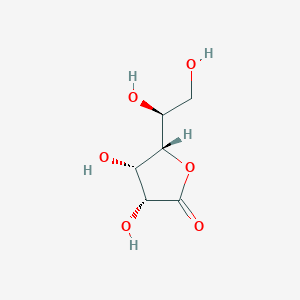
L-Mannono-1,4-lacton
Übersicht
Beschreibung
L-Mannono-1,4-lactone is a carbohydrate-based lactone with the empirical formula C6H10O6. It is a cyclic ester derived from L-mannonic acid and is known for its role in various biochemical processes. This compound is a white crystalline solid that is soluble in water and has a melting point of approximately 153-155°C .
Wissenschaftliche Forschungsanwendungen
Chemical Properties and Structure
L-Mannono-1,4-lactone has the molecular formula . Its structure is characterized by a lactone ring formed through the intramolecular esterification of hydroxyl groups. This cyclic structure contributes to its reactivity and potential applications in synthetic chemistry.
Synthetic Applications
L-Mannono-1,4-lactone serves as an important intermediate in the synthesis of various carbohydrates and derivatives. Its ability to undergo transformations makes it valuable for producing compounds such as L-ribose, which is crucial in the synthesis of nucleoside-based pharmaceuticals.
Case Study: Synthesis of L-Ribose
A notable application involves the one-pot inversion of D-mannono-1,4-lactone to produce L-ribose efficiently. This method highlights the compound's utility in generating valuable sugars for therapeutic use. The conversion process demonstrates high yields and is significant for industrial applications where L-ribose is required in large quantities .
Enzyme Interaction Studies
Research has indicated that L-mannono-1,4-lactone can interact with various enzymes, influencing metabolic pathways. Studies have focused on its role as a substrate for enzymes such as mannose-6-phosphate isomerase, which facilitates the production of L-ribose from other sugar precursors.
Enzyme Specificity
The substrate specificity of mannose-6-phosphate isomerase has been investigated, revealing that it exhibits high activity with aldoses like L-ribulose and D-mannose. This specificity underscores the potential of L-mannono-1,4-lactone in biocatalytic processes aimed at synthesizing rare sugars .
Biological Activities
L-Mannono-1,4-lactone exhibits several biological activities that may be leveraged for therapeutic applications. Its sugar-like structure allows it to participate in metabolic processes and influence cellular functions.
Potential Therapeutic Uses
Preliminary studies suggest that compounds derived from L-mannono-1,4-lactone may possess anti-diabetic properties. For instance, derivatives have been explored for their ability to inhibit enzymes related to carbohydrate metabolism, potentially aiding in the management of type 2 diabetes .
Comparative Analysis with Related Compounds
Understanding how L-mannono-1,4-lactone compares to other similar compounds can provide insights into its unique properties and applications.
Compound | Structure Type | Unique Features |
---|---|---|
D-Mannose | Aldohexose | Precursor to D-mannono-1,4-lactone; non-cyclic |
L-Ribose | Aldopentose | Product of D-mannono-1,4-lactone synthesis; five carbons |
D-Mannonic Acid | Carboxylic acid | Hydrolysis product of D-mannono-1,4-lactone |
D-Sorbitol | Sugar alcohol | Reduced form of D-mannose; different functional properties |
This table illustrates the relationships among these compounds and emphasizes the significance of L-mannono-1,4-lactone within carbohydrate chemistry.
Biochemische Analyse
Biochemical Properties
L-Mannono-1,4-lactone is involved in various biochemical reactions. For instance, it has been reported that L-Mannono-1,4-lactone acts as a substrate for certain enzymes in the biosynthesis of ascorbate .
Dosage Effects in Animal Models
The effects of L-Mannono-1,4-lactone can vary with different dosages in animal models. While specific threshold effects, as well as toxic or adverse effects at high doses, have not been fully elucidated, it is known that the compound’s effects can vary significantly depending on the dosage .
