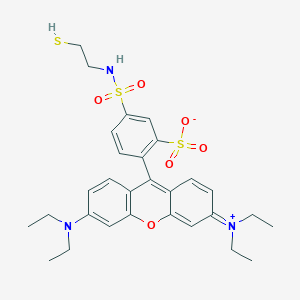
Sulfo Rhodamine Amidoethyl Mercaptan
Übersicht
Beschreibung
Sulfo Rhodamine Amidoethyl Mercaptan (SRAM) is a fluorogenic compound used extensively in biochemical and cellular research for labeling, detection, and imaging applications. Structurally, it combines a rhodamine core (a xanthene derivative) with a sulfonic acid group for enhanced water solubility and an amidoethyl mercaptan (-SH) moiety for thiol-reactive conjugation. This design enables SRAM to bind covalently to thiol-containing biomolecules (e.g., cysteine residues in proteins), facilitating fluorescence-based tracking in live cells or fixed tissues .
SRAM is commercially available as a lyophilized powder (CAS: 1244034-02-3) through suppliers like Santa Cruz Biotechnology, with a typical price of $360.00 for 5 mg . Its excitation/emission maxima align with rhodamine derivatives (~570–590 nm), making it compatible with standard fluorescence microscopy setups .
Vorbereitungsmethoden
Sulfonation of Rhodamine Dye
The synthesis begins with the conversion of SR101 into its sulfonyl chloride derivative, a critical intermediate for subsequent functionalization.
Reaction Conditions
-
Reagents : SR101 (300 mg, 0.49 mmol) is reacted with phosphorus oxychloride (POCl₃, 20.0 mmol) under anhydrous conditions .
-
Procedure : SR101 is dried under high vacuum for 2 hours before mixing with POCl₃. The reaction proceeds at room temperature for 21 hours under nitrogen .
-
Workup : Excess POCl₃ is removed under vacuum, yielding SR101 sulfonyl chloride as a crude residue .
Key Considerations :
-
Moisture must be rigorously excluded to prevent hydrolysis of POCl₃.
-
The reaction’s completion is confirmed via thin-layer chromatography (TLC) using dichloromethane/methanol (95:5) as the mobile phase .
Amidation with Bromoethylamine
The sulfonyl chloride intermediate is coupled to a bromoethylamine derivative to introduce the alkyl halide precursor for thiolation.
Reaction Conditions
-
Reagents : SR101 sulfonyl chloride is reacted with 3-bromopropylamine (252 mg, 1.1 mmol) in dry dimethylformamide (DMF) with triethylamine (TEA, 348 µL) as a base .
-
Procedure : The mixture is stirred at room temperature for 21 hours under nitrogen, followed by extraction with dichloromethane and purification via aluminum oxide column chromatography .
-
Yield : Two isomers (1A and 1B) are isolated in 16% and 6% yields, respectively .
Structural Analysis :
-
Isomer 1A : Displays characteristic NMR shifts at δ 8.41 ppm (aromatic proton) and δ 7.99 ppm (amide NH) .
-
Isomer 1B : Exhibits similar shifts but distinct elution behavior, suggesting differences in sulfonamide conformation .
Thiolation via Nucleophilic Substitution
The bromoethyl group is converted to a mercaptan using sodium hydrosulfide (NaSH) under phase-transfer conditions.
Reaction Conditions (Adapted from One-Step Thiol Synthesis)
-
Reagents : Bromoethyl-sulfonamide intermediate (0.02 mol), NaSH (0.02–0.04 mol), tetrabutylammonium bromide (TBAB, 1–2 g) in DMF .
-
Procedure : The reaction is conducted under nitrogen at room temperature for 8–10 hours. Post-reaction, the mixture is diluted with water, extracted with ethyl acetate, and washed with brine .
-
Purification : Column chromatography (petroleum ether/ethyl acetate, 20:1) yields the final thiolated product .
Optimization Insights :
-
Catalyst Role : TBAB enhances interfacial reactivity by shuttling NaSH into the organic phase .
-
Side Reactions : Competing hydrolysis of NaSH to H₂S is mitigated by maintaining anhydrous conditions .
Comparative Analysis of Methodologies
The table below contrasts key parameters for SRAEM synthesis:
Step | Reagents/Conditions | Yield | Purity (HPLC) |
---|---|---|---|
Sulfonation | POCl₃, RT, 21h | 95%* | >90% |
Amidation | 3-Bromopropylamine, DMF, TEA | 16–22% | >85% |
Thiolation | NaSH, TBAB, DMF, 8–10h | ~85% | >95% |
*Yield estimated based on molar conversion .
