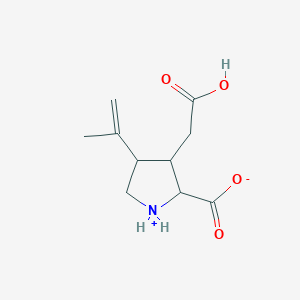
3-Pyrrolidineacetic acid, 2-carboxy-4-(1-methylethenyl)-
Übersicht
Beschreibung
3-(Carboxymethyl)-4-prop-1-en-2-ylpyrrolidin-1-ium-2-carboxylate is a natural product found in Chondria armata with data available.
Wissenschaftliche Forschungsanwendungen
Neurochemische Forschung
Kainic Acid ist ein Agonist von exzitatorischen Aminosäurerezeptoren, der selektiv für den Kainat-Rezeptorsubtyp ist . Es wird in der neurochemischen Forschung verwendet, um die Funktion und das Verhalten dieser Rezeptoren zu untersuchen .
AMPA-Rezeptor-Aktivierung
Neben der Aktivierung von Kainat-Rezeptoren aktiviert Kainic Acid auch AMPA-Rezeptoren (α-Amino-3-Hydroxy-5-Methyl-4-Isoxazolpropionsäure) . AMPA-Rezeptoren sind an der schnellen synaptischen Übertragung im zentralen Nervensystem beteiligt .
Modellierung neurodegenerativer Erkrankungen
Aufgrund seiner exzitotoxischen Eigenschaften wird Kainic Acid häufig in der Forschung verwendet, um Tiermodelle für neurodegenerative Erkrankungen wie Epilepsie und Alzheimer-Krankheit zu erstellen .
Neuropharmakologie
Kainic Acid wird in der Neuropharmakologie verwendet, um die Auswirkungen von Medikamenten auf Kainat- und AMPA-Rezeptoren zu untersuchen .
Neurotoxizitätsstudien
Kainic Acid kann verwendet werden, um Neurotoxizität zu untersuchen, da es durch Exzitotoxizität zum Absterben von Nervenzellen führen kann .
Insektizidforschung
Einige Forschungsarbeiten deuten darauf hin, dass Kainic Acid und seine Derivate als umweltfreundliche Insektizide eingesetzt werden könnten .
Wirkmechanismus
Target of Action
The primary target of 3-Pyrrolidineacetic acid, 2-carboxy-4-(1-methylethenyl)-, also known as Kainic Acid, is the kainate receptor subtype . This receptor is a type of excitatory amino acid receptor that plays a crucial role in the transmission of excitatory signals in the central nervous system .
Mode of Action
Kainic Acid acts as an agonist for the kainate receptor subtype . This means it binds to this receptor and activates it. The activation of these receptors leads to the opening of ion channels that allow the flow of sodium and potassium ions across the cell membrane, resulting in the generation of an action potential .
Biochemical Pathways
The activation of kainate receptors by Kainic Acid triggers a cascade of biochemical events. These include the depolarization of the neuron, the influx of calcium ions, and the activation of second messenger systems . The exact pathways and their downstream effects can vary depending on the specific type of neuron and its location within the nervous system.
Result of Action
The activation of kainate receptors by Kainic Acid can have various effects at the molecular and cellular levels. These include changes in neuronal excitability, synaptic plasticity, and neurotoxicity . The specific effects can depend on factors such as the dose of Kainic Acid and the type of neurons involved.
Action Environment
The action, efficacy, and stability of Kainic Acid can be influenced by various environmental factors. These include the pH and temperature of the surrounding environment, the presence of other molecules that can interact with Kainic Acid, and the specific characteristics of the target neurons .