Vorbereitungsmethoden
Synthetic Routes and Reaction Conditions
L-Mannono-1,4-lactone can be synthesized through the selective anomeric oxidation of unprotected aldoses with bromine. This method typically yields the thermodynamically more stable five-membered lactone (γ-lactone) . Another method involves the dehydrogenation of unprotected or partially protected alditols and aldoses catalyzed by a transition metal complex in the presence of a hydrogen acceptor . Additionally, protected aldoses with a free anomeric hydroxyl group can be converted into the corresponding aldonolactones using chromium (VI) reagents or DMSO-based oxidizing systems .
Industrial Production Methods
Industrial production of L-Mannono-1,4-lactone often involves the aerobic oxidation of unprotected aldoses over heterogeneous catalysts, such as palladium on carbon (Pd/C), gold on carbon (Au/C), or a combination of bismuth-palladium on carbon (Bi-Pd/C) . These methods are preferred for their efficiency and scalability.
Analyse Chemischer Reaktionen
Types of Reactions
L-Mannono-1,4-lactone undergoes various chemical reactions, including oxidation, reduction, and substitution reactions.
Common Reagents and Conditions
Oxidation: Common oxidizing agents include bromine and chromium (VI) reagents.
Reduction: Sodium borohydride (NaBH4) is often used for the reduction of L-Mannono-1,4-lactone.
Substitution: Various nucleophiles can be used to substitute the lactone ring, leading to the formation of different derivatives.
Major Products Formed
The major products formed from these reactions include various aldonolactones and their derivatives, which can be further utilized in the synthesis of more complex molecules .
Wirkmechanismus
L-Mannono-1,4-lactone exerts its effects through its interaction with specific enzymes involved in carbohydrate metabolism. For instance, it is hydrolyzed by lactonases, which convert it into its corresponding acid form . This hydrolysis is stereospecific, meaning that only one enantiomer of the lactone is hydrolyzed by the enzyme, while the other enantiomer acts as a competitive inhibitor .
Vergleich Mit ähnlichen Verbindungen
L-Mannono-1,4-lactone can be compared with other similar carbohydrate-based lactones, such as:
D-Mannono-1,4-lactone: Similar in structure but differs in the stereochemistry of the hydroxyl groups.
L-Gulono-1,4-lactone: Another carbohydrate-based lactone involved in the biosynthesis of ascorbic acid.
D-Glucono-1,4-lactone: Commonly used in food and pharmaceutical industries.
L-Mannono-1,4-lactone is unique due to its specific stereochemistry and its role in the biosynthesis of ascorbic acid, making it a valuable compound in both biochemical research and industrial applications .
Biologische Aktivität
L-Mannono-1,4-lactone is a cyclic ester derived from L-mannonic acid, a sugar acid that plays a significant role in carbohydrate metabolism. This compound has garnered attention due to its potential biological activities, which may have implications in various fields, including biochemistry and pharmacology. This article explores the biological activity of L-mannono-1,4-lactone, supported by research findings and case studies.
Chemical Structure and Properties
L-Mannono-1,4-lactone has the molecular formula C₆H₈O₆ and features a lactone ring formed through the intramolecular esterification of its hydroxyl groups. Its structure is critical for its biological activity, influencing how it interacts with enzymes and other biomolecules.
1. Metabolic Pathways
L-Mannono-1,4-lactone is involved in several metabolic pathways. It can act as an intermediate in the synthesis of complex carbohydrates, which are essential for various cellular functions. The compound's sugar-like structure allows it to participate in metabolic reactions that affect energy production and cellular signaling.
2. Enzymatic Interactions
Research indicates that L-mannono-1,4-lactone can interact with various enzymes, influencing their activity. For example, studies have shown that certain dehydrogenases can utilize L-mannono-1,4-lactone as a substrate, leading to the production of other biologically active compounds. This enzymatic conversion is crucial for understanding its role in carbohydrate metabolism.
Case Study 1: Enzymatic Hydrolysis
A study conducted by BmulJ_04915 highlighted the hydrolysis of sugar lactones including L-mannono-1,4-lactone by specific enzymes from Burkholderia multivorans. The study found that these enzymes exhibited high catalytic efficiency with kcat values indicating rapid conversion rates at physiological pH levels . This underscores the potential of L-mannono-1,4-lactone in biocatalytic applications.