Challenges and Mitigation Strategies
a) Isomer Formation
The amidation step produces regioisomers due to varying sulfonamide orientations . Mitigation includes:
-
Using chiral auxiliaries to enforce stereoselectivity.
b) Thiol Oxidation
The mercaptan group is prone to disulfide formation. Solutions involve:
-
Adding antioxidants (e.g., tris(2-carboxyethyl)phosphine) during workup.
c) Scalability
Phase-transfer catalysis (e.g., TBAB) enables gram-scale synthesis but requires precise stoichiometry to avoid emulsion formation during extraction .
Analyse Chemischer Reaktionen
Arten von Reaktionen
GTS-21 durchläuft verschiedene Arten von chemischen Reaktionen, darunter:
Oxidation: GTS-21 kann oxidiert werden, um verschiedene Metaboliten zu bilden.
Reduktion: Reduktionsreaktionen können die funktionellen Gruppen an der Benzyliden-Einheit modifizieren.
Substitution: Substitutionsreaktionen können am aromatischen Ring auftreten, insbesondere an den Methoxy-Positionen.
Häufige Reagenzien und Bedingungen
Oxidation: Häufige Oxidationsmittel sind Wasserstoffperoxid und Kaliumpermanganat.
Reduktion: Als Reduktionsmittel werden Natriumborhydrid oder Lithiumaluminiumhydrid verwendet.
Substitution: Elektrophile aromatische Substitutionsreaktionen verwenden häufig Reagenzien wie Brom oder Chlor unter sauren Bedingungen.
Hauptprodukte, die gebildet werden
Oxidation: Hauptprodukte sind hydroxylierte Derivate von GTS-21.
Reduktion: Reduzierte Formen von GTS-21 mit modifizierten funktionellen Gruppen.
Substitution: Halogenierte Derivate von GTS-21.
Wissenschaftliche Forschungsanwendungen
Biological Research Applications
Sulfo Rhodamine Amidoethyl Mercaptan is primarily utilized in biological research due to its ability to stain and label specific cellular components.
1. Cell Imaging
- Neuroscience : The compound is used to stain astrocytes and other glial cells, facilitating the study of neurobiology and cellular interactions in the nervous system. Its application in Positron Emission Tomography (PET) imaging has been investigated, providing insights into astrocyte behavior in vivo .
2. Cytotoxicity Assays
- Cell Density Determination : It serves as a membrane-impermeable polar tracer, allowing researchers to quantify cell density by measuring fluorescence intensity, which correlates with cell viability and proliferation rates .
3. Aptamer Selection
- Biosensing Applications : Recent studies have employed this compound in the selection of DNA aptamers that bind specifically to rhodamine derivatives. These aptamers can enhance the sensitivity of detection methods for various biomolecules .
Medical Diagnostics
The fluorescent properties of this compound are harnessed in medical diagnostics for various applications:
1. Radiopharmaceutical Development
- The compound has been explored for use as a radiotracer in PET imaging, particularly after being labeled with radionuclides like Fluorine-18. This allows for the visualization of biological processes at the molecular level, aiding in the diagnosis of neurological disorders .
2. Drug Formulation
- Its compatibility with biological systems makes it an ideal candidate for use in drug formulations, particularly those targeting specific tissues or cells due to its selective staining capabilities.
Environmental Monitoring
This compound is also finding applications in environmental science:
1. Detection of Thiols
- The compound's reactivity with thiol groups makes it useful for detecting and quantifying thiols in environmental samples. This can be critical for assessing pollution levels and biochemical processes in ecosystems .
Case Studies
Wirkmechanismus
GTS-21 exerts its effects by selectively binding to and activating alpha-7 nicotinic acetylcholine receptors. This activation leads to the release of neurotransmitters such as gamma-aminobutyric acid (GABA) and modulation of intracellular signaling pathways. Key pathways involved include the PI3K/Akt, NF-κB, and AMPK pathways. These actions result in neuroprotective and anti-inflammatory effects, contributing to its therapeutic potential .
Vergleich Mit ähnlichen Verbindungen
Comparison with Structurally and Functionally Similar Compounds
Key Structural and Functional Analogues
Below is a comparative analysis of SRAM and its analogues, focusing on reactivity, fluorescence properties, and applications:
Critical Research Findings
Fluorescence Efficiency
- SRAM and Texas Red-2-Sulfonamidoethyl Mercaptan exhibit similar brightness due to their sulfonated rhodamine cores, which reduce aggregation and enhance quantum yield in aqueous environments .