Eigenschaften
IUPAC Name |
3-(carboxymethyl)-4-prop-1-en-2-ylpyrrolidine-2-carboxylic acid | |
---|---|---|
Source | PubChem | |
URL | https://pubchem.ncbi.nlm.nih.gov | |
Description | Data deposited in or computed by PubChem | |
InChI |
InChI=1S/C10H15NO4/c1-5(2)7-4-11-9(10(14)15)6(7)3-8(12)13/h6-7,9,11H,1,3-4H2,2H3,(H,12,13)(H,14,15) | |
Source | PubChem | |
URL | https://pubchem.ncbi.nlm.nih.gov | |
Description | Data deposited in or computed by PubChem | |
InChI Key |
VLSMHEGGTFMBBZ-UHFFFAOYSA-N | |
Source | PubChem | |
URL | https://pubchem.ncbi.nlm.nih.gov | |
Description | Data deposited in or computed by PubChem | |
Canonical SMILES |
CC(=C)C1CNC(C1CC(=O)O)C(=O)O | |
Source | PubChem | |
URL | https://pubchem.ncbi.nlm.nih.gov | |
Description | Data deposited in or computed by PubChem | |
Molecular Formula |
C10H15NO4 | |
Source | PubChem | |
URL | https://pubchem.ncbi.nlm.nih.gov | |
Description | Data deposited in or computed by PubChem | |
DSSTOX Substance ID |
DTXSID90859403 | |
Record name | 2-Carboxy-4-(1-methylethenyl)-3-pyrrolidineacetic acid | |
Source | EPA DSSTox | |
URL | https://comptox.epa.gov/dashboard/DTXSID90859403 | |
Description | DSSTox provides a high quality public chemistry resource for supporting improved predictive toxicology. | |
Molecular Weight |
213.23 g/mol | |
Source | PubChem | |
URL | https://pubchem.ncbi.nlm.nih.gov | |
Description | Data deposited in or computed by PubChem | |
CAS No. |
487-79-6, 62137-25-1 | |
Record name | kainic acid | |
Source | DTP/NCI | |
URL | https://dtp.cancer.gov/dtpstandard/servlet/dwindex?searchtype=NSC&outputformat=html&searchlist=759587 | |
Description | The NCI Development Therapeutics Program (DTP) provides services and resources to the academic and private-sector research communities worldwide to facilitate the discovery and development of new cancer therapeutic agents. | |
Explanation | Unless otherwise indicated, all text within NCI products is free of copyright and may be reused without our permission. Credit the National Cancer Institute as the source. | |
Record name | NSC152017 | |
Source | DTP/NCI | |
URL | https://dtp.cancer.gov/dtpstandard/servlet/dwindex?searchtype=NSC&outputformat=html&searchlist=152017 | |
Description | The NCI Development Therapeutics Program (DTP) provides services and resources to the academic and private-sector research communities worldwide to facilitate the discovery and development of new cancer therapeutic agents. | |
Explanation | Unless otherwise indicated, all text within NCI products is free of copyright and may be reused without our permission. Credit the National Cancer Institute as the source. | |
Record name | 2-Carboxy-4-(1-methylethenyl)-3-pyrrolidineacetic acid | |
Source | EPA DSSTox | |
URL | https://comptox.epa.gov/dashboard/DTXSID90859403 | |
Description | DSSTox provides a high quality public chemistry resource for supporting improved predictive toxicology. | |
Retrosynthesis Analysis
AI-Powered Synthesis Planning: Our tool employs the Template_relevance Pistachio, Template_relevance Bkms_metabolic, Template_relevance Pistachio_ringbreaker, Template_relevance Reaxys, Template_relevance Reaxys_biocatalysis model, leveraging a vast database of chemical reactions to predict feasible synthetic routes.
One-Step Synthesis Focus: Specifically designed for one-step synthesis, it provides concise and direct routes for your target compounds, streamlining the synthesis process.
Accurate Predictions: Utilizing the extensive PISTACHIO, BKMS_METABOLIC, PISTACHIO_RINGBREAKER, REAXYS, REAXYS_BIOCATALYSIS database, our tool offers high-accuracy predictions, reflecting the latest in chemical research and data.
Strategy Settings
Precursor scoring | Relevance Heuristic |
---|---|
Min. plausibility | 0.01 |
Model | Template_relevance |
Template Set | Pistachio/Bkms_metabolic/Pistachio_ringbreaker/Reaxys/Reaxys_biocatalysis |
Top-N result to add to graph | 6 |
Feasible Synthetic Routes
Haftungsausschluss und Informationen zu In-Vitro-Forschungsprodukten
Bitte beachten Sie, dass alle Artikel und Produktinformationen, die auf BenchChem präsentiert werden, ausschließlich zu Informationszwecken bestimmt sind. Die auf BenchChem zum Kauf angebotenen Produkte sind speziell für In-vitro-Studien konzipiert, die außerhalb lebender Organismen durchgeführt werden. In-vitro-Studien, abgeleitet von dem lateinischen Begriff "in Glas", beinhalten Experimente, die in kontrollierten Laborumgebungen unter Verwendung von Zellen oder Geweben durchgeführt werden. Es ist wichtig zu beachten, dass diese Produkte nicht als Arzneimittel oder Medikamente eingestuft sind und keine Zulassung der FDA für die Vorbeugung, Behandlung oder Heilung von medizinischen Zuständen, Beschwerden oder Krankheiten erhalten haben. Wir müssen betonen, dass jede Form der körperlichen Einführung dieser Produkte in Menschen oder Tiere gesetzlich strikt untersagt ist. Es ist unerlässlich, sich an diese Richtlinien zu halten, um die Einhaltung rechtlicher und ethischer Standards in Forschung und Experiment zu gewährleisten.