Case Study 2: Antimicrobial Activity
In another investigation into the antimicrobial properties of various lactones, researchers noted that L-mannono-1,4-lactone exhibited inhibitory effects against several pathogenic microorganisms. This suggests potential applications in developing natural preservatives or therapeutic agents against infections .
Comparative Analysis
To better understand L-mannono-1,4-lactone's unique properties and activities, it is beneficial to compare it with related compounds:
Compound | Structure Type | Unique Features |
---|---|---|
D-Mannose | Aldohexose | Precursor to D-mannono-1,4-lactone; non-cyclic |
D-Mannonic Acid | Carboxylic Acid | Hydrolysis product; precursor to D-mannono-1,4-lactone |
D-Sorbitol | Sugar Alcohol | Reduced form of D-mannose; different functional properties |
L-Ribose | Aldopentose | Product of D-mannono-1,4-lactone synthesis; five carbons |
This table illustrates the relationships between these compounds and highlights how L-mannono-1,4-lactone fits into the broader context of carbohydrate chemistry.
Research Findings
Recent studies have focused on elucidating the mechanisms through which L-mannono-1,4-lactone exerts its biological effects. For instance:
- Antioxidant Properties : Some research suggests that lactones can exhibit antioxidant activities by scavenging free radicals, potentially offering protective effects against oxidative stress .
- Therapeutic Potential : Investigations into its role as a precursor for bioactive compounds have opened avenues for developing new therapeutic agents targeting metabolic disorders and infections .
Eigenschaften
IUPAC Name |
(3R,4S,5S)-5-[(1S)-1,2-dihydroxyethyl]-3,4-dihydroxyoxolan-2-one | |
---|---|---|
Source | PubChem | |
URL | https://pubchem.ncbi.nlm.nih.gov | |
Description | Data deposited in or computed by PubChem | |
InChI |
InChI=1S/C6H10O6/c7-1-2(8)5-3(9)4(10)6(11)12-5/h2-5,7-10H,1H2/t2-,3-,4+,5-/m0/s1 | |
Source | PubChem | |
URL | https://pubchem.ncbi.nlm.nih.gov | |
Description | Data deposited in or computed by PubChem | |
InChI Key |
SXZYCXMUPBBULW-KLVWXMOXSA-N | |
Source | PubChem | |
URL | https://pubchem.ncbi.nlm.nih.gov | |
Description | Data deposited in or computed by PubChem | |
Canonical SMILES |
C(C(C1C(C(C(=O)O1)O)O)O)O | |
Source | PubChem | |
URL | https://pubchem.ncbi.nlm.nih.gov | |
Description | Data deposited in or computed by PubChem | |
Isomeric SMILES |
C([C@@H]([C@H]1[C@H]([C@H](C(=O)O1)O)O)O)O | |
Source | PubChem | |
URL | https://pubchem.ncbi.nlm.nih.gov | |
Description | Data deposited in or computed by PubChem | |
Molecular Formula |
C6H10O6 | |
Source | PubChem | |
URL | https://pubchem.ncbi.nlm.nih.gov | |
Description | Data deposited in or computed by PubChem | |
Molecular Weight |
178.14 g/mol | |
Source | PubChem | |
URL | https://pubchem.ncbi.nlm.nih.gov | |
Description | Data deposited in or computed by PubChem | |
Retrosynthesis Analysis
AI-Powered Synthesis Planning: Our tool employs the Template_relevance Pistachio, Template_relevance Bkms_metabolic, Template_relevance Pistachio_ringbreaker, Template_relevance Reaxys, Template_relevance Reaxys_biocatalysis model, leveraging a vast database of chemical reactions to predict feasible synthetic routes.
One-Step Synthesis Focus: Specifically designed for one-step synthesis, it provides concise and direct routes for your target compounds, streamlining the synthesis process.