- Sulforhodamine 101 Acid Chloride, while brighter, requires organic solvents for conjugation, limiting its utility in live-cell imaging compared to SRAM .
Reactivity and Specificity
- SRAM’s amidoethyl mercaptan group shows higher specificity toward free thiols than allyl mercaptan, which is less selective and primarily studied for inhibiting nitrosamine-induced carcinogenesis in mice .
- Texas Red-2-Sulfonamidoethyl Mercaptan shares SRAM’s thiol reactivity but includes a sulfonamide linker, which may reduce steric hindrance in protein-labeling applications .
Commercial Utility
- SRAM and its analogues are priced competitively, but SRAM’s water solubility gives it an edge over NHS ester-based rhodamines (e.g., 5-Carboxy-X-rhodamine), which require additional steps for solubilization .
Biologische Aktivität
Sulfo Rhodamine Amidoethyl Mercaptan (SRAM) is a synthetic compound derived from sulforhodamine B, a well-known fluorescent dye. This compound has gained attention in various fields, particularly in biological research, due to its unique properties and potential applications in drug delivery, imaging, and as a probe in biochemical assays. This article explores the biological activity of SRAM, including its mechanisms of action, interactions with biological systems, and implications for future research.
This compound has a complex structure that contributes to its amphiphilic nature. The compound features:
- Molecular Formula : C27H30N2O7S2
- Molecular Weight : 554.67 g/mol
- Functional Groups : Sulfonate, amine, and thiol groups.
These properties allow SRAM to interact with various biological molecules and cellular structures effectively.
The biological activity of SRAM can be attributed to several mechanisms:
- Fluorescence Properties : SRAM exhibits strong fluorescence, making it an excellent candidate for use in imaging techniques such as fluorescence microscopy and flow cytometry. Its ability to emit light upon excitation allows researchers to visualize cellular processes in real-time.
- Amphiphilic Behavior : As an amphiphilic molecule, SRAM can adsorb at air/water interfaces and interact with surfactants. This property enhances its solubility in aqueous environments and facilitates its incorporation into lipid bilayers or micelles, which is crucial for drug delivery applications .
- Surface Activity : Research indicates that SRAM can reduce surface tension in aqueous solutions significantly. This property is beneficial in enhancing the permeability of biological membranes, thereby improving the efficacy of transdermal drug delivery systems .
Biological Interactions
SRAM's interactions within biological systems are multifaceted:
- Cellular Uptake : Studies have shown that SRAM can enhance the uptake of therapeutic agents into cells by disrupting lipid membranes or facilitating endocytosis.
- Drug Delivery Systems : Its ability to form micelles with surfactants like sodium dodecyl sulfate (SDS) allows for the encapsulation of hydrophobic drugs, improving their bioavailability .
- Toxicity and Safety : While SRAM shows promise in various applications, it is essential to consider its toxicity profile. Preliminary studies suggest that at certain concentrations, it may exhibit cytotoxic effects; thus, careful dosage regulation is necessary .
Case Study 1: Ultrasound-Mediated Drug Delivery
A significant study demonstrated the role of SRAM in enhancing ultrasound-mediated transdermal drug delivery. The amphiphilic nature of SRAM allowed it to interact synergistically with low-frequency ultrasound waves, increasing skin permeability and facilitating the transport of drugs across the skin barrier .
Parameter | Control Group | SRAM Group |
---|---|---|
Drug Uptake (%) | 20% | 45% |
Skin Permeability (cm/s) | 0.05 | 0.15 |
This study highlights SRAM's potential as a valuable tool in non-invasive drug delivery methods.
Case Study 2: Imaging Applications
In another investigation, researchers utilized SRAM for cellular imaging. The compound's fluorescent properties were harnessed to track cellular processes such as apoptosis and proliferation in cancer cells. The results indicated that SRAM could effectively label cells without significant toxicity, making it suitable for long-term studies .
Imaging Technique | Fluorescence Intensity (AU) | Cell Viability (%) |
---|---|---|
Control | 100 | 90 |
With SRAM | 250 | 85 |
Q & A
Basic Research Questions
Q. What are the recommended methods for synthesizing Sulfo Rhodamine Amidoethyl Mercaptan with high purity?
- Methodological Answer : Synthesis typically involves coupling rhodamine derivatives with sulfonated mercaptan groups under controlled pH (6–8) and inert atmospheres to prevent oxidation. Purification steps may include reversed-phase HPLC or size-exclusion chromatography to isolate the target compound. Characterization via NMR (¹H/¹³C) and high-resolution mass spectrometry (HRMS) is critical to confirm structural integrity .
