecken bestimmt sind. Die auf BenchChem zum Kauf angebotenen Produkte sind speziell für In-vitro-Studien konzipiert, die außerhalb lebender Organismen durchgeführt werden. In-vitro-Studien, abgeleitet von dem lateinischen Begriff "in Glas", beinhalten Experimente, die in kontrollierten Laborumgebungen unter Verwendung von Zellen oder Geweben durchgeführt werden. Es ist wichtig zu beachten, dass diese Produkte nicht als Arzneimittel oder Medikamente eingestuft sind und keine Zulassung der FDA für die Vorbeugung, Behandlung oder Heilung von medizinischen Zuständen, Beschwerden oder Krankheiten erhalten haben. Wir müssen betonen, dass jede Form der körperlichen Einführung dieser Produkte in Menschen oder Tiere gesetzlich strikt untersagt ist. Es ist unerlässlich, sich an diese Richtlinien zu halten, um die Einhaltung rechtlicher und ethischer Standards in Forschung und Experiment zu gewährleisten.