Accurate Predictions: Utilizing the extensive PISTACHIO, BKMS_METABOLIC, PISTACHIO_RINGBREAKER, REAXYS, REAXYS_BIOCATALYSIS database, our tool offers high-accuracy predictions, reflecting the latest in chemical research and data.
Strategy Settings
Precursor scoring | Relevance Heuristic |
---|---|
Min. plausibility | 0.01 |
Model | Template_relevance |
Template Set | Pistachio/Bkms_metabolic/Pistachio_ringbreaker/Reaxys/Reaxys_biocatalysis |
Top-N result to add to graph | 6 |
Feasible Synthetic Routes
Q1: What is unique about the reactivity of L-Mannono-1,4-lactone compared to other aldono-1,4-lactones?
A: While the provided research doesn't directly compare the reactivity of L-Mannono-1,4-lactone to other aldono-1,4-lactones, it highlights its selective reactivity in specific reactions. For example, [] describes how L-Mannono-1,4-lactone undergoes regioselective pivaloylation, primarily yielding the 2,5,6-tri-O-pivaloyl derivative. This selectivity suggests that the hydroxyl groups at positions 2, 5, and 6 are more reactive towards pivaloyl chloride under the reaction conditions. This information could be valuable for researchers aiming to selectively modify L-Mannono-1,4-lactone for further synthetic applications. You can find more details on this in the paper "Regioselective Acylations of Aldono-1,4-lactones": .
Q2: How does the structural conformation of L-Mannono-1,4-lactone influence its reactivity?
A: [] investigates the reaction of monolithiated 1,7-dicarba-closo-dodecaborane(12) with O-isopropylidene derivatives of L-Mannono-1,4-lactone. The research indicates that the reaction product, a novel boron neutron capture candidate, preferentially adopts the β-L configuration. This suggests that the specific arrangement of atoms in the L-Mannono-1,4-lactone structure influences the stereochemistry of the final product. Understanding these structural nuances can be crucial for predicting the outcomes of reactions involving this compound. For further details, refer to the paper "1-[1,7-dicarba-closo-dodecaboran(12)-1-yl]aldoses: Novel Boron Neutron Capture Candidates": .
Q3: Are there specific analytical techniques mentioned in the research that are particularly useful for studying L-Mannono-1,4-lactone and its derivatives?
A: [] focuses on determining the absolute configuration of a derivative of L-Mannono-1,4-lactone, 2,3:5,6-Di-O-isopropylidene-3,5-di-C-methyl-l-mannono-1,4-lactone, using X-ray crystallography. This technique proved essential for unequivocally establishing the relative and absolute configuration of the molecule. This highlights the importance of X-ray crystallography in analyzing the three-dimensional structures of L-Mannono-1,4-lactone derivatives and understanding their stereochemistry. The research paper "2,3:5,6-Di-O-isopropylidene-3,5-di-C-methyl-l-mannono-1,4-lactone": provides a comprehensive look at this application.
Haftungsausschluss und Informationen zu In-Vitro-Forschungsprodukten
Bitte beachten Sie, dass alle Artikel und Produktinformationen, die auf BenchChem präsentiert werden, ausschließlich zu Informationszwecken bestimmt sind. Die auf BenchChem zum Kauf angebotenen Produkte sind speziell für In-vitro-Studien konzipiert, die außerhalb lebender Organismen durchgeführt werden. In-vitro-Studien, abgeleitet von dem lateinischen Begriff "in Glas", beinhalten Experimente, die in kontrollierten Laborumgebungen unter Verwendung von Zellen oder Geweben durchgeführt werden. Es ist wichtig zu beachten, dass diese Produkte nicht als Arzneimittel oder Medikamente eingestuft sind und keine Zulassung der FDA für die Vorbeugung, Behandlung oder Heilung von medizinischen Zuständen, Beschwerden oder Krankheiten erhalten haben. Wir müssen betonen, dass jede Form der körperlichen Einführung dieser Produkte in Menschen oder Tiere gesetzlich strikt untersagt ist. Es ist unerlässlich, sich an diese Richtlinien zu halten, um die Einhaltung rechtlicher und ethischer Standards in Forschung und Experiment zu gewährleisten.