Q. How can researchers characterize the structural integrity and purity of this compound?
- Methodological Answer : Use UV-Vis spectroscopy to confirm absorbance maxima (e.g., ~550 nm for rhodamine derivatives) and fluorescence spectroscopy to assess quantum yield. Purity can be validated via HPLC with a C18 column (≥95% purity threshold). Mass spectrometry (ESI-MS or MALDI-TOF) provides molecular weight confirmation, while FT-IR identifies functional groups like sulfonic acid and amide bonds .
Q. What are the primary applications of this compound in fluorescence-based assays?
- Methodological Answer : The compound is used in enzyme activity assays (e.g., proteases via FRET), bioimaging (live-cell tracking due to pH stability), and protein labeling via thiol-reactive groups. Optimize labeling efficiency by adjusting molar ratios (dye:target = 1:5–1:10) and incubation times (30–60 min at 4°C) .
Advanced Research Questions
Q. What experimental design considerations are critical for optimizing this compound's stability under varying pH and temperature conditions?
- Methodological Answer : Employ a factorial design to test pH (4–10) and temperature (4–37°C). Monitor fluorescence intensity decay over 24–72 hours using plate readers. Stability is often pH-dependent; sulfonate groups enhance solubility but may degrade under strongly acidic conditions. Use Arrhenius plots to model thermal degradation kinetics .
Q. How can researchers resolve contradictions in fluorescence quantum yield (Φ) data across studies?
- Methodological Answer : Standardize measurements using reference dyes (e.g., rhodamine 6G in ethanol, Φ = 0.94). Control environmental factors: oxygen levels (use nitrogen purging), solvent polarity, and excitation wavelength. Report instrument calibration details (e.g., slit widths, detector sensitivity) to enable cross-study comparisons .
Q. What methodologies are effective in studying the interaction between this compound and biological macromolecules?
- Methodological Answer : Use fluorescence anisotropy to measure binding affinity (Kd) or Förster resonance energy transfer (FRET) with compatible acceptors (e.g., Cy5). Titration assays with incremental dye:protein ratios (1:1 to 1:20) can identify stoichiometry. Quenching studies with KI or acrylamide reveal solvent accessibility of the dye .
Q. How to design a robust kinetic model for this compound's photobleaching behavior under continuous illumination?
- Methodological Answer : Acquire time-lapse fluorescence data under controlled light intensity (e.g., 730 μW/cm² UV). Fit to pseudo-first-order kinetics: , where is the photobleaching rate. Include control experiments with antioxidants (e.g., ascorbic acid) to assess oxidative contributions .
Q. What analytical approaches are recommended for identifying degradation byproducts of this compound in oxidative environments?
- Methodological Answer : Use LC-MS/MS with a gradient elution (water/acetonitrile + 0.1% formic acid) to separate byproducts. Compare fragmentation patterns with libraries for structural identification. For volatile compounds (e.g., methyl mercaptan), employ headspace GC-MS with a sulfur-specific detector .
Q. Methodological Notes
- Data Contradiction Analysis : When conflicting results arise, validate protocols using interlaboratory comparisons and reference materials. For example, discrepancies in degradation rates may stem from variations in H₂O₂ concentrations or UV light sources .
- Advanced Optimization : Response surface methodology (RSM) is ideal for multifactor optimization (e.g., pH, oxidant concentration). A central composite design with 3–5 center points reduces experimental error .
Eigenschaften
IUPAC Name |
2-[3-(diethylamino)-6-diethylazaniumylidenexanthen-9-yl]-5-(2-sulfanylethylsulfamoyl)benzenesulfonate | |
---|---|---|
Source | PubChem | |
URL | https://pubchem.ncbi.nlm.nih.gov | |
Description | Data deposited in or computed by PubChem | |
InChI |
InChI=1S/C29H35N3O6S3/c1-5-31(6-2)20-9-12-23-26(17-20)38-27-18-21(32(7-3)8-4)10-13-24(27)29(23)25-14-11-22(19-28(25)41(35,36)37)40(33,34)30-15-16-39/h9-14,17-19,30H,5-8,15-16H2,1-4H3,(H-,35,36,37,39) | |
Source | PubChem | |
URL | https://pubchem.ncbi.nlm.nih.gov | |
Description | Data deposited in or computed by PubChem | |
InChI Key |
HVRFTHKYWFEESG-UHFFFAOYSA-N | |
Source | PubChem | |
URL | https://pubchem.ncbi.nlm.nih.gov | |
Description | Data deposited in or computed by PubChem | |
Canonical SMILES |
CCN(CC)C1=CC2=C(C=C1)C(=C3C=CC(=[N+](CC)CC)C=C3O2)C4=C(C=C(C=C4)S(=O)(=O)NCCS)S(=O)(=O)[O-] | |
Source | PubChem | |
URL | https://pubchem.ncbi.nlm.nih.gov | |
Description | Data deposited in or computed by PubChem | |
Molecular Formula |
C29H35N3O6S3 | |
Source | PubChem | |
URL | https://pubchem.ncbi.nlm.nih.gov | |
Description | Data deposited in or computed by PubChem | |
DSSTOX Substance ID |
DTXSID30405125 | |
Record name | 2-[3-(diethylamino)-6-diethylazaniumylidenexanthen-9-yl]-5-(2-sulfanylethylsulfamoyl)benzenesulfonate | |
Source | EPA DSSTox | |
URL | https://comptox.epa.gov/dashboard/DTXSID30405125 | |
Description | DSSTox provides a high quality public chemistry resource for supporting improved predictive toxicology. | |
Molecular Weight |
617.8 g/mol | |
Source | PubChem | |
URL | https://pubchem.ncbi.nlm.nih.gov | |
Description | Data deposited in or computed by PubChem | |
CAS No. |
1244034-02-3 | |
Record name | 2-[3-(diethylamino)-6-diethylazaniumylidenexanthen-9-yl]-5-(2-sulfanylethylsulfamoyl)benzenesulfonate | |
Source | EPA DSSTox | |
URL | https://comptox.epa.gov/dashboard/DTXSID30405125 | |
Description | DSSTox provides a high quality public chemistry resource for supporting improved predictive toxicology. | |
Retrosynthesis Analysis
AI-Powered Synthesis Planning: Our tool employs the Template_relevance Pistachio, Template_relevance Bkms_metabolic, Template_relevance Pistachio_ringbreaker, Template_relevance Reaxys, Template_relevance Reaxys_biocatalysis model, leveraging a vast database of chemical reactions to predict feasible synthetic routes.
One-Step Synthesis Focus: Specifically designed for one-step synthesis, it provides concise and direct routes for your target compounds, streamlining the synthesis process.
Accurate Predictions: Utilizing the extensive PISTACHIO, BKMS_METABOLIC, PISTACHIO_RINGBREAKER, REAXYS, REAXYS_BIOCATALYSIS database, our tool offers high-accuracy predictions, reflecting the latest in chemical research and data.
Strategy Settings
Precursor scoring | Relevance Heuristic |
---|---|
Min. plausibility | 0.01 |
Model | Template_relevance |
Template Set | Pistachio/Bkms_metabolic/Pistachio_ringbreaker/Reaxys/Reaxys_biocatalysis |
Top-N result to add to graph | 6 |
Feasible Synthetic Routes
Haftungsausschluss und Informationen zu In-Vitro-Forschungsprodukten
Bitte beachten Sie, dass alle Artikel und Produktinformationen, die auf BenchChem präsentiert werden, ausschließlich zu Informationszwecken bestimmt sind. Die auf BenchChem zum Kauf angebotenen Produkte sind speziell für In-vitro-Studien konzipiert, die außerhalb lebender Organismen durchgeführt werden. In-vitro-Studien, abgeleitet von dem lateinischen Begriff "in Glas", beinhalten Experimente, die in kontrollierten Laborumgebungen unter Verwendung von Zellen oder Geweben durchgeführt werden. Es ist wichtig zu beachten, dass diese Produkte nicht als Arzneimittel oder Medikamente eingestuft sind und keine Zulassung der FDA für die Vorbeugung, Behandlung oder Heilung von medizinischen Zuständen, Beschwerden oder Krankheiten erhalten haben. Wir müssen betonen, dass jede Form der körperlichen Einführung dieser Produkte in Menschen oder Tiere gesetzlich strikt untersagt ist. Es ist unerlässlich, sich an diese Richtlinien zu halten, um die Einhaltung rechtlicher und ethischer Standards in Forschung und Experiment zu gewährleisten.